Introduction
Diabetes, an endocrine and metabolic disease, has become the third most non-infectious chronic disease threatening human health. Type-2 diabetes mellitus (T2DM) takes up more than 90% of people with diabetes and has become a major public health issue worldwide (Yan et al., 2019). It is characterized by increased blood glucose level, which cause damage to the body’s systems, particularly blood vessels and nerves (Rittiphairoj et al., 2019).
α-Glucosidase, which is a digestive enzyme present in the membrane of small intestine brush border, hydrolyzes disaccharides and/or polysaccharides into monosaccharide units for the digestion and absorption of carbohydrates. The absorption of carbohydrates by α-glucosidase generally progresses rapidly in the upper part of the small intestine, leading to a sharp rise in postprandial blood glucose levels. Therefore, it is essential to inhibit α-glucosidase and α-amylase in the postprandial glycemic management of patients with T2DM and pre-diabetes by reducing the post-prandial blood glucose level increasing after carbohydrate diet (Ali et al., 2006).
Short-chain fatty acid (SCFA) produced by intestinal microbes fermenting carbohydrate has beneficial effects on humans; and a deficiency of SCFA production is associated with T2DM (Zhao et al., 2018). Butyrate, acetate, and propionate are SCFAs that are fermented by enterobacteria from dietary fiber and take an important role in energy metabolism (Cummings, 1981). In animal experiments, propionate affects the production of gluconeogenesis, liponeogenesis and protein in the liver, and acetate acts as a substrate for cholesterol synthesis (Schwiertz et al., 2010).
One of the major activities of the large intestinal microbiota is to decompose substrates such as resistant starch and dietary fiber, which are not totally hydrolyzed by host enzymes in the small intestine (Bird et al., 2000; Louis et al., 2007; Topping and Clifton, 2001). Medium chain fatty acids (MCFA) seem to offer protection from lipo-toxicity and subsequent insulin resistance without caloric restriction (Wein et al., 2009). MCFAs reduced accumulation of fat and improved glucose tolerance. So, dietary supplements including MCFAs may help prevent obesity and peripheral insulin resistance (Turner et al., 2009).
Lactic acid bacteria are industrially important microorganisms because they have been safely used in production of fermentation and functional foods for a long time (Rhee et al., 2011). Pediococcus pentosaceus is one of the most commonly found strain in food and dairy environments (Banwo et al., 2013).
This study was conducted to investigate the antidiabetic effect and physiological characteristics of P. pentosaceus KI62 to determine whether P. pentosaceus KI62 isolated from kimchi can be applied as a functional food or fermented milk.
Materials and Methods
Using a modified MRS medium, the strain KI62 was isolated from homemade kimchi (Lim et al., 2011). The strain was incubated in Lactobacilli MRS broth (Difco, Detroit, MI, USA) as a growth medium at 37°C for 18 h.
A modified version of the method of determining α-amylase activity by Xiao et al. (2006) was used. Porcine pancreas α-amylase was purchased from Sigma (St. Louis, MO, USA). The substrate was prepared by boiling 0.5% soluble starch in distilled water for 5 min, and then leaving it to cool at room temperature. The sample (100 μL) and substrate (500 μL) were mixed in 400 μL of 0.04 M phosphate buffer (pH 5.8). After that, 0.5 mg/mL α-amylase solution (100 μL) was added, and the solution was incubated at 25°C for 10 min. The reaction was stopped by adding 100 μL 0.1M HCl, and then 100 μL of the solution was reacted with 1.5 mL iodine solution for 30 min at room temperature. Using a microplate reader (Spectramax Plus 384, Molecular Devices, Sunnyvale, CA, USA), the absorbance of the reactant was determined at 660 nm.
A α-glucosidase inhibition assay was carried out as previously described (Si et al., 2010), but it was modified as follows: Inhibitory activity was measured using α-glucosidase from Saccharomyces cerevisiae (Sigma). α-glucosidase (50 μL, 0.75 U/mL) and 0.2 M potassium phosphate buffer (pH 6.5, 50 μL) were mixed with 50 μL of the test sample. After pre-incubation at 37°C for 15 min, 3 mM 4-Nitrophenyl-α-D-glucopyranoside (pNPG, 100 μL) was added to the mixture. The enzymatic reaction was allowed to proceed at 37°C for 10 min and was stopped by the addition of 750 μL of 0.1 M Na2CO3. 4-Nitrophenol absorption was measured at 405 nm using a microplate reader.
