Introduction
The World Health Organization defines probiotics as “living microorganisms which, when administered in adequate amounts, confer a health benefit on the host” (Aljohani et al., 2024). Probiotics promote human health by maintaining the intestinal microbiota balance, enhancing immune function, and preventing conditions such as diarrhea, inflammatory bowel disease, and hypercholesterolemia (Hill et al., 2014; Sanders et al., 2019). These benefits have driven the global popularity of probiotics, making them essential health supplements and functional foods (Kechagia et al., 2013).
Recently, the Southeast Asian probiotic market has grown owing to increasing awareness of immunity enhancement and gut health benefits (China Research & Intelligence, 2023). However, the applications of heat-killed lactic acid bacteria (HK-LAB) are limited. HK-LAB inactivated via heat treatment retain health-promoting properties and offer advantages, such as stability and safety, for functional food applications (Piqué et al., 2019; Siciliano et al., 2021). Localized LAB strains, which reflect the unique gut microbiota of Southeast Asia, can improve product efficacy and market acceptance (Lee et al., 2017b).
Unlike live probiotics, HK-LAB do not proliferate in the gut, thus minimizing microbiota disruption (Sugahara et al., 2017). Heat treatment enhances functional properties by releasing intracellular antioxidants that reduce oxidative stress (Liu and Pan, 2010). HK-LAB also suppress pro-inflammatory cytokines [tumor necrosis factor (TNF)-α, interleukin (IL)-1β, IL-6] and increase anti-inflammatory cytokines (IL-10), supporting immune modulation (Choi et al., 2018). Lipoteichoic acid (LTA), a major cell wall component of Gram-positive bacteria, interacts with Toll-like receptor-2 (TLR-2) to modulate inflammatory responses and promote immune balance (Kang et al., 2016).
Despite these benefits, research on HK-LAB, particularly on LTA content under varying heat treatment conditions, remains limited. In this study, we isolated LAB strains from Vietnamese feces to produce HK-LAB, and compared their functional properties with those of live cells. Through LTA analysis and cellular assays, we evaluated their potential as immunomodulatory ingredients for functional foods targeted in Southeast Asian markets.
Materials and Methods
The selected strains were screened based on colony shape, Gram staining, and microscopic morphology. The selected strains were confirmed by 16S-rRNA gene sequencing based on sequence homology against 16S-rRNA gene sequences in the GenBank database (Macrogen, Seoul, Korea).
Tolerance to artificial gastric juice and bile was measured using the method described by Vinderola and Reinheimer (2003). To investigate the survival of LAB strains under acidic condition, each strain of LAB was harvested by centrifugation at 6,000×g for 10 min, washed with PBS (pH 7.4), and inoculated (1%) into MRS broth acidified to pH adjusted 3.0, 2.0 and 1.5 (using HCl) containing 1,000 units of pepsin (Sigma-Aldrich, St. Louis, MO, USA) and incubated at 37°C for 2 h. Strains inoculated in non-acidified MRS broth were used as controls. Artificial bile acid tolerance was determined by cultivating the LABs in artificial bile. LAB cultivated in artificial gastric juice were harvested by centrifugation at 6,000×g for 10 min and washed with PBS (pH 7.4). The LABs were incubated at 37°C for 24 h in MRS broth containing artificial bile (0.3% Oxgall; Becton Dickinson, Franklin Lakes, NJ, USA). The number of viable cells was determined by plating 1 mL aliquots of 10-fold serial dilutions in 0.85% saline on BCP agar. Plates were incubated at 37°C for 48 h, and CFUs were estimated.
Proteolytic activity was measured using a previously described method (Riffel and Brandelli, 2006) with some modifications. Selected LAB strains were screened for proteolytic activity using an agar well diffusion test on skim milk containing 2.0% (w/v) agar (Becton Dickinson). After centrifugation at 5,000×g for 10 min, a 100 μL aliquot of the supernatant was loaded into 9 mm-diameter wells on skim milk agar plates. Proteolysis resulted in the formation of clear zones around the wells. Protease activity was determined by measuring the diameter of the clear zone area every 24 h over 72 h of incubation at 37°C.
The β-galactosidase activity was measured as previously described (Jalili et al., 2009), with slight modifications. Cultures were grown in MRS broth at 37°C for 18 h, and OD600 was recorded. A 1 mL culture aliquot was centrifuged at 12,000×g for 10 min at 4°C, washed twice with PBS (pH 7.4), and resuspended in 300 μL of 0.25 M Tris buffer (pH 8.0). Cell lysis was performed via freeze-thaw cycles (–80°C for 30 min, room temperature for 30 min). After centrifugation (12,000×g, 3 min, 4°C), 30 μL of distilled water, 70 μL of 4 mg/mL o-nitrophenyl-β-D-galactopyranoside (Sigma-Aldrich), and 200 μL of Z buffer with β-mercaptoethanol were added to 100 μL of supernatant. The reaction was incubated at 37°C for 30 min in the dark and stopped with 500 μL of 1 M sodium carbonate. Absorbance was measured at OD420 and OD550, and β-galactosidase activity was calculated using the formula:
Where t, v, OD420, OD550, and OD600 denote reaction time (min), sample volume (mL), and absorbance (420, 550, and 600 nm), respectively.
LAB was subcultured three times at 16–18 h intervals in MRS broth, and the grown LAB was centrifuged at 10,000×g for 10 min at 4°C. After removing the supernatant, PBS (pH 7.4) was added to the cells. This process was repeated twice to harvest at 109 CFU/mL. For the heat shock treatment, cells were treated at different temperatures and times, and the viable cell count was measured.
The heat-treated dead cells were centrifuged at 18,510×g for 10 min at 4°C; then only the supernatant was taken and used as a sample. Protein was quantified using a BCA protein assay kit (Thermo Fisher Scientific, Waltham, MA, USA) and colorimetric methods (Klotz et al., 2017). Ten microliter of samples were added to a 96-well cell culture plate, and then 190 μL of reagent was added to each well. After incubation at room temperature for 30 min, absorbance was measured at 562 nm using a microplate reader.
The heat-treated dead cells were centrifuged at 18,510×g for 10 min at 4°C; then only the supernatant was taken and used as a sample. Phosphate analysis was performed using a phosphate assay kit (Sigma-Aldrich) and colorimetric methods. Fifty microliter of samples were added to a 96-well cell culture plate, and then 100 μL of reagent was added to each well. After incubation at room temperature for 30 min, the absorbance of the solution was measured at 620 nm using a microplate reader.
2,2'-Azino-bis(3-ethylbenzothiazoline-6-sulfonic acid) (ABTS) powder (7 mM, Sigma-Aldrich) in ethanol was prepared to investigate ABTS radical scavenging activity. Then, ABTS and 2.45 mM potassium persulfate (Sigma-Aldrich) were mixed in a 1:1 (v/v) ratio to prepare an ABTS solution. The resulting ABTS solution was stored at room temperature for 16 h. The ABTS•+ stock solution was diluted with ethanol to an absorbance of 0.7±0.02 at 734 nm. Nine hundred microliter of the diluted ABTS•+ solution was added to 100 μL of live and dead cell samples and reacted at 37°C for 10 min. Absorbance was measured at 734 nm after centrifugation at 12,000×g for 10 min and was calculated as follows (Hussen and Endalew, 2023).
The 2,2-diphenyl-1-picrylhydrazyl (DPPH) free radical scavenging activity was measured using a previously reported method (Han et al., 2020) with slight modifications. First, 0.4 mM DPPH was prepared in ethanol. Subsequently, 1.25 mL of DPPH solution was mixed with 500 μL of live and dead cell samples and reacted in the dark at room temperature for 30 min. Absorbance was measured at 517 nm after centrifugation at 12,000×g for 10 min, and was calculated as follows:
Ferric-reducing antioxidant power (FRAP) was measured using a previously reported method (Aregbe et al., 2024). To prepare the FRAP solution, an acetate buffer (300 mM, pH 3.6) was prepared by mixing sodium acetate trihydrate (Sigma-Aldrich) and acetic acid (J.T. Baker, Phillipsburg, NJ, USA). Then, a 10 mM TPTZ was prepared by dissolving 2,4,6‐tri (2‐pyridyl)‐1,3,5‐triazine (Sigma-Aldrich) in 40 mM HCl (DAEJUNG, Siheung, Korea). Finally, a 20 mM Iron(III) chloride hexahydrate (Sigma-Aldrich) solution was prepared by dissolving Iron(III) chloride hexahydrate (97% A.C.S reagent; Sigma-Aldrich) solution was prepared in ethanol (Samchun Chemical, Seoul, Korea). The three solutions were mixed in a ratio of 10:1:1 (v/v). For the assay, 1 mL of the FRAP solution was mixed with 30 μL of live and dead cell samples or standard solution. The mixture was vortexed and reacted at 37°C for 10 min in the dark. The absorbance was measured at 593 nm after centrifugation at 12,000×g for 10 min. The antioxidant capacity was expressed as Trolox (Sigma-Aldrich) equivalents (mM).
LTA extraction and high-performance liquid chromatography (HPLC) analyses were performed as previously described (Lu et al., 2022; Tadmor et al., 2014). Five liter of dead cells were centrifuged at 10,000×g for 10 min at 4°C and suspended with 50 mL of 0.1 M sodium citrate buffer (pH 4.7; Sigma-Aldrich). The samples were sonicated at 400 W for 30 min in an ice-cold bath. The suspension was then mixed with 50 mL of n-butyl alcohol and shaken at room temperature for 30 min. After centrifugation, the supernatant from the lower aqueous phase was collected and separated into two phases. The samples were then lyophilized. The lyophilized LTA sample was mixed with 5 mL of 10% 1-propanol in 0.1 M ammonium acetate (pH 4.7; Sigma-Aldrich) and centrifuged at 17,000×g for 60 min. The supernatant was subjected to reverse high-performance liquid chromatography (RP-HPLC; Waters, Milford, CT, USA) on a C18 analytical column (YMC Hydrosphere C18 column, 4.6 mm×250 mm, particle size 5 um; YMC, Kyoto, Japan) using 10% 1-propanol in 0.1 M ammonium acetate (pH 4.7; Table 1).
RAW 264.7 cell (KCTC, Seoul, Korea) are mouse macrophage cells. RAW 264.7 cells were cultured in Dulbecco’s modified Eagle’s medium, supplemented with heat-inactivated 10% FBS and 1% penicillin at 37°C, in a 5% CO2 atmosphere. The cells were subcultured and plated at 80%–90% of confluency.
Cell viability was determined using the 3-(4,5-dimethylthiazol-2-yl)-2,5-diphenyltetrazolium bromide (MTT) assay as previously described (Khanna et al., 2020), with minor modifications. RAW 264.7 cells were seeded at 5×104 cells/well in 96-well cell culture plates for 24 h. After removing the medium, RAW 264.7 cells were treated with various concentrations of live and dead cells in serum-free medium for 24 h. After that, 10 μL of MTT (Sigma-Aldrich) solution was added to each well. Incubation was continued for 2 h of incubation (37°C, 5% CO2). After removing the supernatant, 150 μL of dimethyl sulfoxide was added to each well. The cell culture plate was shaken for 30 min to dissolve the formazan crystals formed by the reaction between the MTT reagent and the metabolites of viable cells. Absorbance was measured at a wavelength of 540 nm using a microplate reader, and cell viability was expressed as a percentage of the untreated control cells.
Nitric oxide (NO) production was measured as nitrite using the Griess reaction (Sun et al., 2003). RAW 264.7 cells were seeded at 1×106 cells/well in 24-well cell culture plates and incubated for 24 h. After removing the medium, RAW 264.7 cells were treated with various concentrations of live and dead cells in serum-free medium for 2 h. After that, lipopolysaccharide (LPS; 1 μg/mL) was added to each well and incubated for 24 h. Following the incubation, 100 μL of the reaction mixture was extracted and added to a 96-well microplate followed by the addition of Griess reagent. The mixture was shaken in the dark for 20–30 min at room temperature and the absorbance was measured at 540 nm. A fresh culture medium was used as a blank control.
RAW 264.7 cells were seeded at 1×106 cells/well in 24-well cell culture plates and incubated for 24 h. After removing the medium, RAW 264.7 cells were treated with various concentrations of live and dead cells in a serum-free medium for 2 h. Subsequently, LPS was added at a final concentration of 1 μg/mL, and the cells were incubated for 24 h. Then, the supernatant was collected and cytokine levels were measured using Mouse TNF-α, IL-1β, and Mouse IL-6 enzyme-linked immunosorbent assay (ELISA) kits (Thermo Fisher Scientific) according to the manufacturer’s instructions. The production levels of TNF-α, IL-1β, and IL-6 were determined using ELISA (Wang et al., 2020).
Sodium dodecyl sulfate–polyacrylamide gel electrophoresis (SDS-PAGE) was performed according to the Laemmli method (Laemmli, 1970). Dead cell degradation was assessed by SDS-PAGE, as previously described method (Eslami et al., 2013) with minor modifications, using digestive enzymes such as amylase, pepsin, and oxgall. The molecular weights of dead cells were estimated using 12% acrylamide gels and a mini-protein tetra electrophoresis system (Bio-Rad, Hercules, CA, USA). The protein bands were stained with Coomassie Brilliant Blue R-250 (Bio-Rad) using Precision Plus Protein Dual Xtra Standards (Bio-Rad) as molar mass standards. Ten percentage of α-Amylase (Novozymes, Bagsvaerd, Denmark) was added to dead cell sample and reacted at 37°C for 5, 15, and 30 min. After centrifugation at 10,000×g for 10 min and 4°C, the decomposition efficiency was measured through SDS-PAGE using supernatant. The remaining pellet was added with the same amount of PBS buffer as the supernatant, adjusted to pH 3.0 using HCl, and then added pepsin (1,000 unit/mL; Sigma-Aldrich) and reacted at 37°C for 30, 60, 120 min. Centrifugation was performed as described above and SDS-PAGE was performed. After mixing the same amount of PBS buffer as the supernatant in the remaining pellet, 0.3% oxgall (BD Difco) was added and reacted at 37°C for 6, 12, 24 h, followed by centrifugation as described above, and SDS-PAGE was measured with the supernatant.
Results
Gram staining was performed to determine the morphological characteristics of each strain, and all isolates were confirmed to be Gram-positive. Six strains were identified using 16S rRNA sequencing. Based on the 16S rRNA sequence analysis, the isolates were classified as one strain of Ligilactobacillus salivarius, four strains of Lactiplantibacillus plantarum, and one strain of Limosilactobacillus fermentum. The strains were named L.salivarius V4, L. plantarum V7, V10, V11, and V12, and L. fermentum V8 (Table 2).
Survival of LAB strains isolated from Vietnamese feces was evaluated using artificial gastric fluid and bile salts (Table 3). The results demonstrated that the LAB strains exhibited excellent resistance to artificial gastric juice, with varying survival rates among the strains. Among these strains, L. plantarum V10 showed the highest survival rate. These findings are consistent with those reported by Mechai et al. (2014), who observed that the resistance of probiotic strains is strain-dependent.
Resistance to intestinal bile salts is a critical factor for probiotic selection. As the concentration of human bile typically ranges from 0.3% to 0.5%, an artificial bile acid environment was simulated using 0.3% OxGall (Hu et al., 2018). Resistance to artificial bile acids was higher in the LAB strains than in the commercial strain L. acidophilus LA-5. Notably, L. plantarum V10 exhibited superior resistance to bile acids (Table 4).
Bacterial proteolytic activity was assessed based on the ability to produce clear zones on skimmed milk agar. A clear zone was observed around the wells for these six strains, indicating proteolytic activity. Among the six strains tested, L. salivarius V4 and L. plantarum V10 exhibited the highest proteolytic activities on skim milk agar (Table 5).
β-Galactosidase plays an important role in the hydrolysis of lactose (Brás et al., 2010). This enzyme is widely used to improve lactose digestion and to convert lactose into short-chain fatty acids that are beneficial to the host (Naidu et al., 1999). L. salivarius V4, L. fermentum V8, and L. plantarum V10 showed higher β-galactosidase activity than the commercial strain L. acidophilus LA-5 (Fig. 1).