The KI62 strain was inoculated to 1% in MRS broth and MRS broth containing 3% indigestible polysaccharide (maltodextrin), respectively, and cultured at 37°C for 18 h, and the supernatant was isolated to determine the contents of propionic acid, acetic acid, and butyric acid.
The five milliliter of the sample was diluted with distilled water until the color of sample faded, then a few drops of 1% phenolphthalein solution was added to it. The total acid was titrated and calculated according to the following formula.
V1: Amount of 0.1 N sodium hydroxide solution (mL) consumed in the titration
f: Titer of 0.1 N sodium hydroxide solution (1.000)
V2: Amount of sample (mL)
The four gram of the sample was added to 40 mL of ACN and then extracted for 30 min using a sonicator. The extracted solution was centrifuged at 1,770×g for 10 min to separate the supernatant. The separated supernatant was filtered with a 0.22 μm membrane filter, concentrated using a nitrogen concentrator, and analyzed by gas chromatograph / mass spectrometer (GC-MS). The GC-MS analysis conditions are shown in Table 1.
Chloroform-methanol extraction was used to extract butyric acid. Samples extracted with chloroform-methanol were concentrated using an evaporator, and then esterification of fatty acids to fatty acid methyl esters was performed according to the following method. The 20 mg of lipid and 2 mL of 0.5N NaOH/methanol was added and hydrolyzed on a heating block (100°C) for about 5 min. After cooling, 2 mL of 14% BF3/methanol was added and reacted for 5 min, followed by shaking with 2 mL of isooctane. After the reaction, 2 mL of saturated saline was added to the tube containing the sample. After stopping the plug and shaking it gently for 5 s, the isooctane layer was extracted and dehydrated using anhydrous sodium sulfate. A dehydrated fatty acid methyl ester test solution was received and injected into a gas chromatograph (HP-6890GC FID, Agilent Technologies, Santa Clara, CA, USA) for analysis. The gas chromatograph analysis conditions are shown in Table 2.
The experiment was carried out using the same method of measuring the butyric acid content.
To analyze the DNA sequence of lactic acid bacteria, universal primers 27F 5' (AGA GTT TGA TCC TGG CTC AG) 3' and 1492R 5' (GGT TAC CTT GTT ACG ACT T) 3' were used, and PCR was performed using a Big Dye terminator cycle sequencing kit v.3.1 (Applied BioSystems, Waltham, MA, USA). The amplification process was as follows: 95°C, 5 min; 95°C, 30 s; and 55°C, 2 min. It was performed 30 times at 68°C and 1 min and 30 s, and was finished at 68°C and 10 min. After removing the dNTP and the reactant, which do not participate in the reaction with the PCR product of the Montage PCR Cleanup kit (Millipore), sequencing was performed using primers 785F 5' (GGA TTA GAT ACC CTG GTA) 3' and 907R 5' (CCG TCA ATT CMT TTR AGT TT) 3' with an automated DNA sequencing system (model 3730XL, Applied BioSystems).
Antibiotic susceptibility, enzyme activity, pH and bile tolerance, antimicrobial activity, and adherence assay were conducted to measure probiotic property. The antibiotic susceptibility of P. pentosaceus KI62 was tested using the broth micro-dilution procedure (Phillips, 1991). The LAB Susceptibility test medium with cysteine (LSM-C), which consists of a mixture of Iso-Sensitest broth (90%) and MRS broth (10%), supplemented with 0.3 g/L L-cysteine (Klare et al., 2007), was used as the medium. The enzyme activity of strain was determined using an API ZYM kit (bioMérieux, Lyon, France). pH tolerance was tested as described by Clark et al. (1993). Bile tolerance was tested according to method of Gilliland and Walker (1990). The P. pentosaceus KI62 strain culture was inoculated into MRS broth containing 0.05% L-cysteine (Sigma) with/without 0.3% ox gall (Sigma). According to method of Gilliland and Speck (1977), antimicrobial activity of strain was measured for Escherichia coli ATCC 21985, Salmonella Typhimurium ATCC 14028, Listeria monocytogenes ATCC 15313, and Staphylococcus aureus ATCC 6538. The intestinal adhesion ability of the strain was performed using HT-29 cells according to method of Kim et al. (2008). After culturing the strain and the cells together, the number of strains adhered to the cells was counted using a BCP plate count agar.