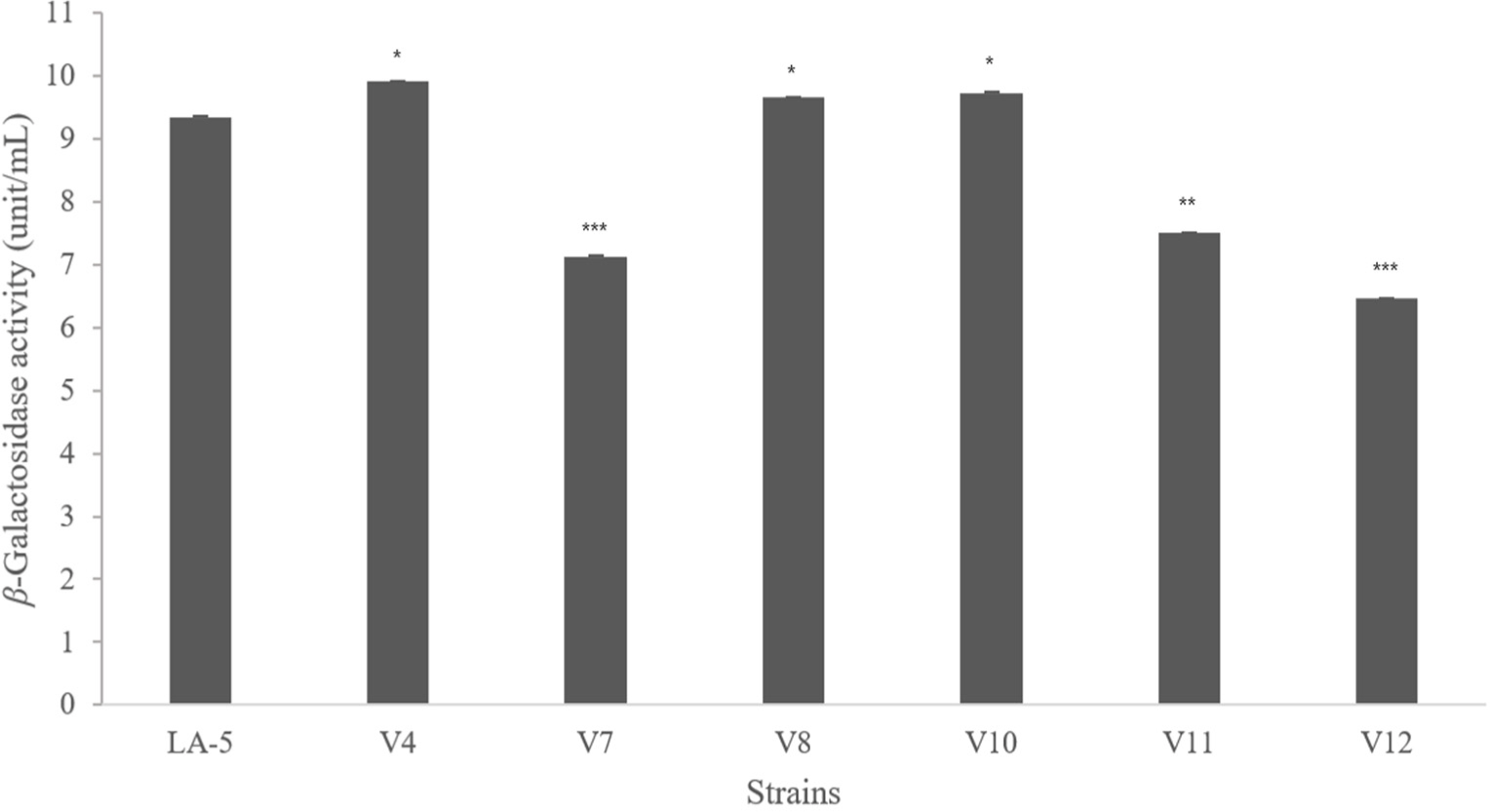
To prepare dead cell, four types of lactic acid bacteria isolated from Vietnamese feces were subjected to heat treatment at 65°C for 5, 15, 30, and 60 min and at 95°C for 5 and 10 min, after which viable cell counts were performed. As a result, all bacterial cells were completely killed after 60 min at 65°C and 10 min at 95°C (Fig. 2). Therefore, in subsequent experiments, dead cells were prepared by heat treatment at 65°C for 60 and 90 min and at 95°C for 10 and 20 min, ensuring complete inactivation and the experiments were conducted.
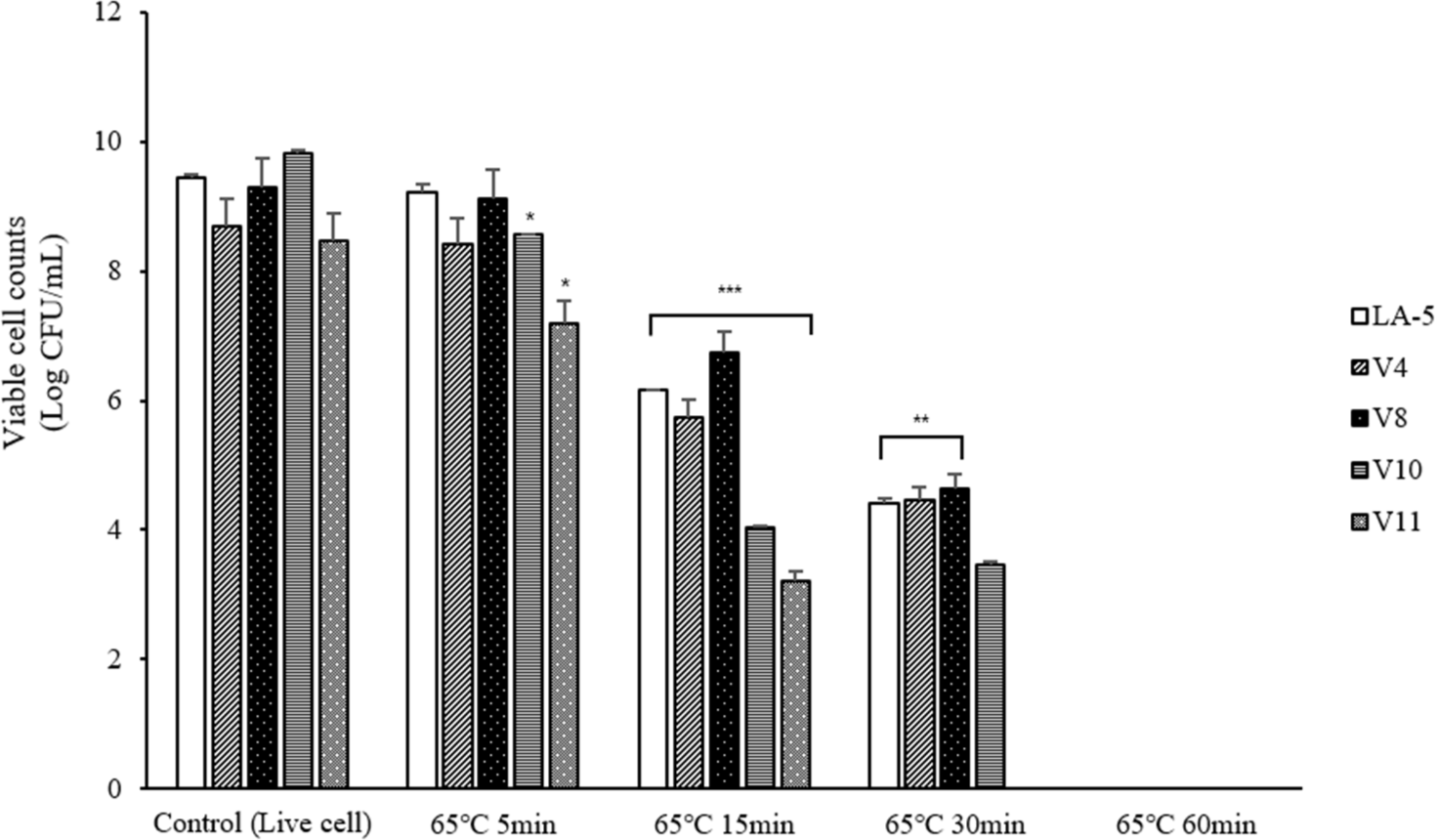
As a result of protein quantification analysis revealed that the protein content of dead cells heat-treated at 95°C was lower than that of cells killed heat-treated at 65°C. Furthermore, as the duration of the heat treatment increased, the protein content decreased. This result, shows that when heat treatment at 95°C, the protein was decomposed and the protein content was reduced (Fig. 3).
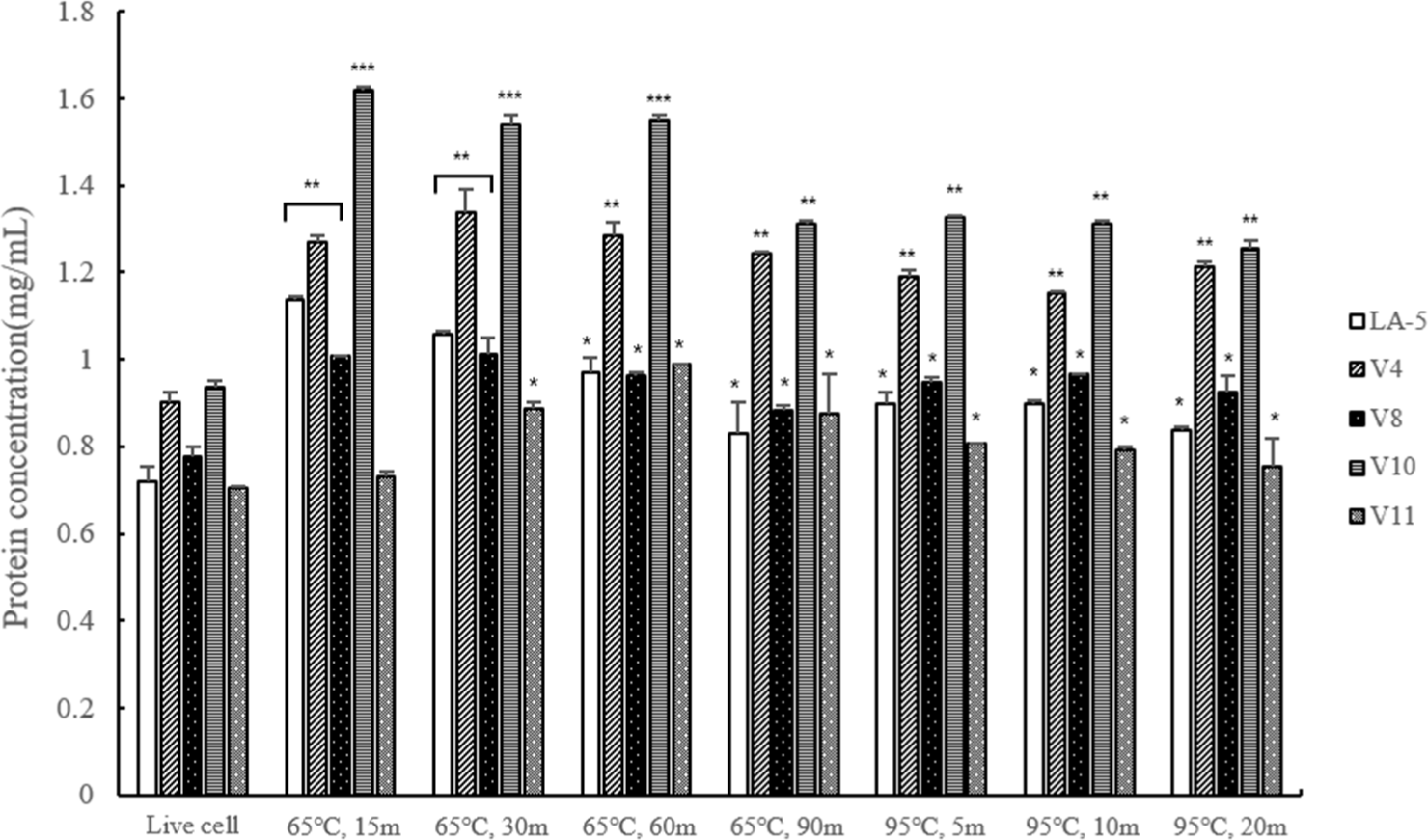
Conducting quantitative phosphate analysis to measure the content of LTA indirectly revealed that the content was lower at 95°C compared to the lactic acid bacteria heat-treated at 65°C, and similarly, the longer the heat treatment time, the lower the content. However, at 95°C, it was confirmed that there was no difference over time (Fig. 4).
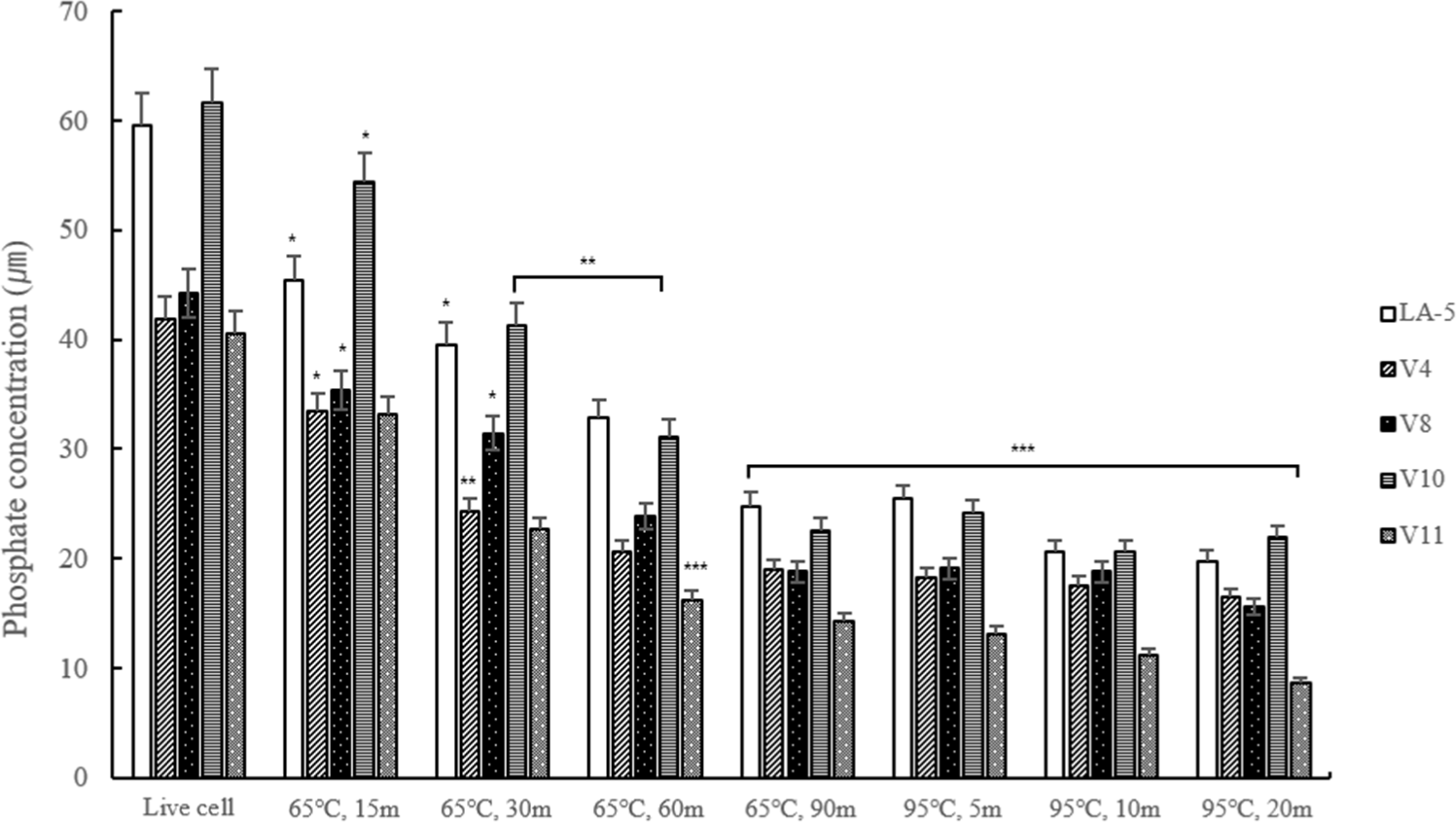
The Trolox equivalent antioxidant capacity assay measures the ability of a compound to eliminate or scavenge radicals compared to Trolox as an antioxidant reference (Leung et al., 2009). The results of the ABTS assay are shown in Fig. 5A. Heat-treated dead cells showed higher antioxidant capacity than live cells. In particular, ABTS radical scavenging activity was higher in V4 and V10 heat-treated at 65°C for 60 min than Enterococcus faecalis EF-2001, a commercial strain of dead cells.