Results and Discussion
After collecting 40 kinds of kimchi in each region, 167 single colonies forming yellow colonies were isolated using a modified MRS medium.
To select strong inhibitory activities of α-amylase and α-glucosidase, we determined the α-amylase and α-glucosidase inhibitory activities of 167 kinds of isolated strain in kimchi. The KI62 strain exhibited α-amylase and α-glucosidase inhibitory activity of 94.86±3.30% and 98.59±0.52%, respectively (Table 3). Because the dietary habits of Korean people include far more carbohydrates than those of western countries, it is nesessary to combine the mechanisms of inhibiting carbohydrate and fat absorption in order to improve obesity (Jang and Jeong, 2010).
Strain | α-Amylase inhibition | α-Glucosidase inhibition |
---|---|---|
KI62 | 94.86±3.30 | 98.59±0.52 |
When the KI62 strain was inoculated in MRS broth, the contents of the SCFA were propionic acid 5.95±1.66 mg/kg, acetic acid 1.15±0.00 g/100 mL, and butyric acid 2.38±0.02 g/kg. On the other hand, when the KI62 strain was inoculated in MRS broth with maltodextrin, the contents of the SCFA were propionic acid 18.05±1.85 mg/kg, acetic acid 1.12±0.07 g/100 mL, and butyric acid 2.19±0.061 g/kg (Fig. 1).
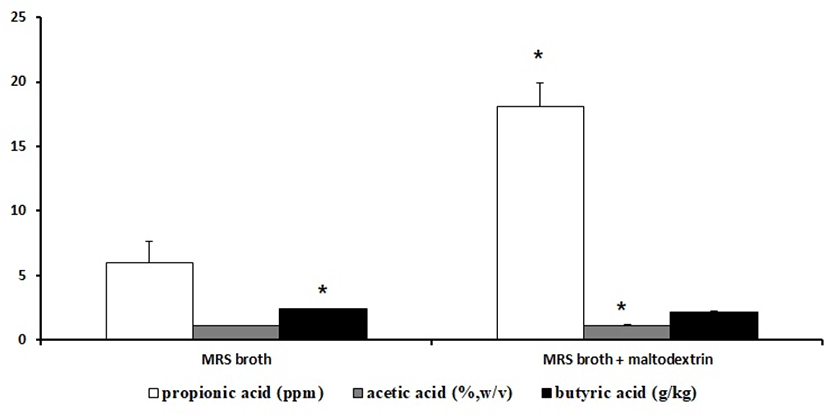
Meanwhile, the contents of the MCFA in MRS broth were C8 0.214±0.007 mg/kg, C10 0.250±0.011 mg/kg, and C12 0.223±0.035 mg/kg. On the other hand, the contents of the MCFA in MRS broth with maltodextrin were C8 0.262±0.031 mg/kg, C10 0.279±0.021 mg/kg, and C12 0.203±0.009 mg/kg (Fig. 2).
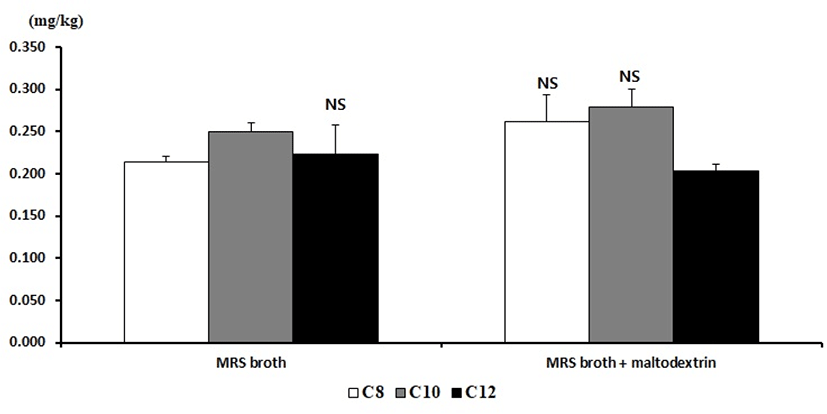
Following sequence analysis, it was identified as P. pentosaceus with a similarity of 99% (Data not shown). On the basis of previous studies, it was named P. pentosaceus KI62.