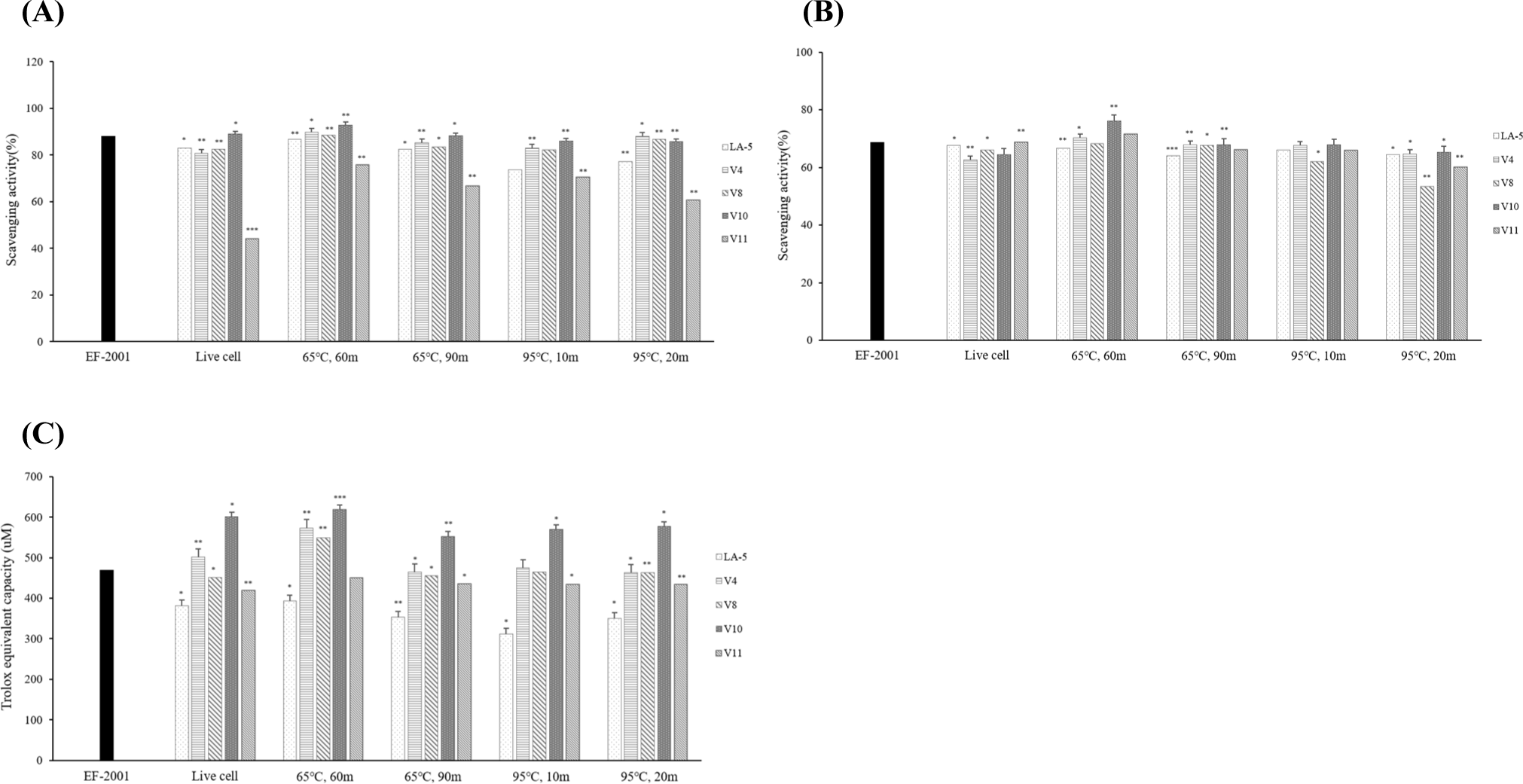
DPPH is a stable free radical that has been widely accepted as a tool for estimating the free radical-scavenging activity of antioxidants (Lin and Chang, 2000). Based on the Trolox standard curve, DPPH radical scavenging activity values were statistically analyzed. According to the results (Fig. 5B), heat-treated dead cells at 65°C for 60 min showed higher antioxidant activity than live cells. Except for heat-treated dead cells at 65°C for 60 min, the other heat-treated dead cells showed antioxidant activity similar to live cells. In addition, it was confirmed that V4, V10, and V11 heat-treated at 65°C for 60 min showed higher antioxidant activity than E. faecalis EF-2001, a commercial strain of dead cells.
FRAP analysis is a method of verifying oxidative activity through electron donation ability; when ferric tripyridyltriazine (Fe3+-TPTZ), which acts as an oxidizing agent, and antioxidants react, the reduction to blue ferrous tripyridyltriazine (Fe2+-TPTZ) is quantified through absorbance measurement (Spiegel et al., 2020). When compared to LA-5 heat treated under the same conditions as the V strain, all strains showed higher iron-reducing abilities than LA-5. It was confirmed that the V4, V8, and V10 strains heat-treated at 65°C for 60 min showed higher iron-reducing ability than E. faecalis EF-2001, the commercial strain of dead cells (Fig. 5C).
In the three antioxidant assays (ABTS, DPPH, and FRAP), V10 showed the highest antioxidant activity. Thus, L. plantarum V10 was selected as the final strain.
LTA is a key component of the cell wall in Gram-positive bacteria, and it has been the focus of numerous recent studies. While considerable research has been conducted on the LTA of pathogenic Gram-positive bacteria, studies on the LTA of LAB remain limited.
The LTA of dead cells was extracted and linearity was obtained through HPLC analysis with R2=0.9953. Based on the standard curve of LTA from Staphylococcus aureus (Sigma-Aldrich), the LTA content was statistically analyzed. As a result of analyzing the LTA content of heat-treated dead cells, it was confirmed that the commercial strain EF-2001 was 552 ppm, V10 heat-treated at 65°C for 60 min was 1,244 ppm, V10 heat-treated at 65°C for 90 min was 617 ppm, V10 heat-treated at 95°C for 10 min was 582 ppm and V10 heat-treated at 95°C for 20 min was 414 ppm (Table 6). It was confirmed that the LTA content of heat-treated at 65°C for 60 min was the highest. Except for heat-treated dead cells at 95°C for 20 min, the other heat-treated dead cells were higher than that of commercial strains EF-2001. As the LTA content decreased, the peak resolution also decreased (Fig. 6).