Table 4 shows the MIC values obtained for the 16 kinds of different antibiotics tested in P. pentosaceus KI62. The penicillin-G and rifampicin MIC value were the lowest among the antibiotics. P. pentosaceus KI62 showed the highest vancomycin MIC. Banwo et al. (2013) reported that vancomycin resistance of pediococci is prevalent, but, fortunately, it was thought to be endogenous for a modified precursor ending in D-Ala-A-lactate. Similarly, resistance to aminoglycosides such as kanamycin, gentamicin, and streptomycin is also an inherent characteristic of Pediococcus spp. (Hummel et al., 2007). According to Danielsen et al. (2007), penicillin-G, chloramphenicol, and erythromycin were consistent with reports of active antibiotics against the Pediococcus spp. strain.
According to the European Food Safety Authority (EFSA, 2008) and the Scientific Committee for Animal Nutrition (Chesson et al., 2002), P. pentosaceus KI62 was susceptible to clindamycin and erythromycin. However, according to those same sources, it was resistant to gentamycin, kanamycin, streptomycin, ampicillin, tetracycline, clindamycin, erythromycin, and chloramphenicol because the MICs were equal to or higher than the breakpoints. These results show that the P. pentosaceus KI62 strain generally has antibiotic tolerance.
The enzyme activities of the P. pentosaceus KI62 strain are shown in Table 5. The KI62 did not produce β-glucuronidase, a harmful enzyme related to the inducement of toxins, carcinogenesis, and mutagens (Dabek et al., 2008). Notably, the activity of leucine arylamidase was 5 degrees, and that of valine arylamidase was 4 degrees. β-galactosidase and β-glucosidase are useful enzymes. Especially, the KI62 displayed β-galactosidase activity that can relieve the symptoms of lactose intolerance because β-galactosidase hydrolyzes lactose to galactose and glucose in milk (De Verse et al., 2001). According to Tzanetakis and Litopoulou-Tzanetaki (1989), the average enzyme activity of leucine arylamidase and valine arylamidase among 49 strains of P. pentosaceus isolated from raw goat milk and Feta and Kaseri cheese were 4.98 degrees and 4.92 degrees, respectively, and the average enzyme activity of β-galactosidase and β-glucosidase were 4.61 degrees and 2.99 degrees, respectively. These results showed that the enzyme activity of leucine arylamidase and valine arylamidase was similar, while β-galactosidase and β-glucosidase showed slightly lower enzyme activity.
A value ranging from 0 to 2 is assigned to the standard color: zero represents a negative; 5 represents a reaction of maximum intensity. Values 1 through 4 represent intermediate reactions depending on the level of intensity. The approximate activity may be estimated from the color strength: 1 corresponds to the liberation of 5 nanomoles; 2, to 10 nanomoles; 3, to 20 nanomoles; 4, to 30 nanomoles; and 5, to 40 nanomoles or more.
To be used as probiotic, bacteria should have strong resistance to acid and bile (Lee and Salminen, 1995). Acid and bile tolerance is required for bacterial growth and is involved in the defense mechanisms in the intestine. The bacteria should also survive during passing through the stomach as well as in food (Lee and Salminen, 1995; Henriksson et al., 1999; Succi et al., 2005). The pH of the stomach is 2-3, and the food passes through the stomach for a period of 2-3 h (Maragkoudakis et al., 2006).
As a result of incubation for 7 h in MRS broth, the log value of strain was reached at 9.20. But, the log value of strains was 8.44 in MRS broth adding 0.3% oxgall. Consequently, the survival rate of P. pentosaceus KI62 in MRS broth containing 0.3% bile was 91.67% (Fig. 3). P. pentosaceus KI62 has probiotic potential because a relatively high percentage of the strain survived in MRS broth adding 0.3% bile salt.