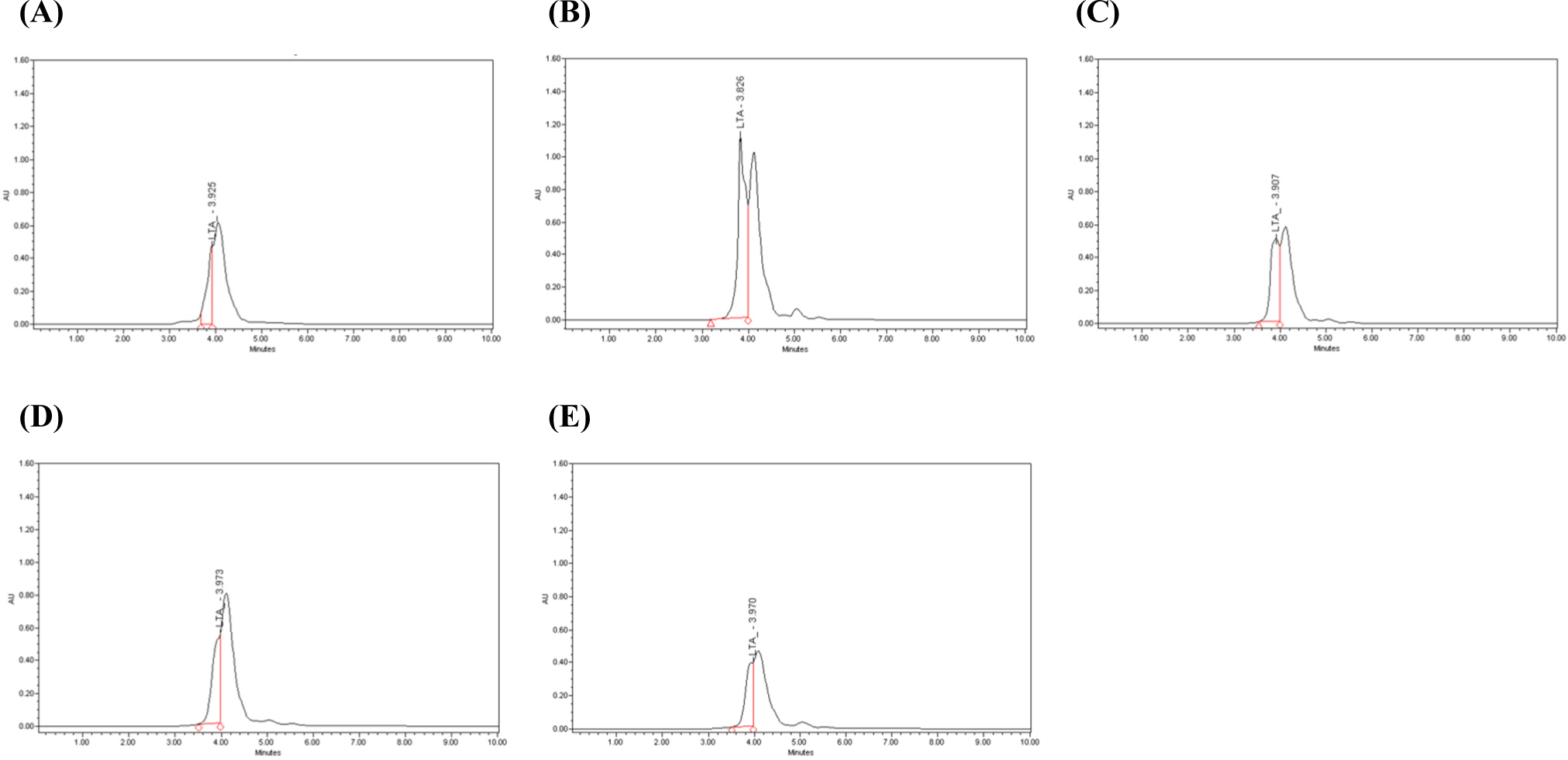
MTT is an indicator of mitochondrial activity and is primarily used as an index of cell survival. Active mitochondrial dehydrogenase in viable cells cleaves MTT to produce formazan, which is directly correlated with the number of metabolically active cells (Carreño et al., 2021). According to the experimental results, live cells showed no cytotoxic or growth-promoting activity at any concentration. In addition, the heat-treated dead cells were not able to confirm cytotoxicity or growth-promoting activity at the remaining concentration except for the concentration of 107 CFU/mL of heat-treated dead cell at 95°C for 20 min (Fig. 7). Live cells and heat-treated dead cells showed 80% or more cell viability; therefore, it was confirmed that live and dead cells were not cytotoxic to macrophages.
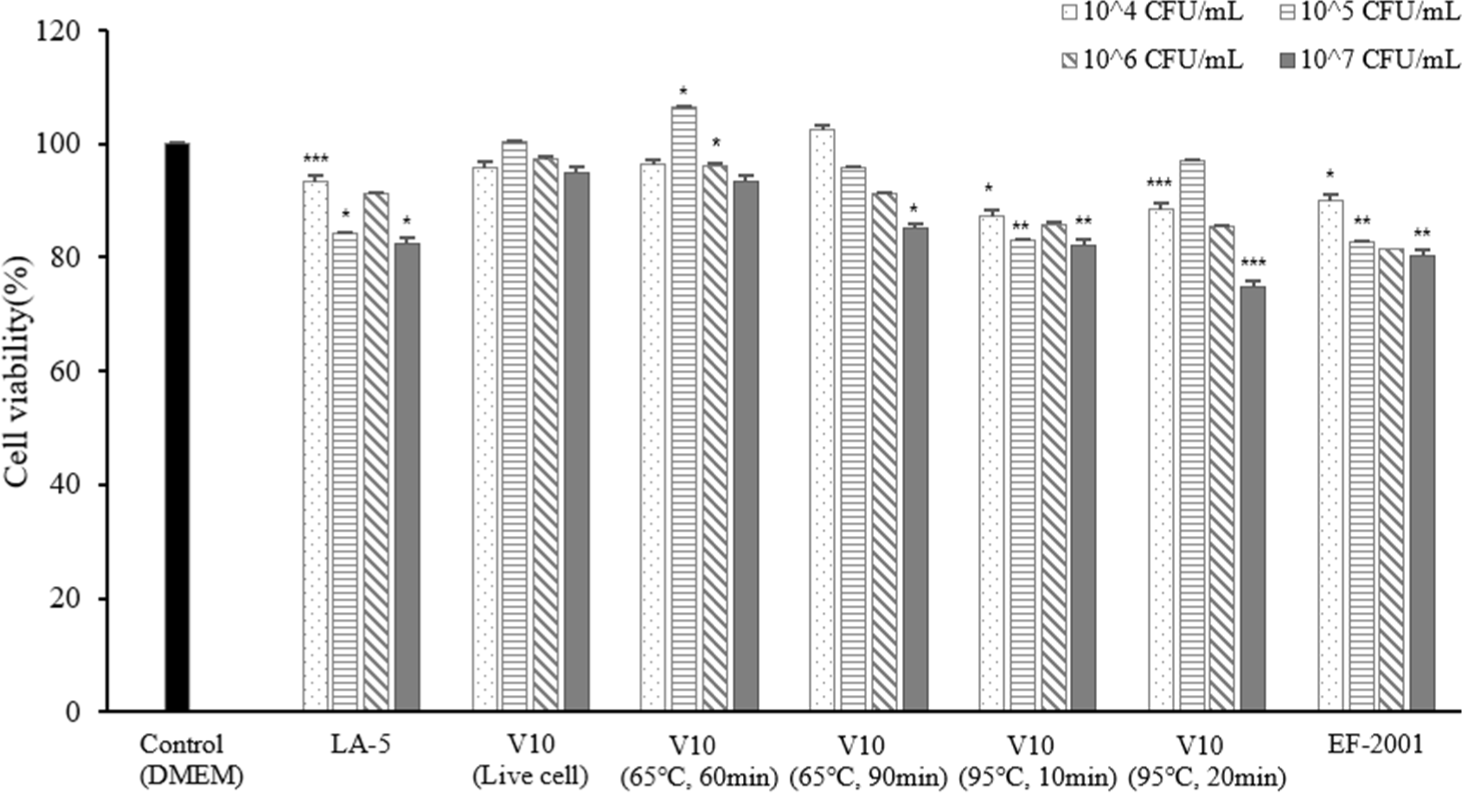
NO is a signaling molecule produced by various cells, including macrophages, that plays a crucial role in immune responses. NO production contributes to the elimination of pathogens and modulation of inflammatory processes (Lee et al., 2017a).
To evaluate their anti-inflammatory potential, the effects of live and heat-treated dead cells on NO production were examined in LPS-induced RAW 264.7 cells. Compared to the LPS-negative group, treatment with LPS significantly increased NO production. However, treatment with live cells and heat-treated dead cells inhibited NO production in a dose-dependent manner, except for 107 CFU/mL live cells (Fig. 8).
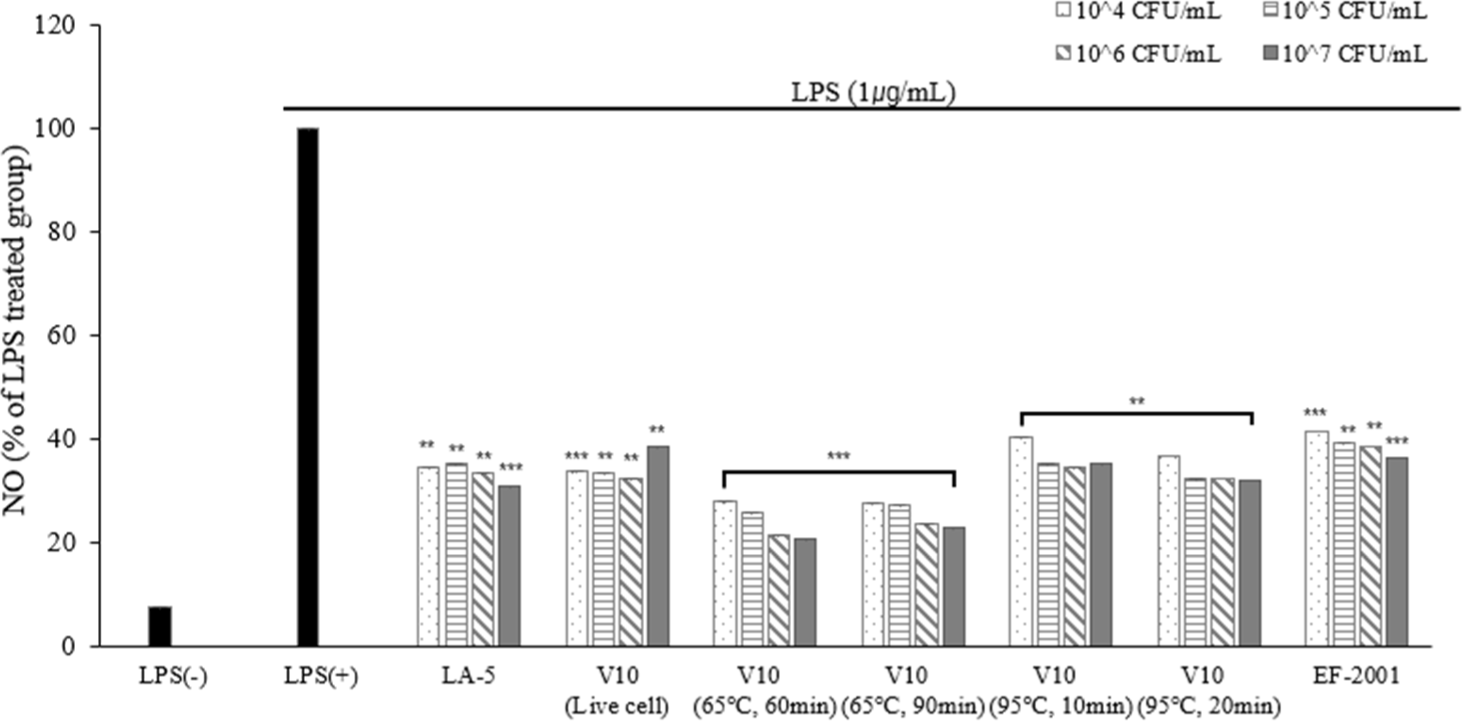
To maintain the immune balance, there must be direct and indirect interactions between immune cells. Cytokines can induce the proliferation, differentiation, and changes in the function and activity of various immune cells. Diseases are mostly associated with inflammation and inflammatory cells secrete substances that induce inflammation. The substance secreted at this time is called an inflammatory cytokine, which increases when environmentally harmful factors, viruses, and drugs enter the body (Barland et al., 2004). In this study, the expression levels of TNF-α, IL-1β, and IL-6 were measured.
According to the results of cytokine measurement using ELISA, three cytokines (TNF-α, IL-1β, and IL-6) production was significantly lower than LPS (+) group in live and dead cells (Fig. 9). Lower levels of TNF-α cytokines were confirmed in dead cells than in live cells. The low expression level of TNF-α from macrophage effect by strains prevents the activation of pro-inflammatory cytokines which are produced by the expression of TNF-α. Based on these results, anti-inflammatory activity can be found in both live and dead cells. In addition, it was confirmed that the cytokine levels of the three cytokines (TNF-α, IL-1β, and IL-6) were the lowest in heat-treated dead cells at 65°C for 60 min.
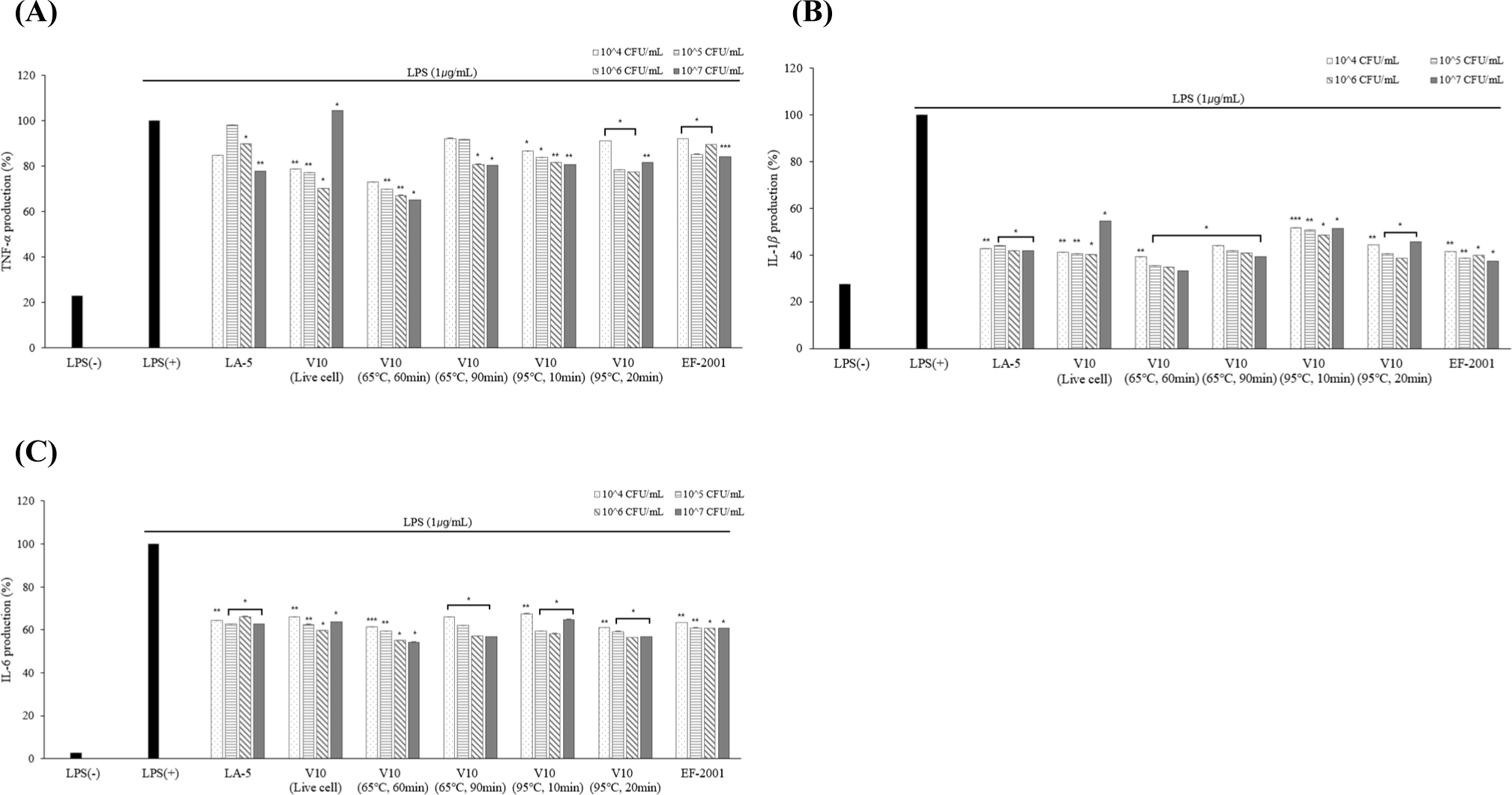
Therefore, the final heat treatment condition for dead cells was set to 65°C for 60 min to indirectly evaluate the degradation rate of the dead cells via digestive enzyme treatment.
SDS-PAGE analysis was performed using commercial enzymes (amylase, pepsin, and oxgall) to indirectly evaluate whether heat-treated dead cells underwent decomposition in the human digestive system following ingestion.
Following treatment with 10% amylase, the degradation of dead cells was evaluated under various conditions (5, 15, and 30 min) by sodium dodecyl sulfate-polyacrylamide gel electrophoresis. No evidence of cell decomposition was observed under any of these conditions. Considering that the average duration of mastication during human digestion is approximately 5 min, the amylase treatment time was standardized to 5 min.
Subsequent treatment with 0.3% pepsin (pH 3.0) was performed, and the degradation of dead cells was assessed using SDS-PAGE at different time intervals (30, 60, and 120 min). No degradation was observed under any of these conditions. Because the average gastric transit time in humans is approximately 30 min, the pepsin treatment time was set to 30 min.
SDS-PAGE analysis was conducted to assess degradation during oxgall treatment (0.3%) over extended periods (6, 12, and 24 h). The results indicated the partial decomposition of dead cells, with some molecules degraded into smaller fragments (Fig. 10).