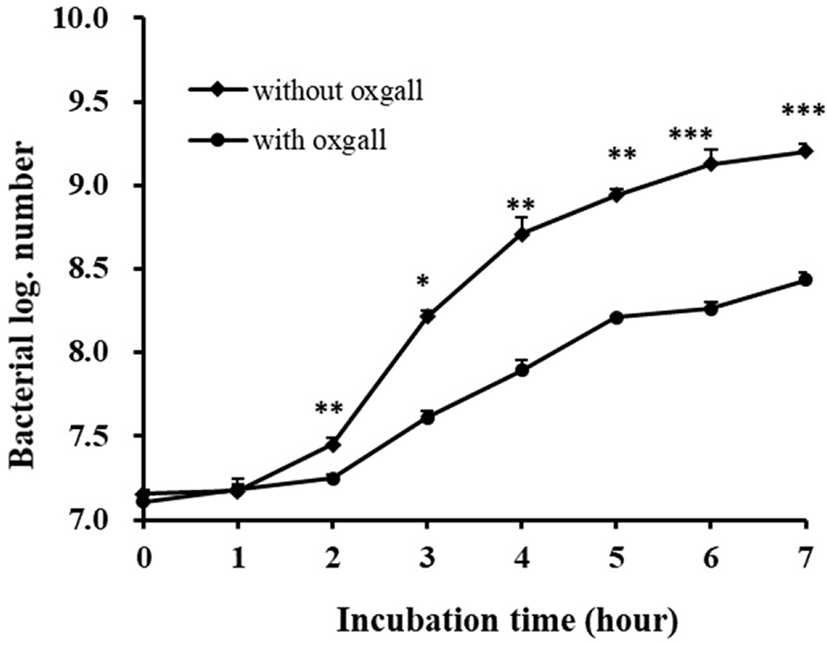
Fig. 4 shows the pH tolerance of P. pentosaceus KI62. When incubation for 3 h in pH 2.0, it had a survival rate of 98.63% and the growth of the strain was not influenced by pH 3, 4, or 6.4. These results show that the strain was more resistant than Vidhyasagar and Jeevaratnam (2013), who reported that the number of bacteria decreased by 1–2 log when inoculated into MRS broth with P. pentosaceus at pH 2 for 2 h.
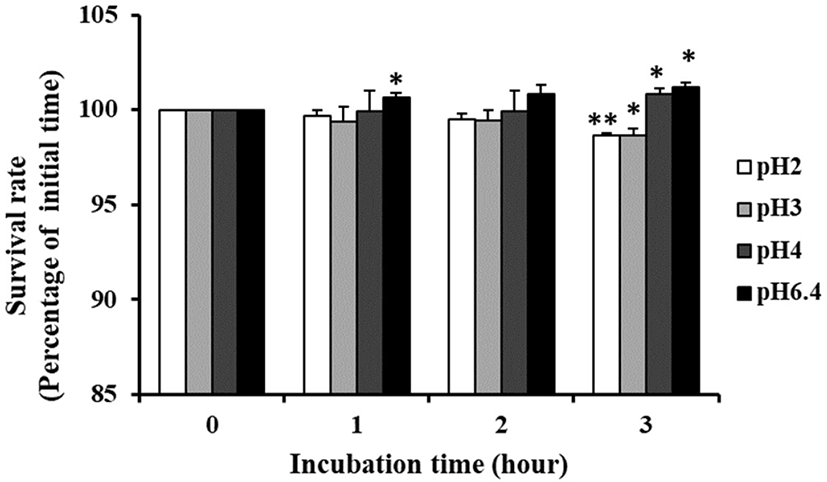
In other words, P. pentosaceus KI62 has the best acid and bile tolerance ability because a relatively high percentage of the strain survived in MRS broth adding 0.3% bile salt as well as under a highly acidic condition.
Some strains of LAB produce a variety of antimicrobial substances that can prevent the growth of pathogenic and spoilage bacteria. The antimicrobial metabolites of LAB include hydrogen peroxide, organic acid, bacteriocins, and diacetyl (Ahmadova et al., 2013). To improve human health, probiotics have to decrease the incidence of pathogenic bacteria. Therefore, the process of choosing beneficial probiotics in the presence of pathogenic bacteria is important (Kesarcodi-Watson et al., 2012).