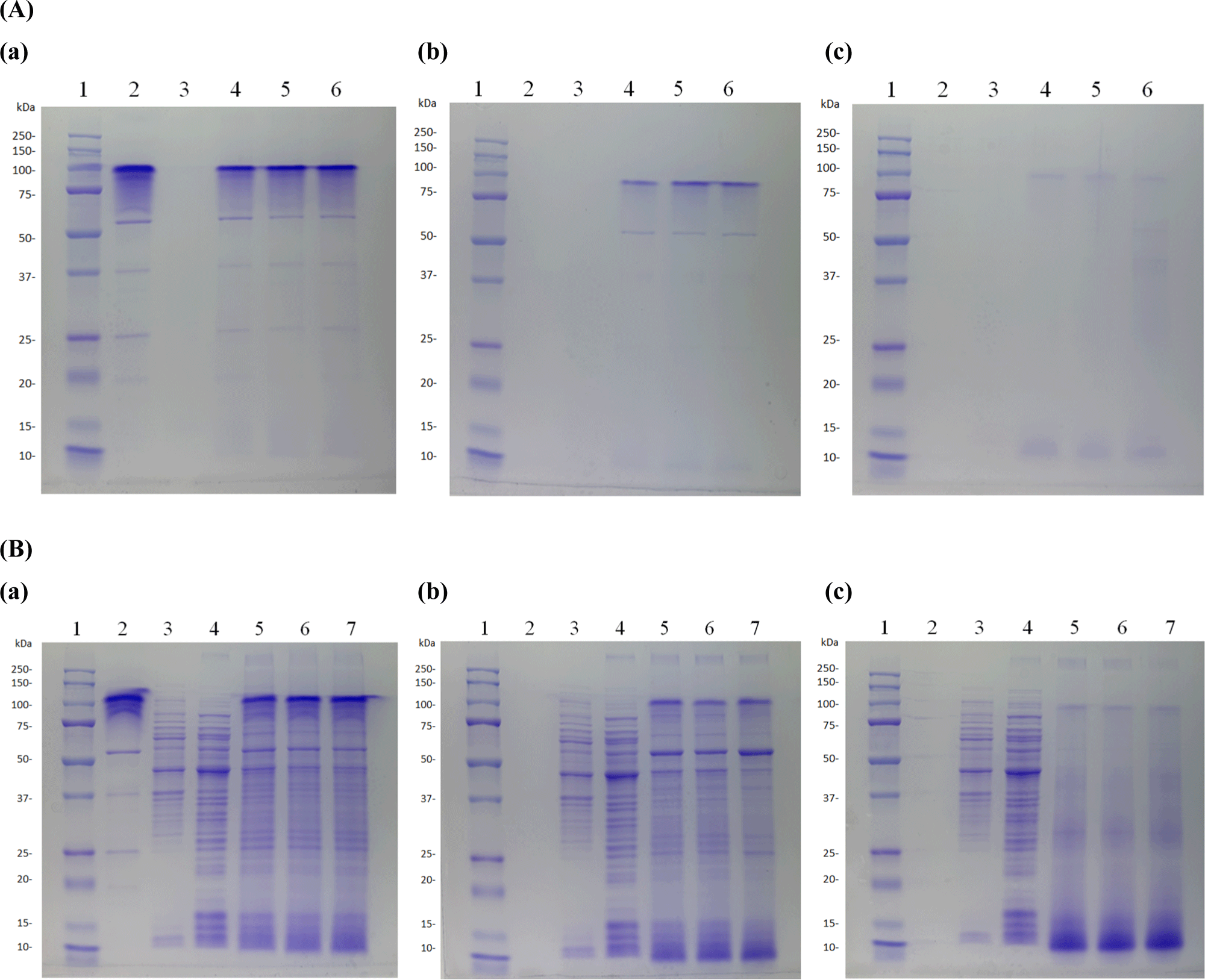
In conclusion, dead heat-treated cells did not undergo degradation upon treatment with 10% amylase or 0.3% pepsin (pH 3.0), confirming their ability to reach the intestine. However, partial decomposition into smaller molecular fragments was observed after treatment with 0.3% oxgall.
Discussion
Heat-treated dead cells with antioxidant and immune-enhancing properties are known to have beneficial physiological and immunological effects. In particular, the functional properties of dead heat-treated cells vary with temperature and time conditions (de Almada et al., 2016). In this study, we confirmed the functional and immunological effects of HK-LAB isolated from Vietnamese feces by varying the heat-treatment temperature and time. In addition, tolerance to decomposition in the human gastrointestinal tract has been indirectly confirmed using commercial enzymes.
Six strains used in this study were derived from Vietnamese feces. One strain of L. salivarius, four strains of L. plantarum, and one strain of L. fermentum were selected through basic physiological activity verification. Of the six strains tested, four with potential for use as commercial lactic acid bacteria were selected through verification of their basic physiological activity.
All bacteria did not survive under heat treatment conditions of 65°C for 60 min or 95°C for 10 min or longer. In protein quantification, the protein content was higher at 65°C compared to 95°C, and the longer the heat treatment time, the lower the protein content. It means that the protein content was reduced due to degradation of the protein at 95°C. In addition, to indirectly measure the LTA content, we conducted quantitative analysis of phosphate, a component of LTA. The results indicated that the phosphate content was lower in heat-treated dead cells at 95°C compared to those treated at 65°C, indirectly suggesting a higher LTA content at 65°C.
The antioxidant activity of the dead cells was confirmed for each heat treatment condition and compared with that of live bacteria. E. faecalis (EF-2001) is a Gram-positive bacterium with immunomodulatory and preventive properties. According to the research results of Choi et al. (2018), heat-killed E. faecalis (EF-2001) has various beneficial effects on human health, including anti-allergic, anti-inflammatory, and antitumor activities. Through the results of antioxidant activities (ABTS, DPPH, FRAP), heat-treated dead cells at 65°C for 60 min showed higher antioxidant activities than the live cells and EF-2001. V10 strain showed the highest antioxidant activity. These results are similar to those of other studies, showing that dead cells have a better antioxidant effect than live cells (Jang et al., 2018).
A previous study showed that extracted and purified LTA, a cell wall component of dead cells, inhibited cytokine production and analyzed the structure of LTA according to strain in Gram-positive bacteria (Lu et al., 2022; Morath et al., 2001). However, few studies have confirmed LTA content of LTA, HPLC analysis was performed to confirm the LTA content of dead cells following heat treatment. As a result of the analysis, except for the dead cells treated at 95°C for 20 min, the LTA content of heat-treated dead cells was higher than that of the commercial strain EF-2001, and the dead cells treated at 65°C for 60 min showed the highest LTA content.
Subsequently, the immunomodulatory effect of dead cells was confirmed. As a result of NO analysis, NO production was inhibited as the concentration of the dead cells increased, and the dead cells treated at 65°C for 60 min showed the lowest oxidation inhibition rate. In addition, the cytokines (TNF-α, IL-1β, and IL-6) of the dead cells were significantly lower than those of the LPS (+) group in a concentration-dependent manner, and, similar to the NO results, were the lowest in the dead cells heat-treated at 65°C for 60 min. This is consistent with previous studies showing that dead cells have similar or higher immune effects than live cells (Li et al., 2009). Based on these findings, the LTA content is expected to be closely associated with the immune response, highlighting the need for further research in this area.
Finally, SDS-PAGE was performed to indirectly evaluate whether heat-treated dead cells decompose in a simulated gastrointestinal environment. The results confirmed denaturation when artificial digestive enzymes were used in the presence of oxgall. Dead cells can be partially degraded by digestive enzymes while retaining their immune-stimulatory effects, thereby improving the intestinal environment (Taverniti and Guglielmetti, 2011). This finding indirectly suggests that heat-treated dead cells can maintain their immunomodulatory properties even when partially decomposed during digestion.
Conclusion
In this study, we confirmed the immunological effects of heat-treated dead cells isolated from Vietnamese feces, and demonstrated that heat-treated dead cells with immunomodulatory functions in the Southeast Asian market can be utilized as functional food ingredients.
Recently, active research has been conducted on the immunological effects of heat treatment on dead cells. However, studies that specifically analyze the content of LTA, a cell wall component of dead cells, remain insufficient. Further research in this area is required. In addition, because the method of inducing immune responses varies depending on the structural characteristics of LTA, additional research is needed to analyze not only the content, but also the structural characteristics of LTA. This study provides basic data for the development of functional food ingredients that utilize dead cells in the Southeast Asian market.