P. pentosaceus KI62 showed resistance to E. coli, S. Typhimurium, L. monocytogenes, and S. aureus at rates of 29.41%, 38.10%, 51.72%, and 50.47%, respectively (Table 6). The pH value of pathogens after incubation for 6 h was around 5.24–6.24, whereas the pH value of a culture with P. pentosaceus KI62 and pathogens was around 4.67–4.75. Although the lactic acid produced during culture was not large, it was found to have an effect on antibacterial activity. Bao et al. (2010) investigated the ability for co-aggregation with pathogens of 11 strains isolated from traditional dairy products. The 11 strains showed resistance to E. coli, S. Typhimurium, L. monocytogenes, and S. aureus at rates of 10.5%–32.4%, 10.0%–29.7%, 11.0%–34.0%, and 17.7%–49.9%, respectively. These results showed that the P. pentosaceus KI62 strain exhibited higher overall antimicrobial activity, especially L. monocytogenes and S. aureus.
The adhesion to intestinal epithelium is one of the main screening criterion for choosing probiotics (Blum et al, 1999). This ability takes account of precondition for showing beneficial effects, such as the bar of enteropathogenic bacteria (Bernet et al., 1993; Lee et al., 2003). HT-29 cells are generally derived from colon carcinoma, and representing the property of a differentiated absorbent enterocytes. Lactobacillus rhamnosus GG was demonstrated to have great ability to adhere to the epithelial cell line in many previous studies (Gopal et al., 2001; Martín et al., 2005). As shown in Fig. 5, P. pentosaceus KI62 and L. rhamnosus GG adhered to HT-29 cell was 23.31% and 24.49%, respectvely. These results were higher than those of Vidhyasagar and Jeevaratnam (2013), who reported that 16% of P. pediococcus VJ13 adhered to Caca-2 cells. Thus, one can say that P. pentosaceus KI62 exhibits great adherence to the epithelial surface.
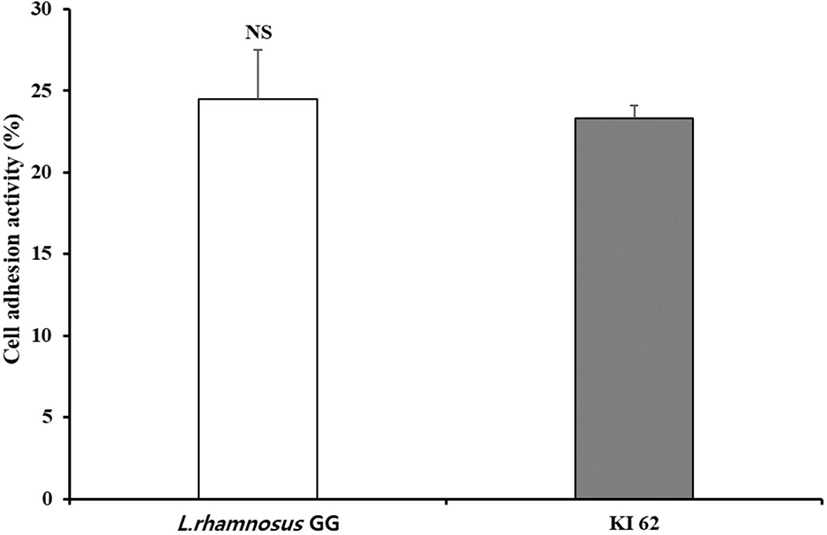
Conclusion
This study was conducted to investigate the anti-diabetic effects of P. pentosaceus KI62 selected from among LAB isolated from kimchi, and to study its physiological characteristics to confirm the potential of health functional food or fermented milk as a starter. On the basis of the nucleotide sequence of 16S rDNA gene, it was named P. pentosaceus KI62. The P. pentosaceus KI62 strain was observed to exhibit α-amylase and α-glucosidase inhibitory activity of 94.86±3.30% and 98.59±0.52%, respectively. The contents of SCFA in MRS broth containing 3% maltodextrin inoculated by P. pentosaceus KI62 were propionic acid 8.78±1.12 mg/kg, acetic acid 1.34±0.07 g/100 mL, and butyric acid 0.876±0.003 g/kg. The contents of MCFAs in MRS broth containing 3% maltodextrin inoculated by P. pentosaceus KI62 were C8 0.262±0.031 mg/kg, C10 0.279±0.021 mg/kg, and C12 0.203±0.009 mg/kg. In a comparison of sixteen different antibiotics, P. pentosaceus KI62 showed higher sensitivity to penicillin-G, rifampicin, and clindamycin, as well as the highest resistance to vancomycin and ampicillin.
P. pentosaceus KI62 has the best bile and acid tolerance ability. It showed resistance to E. coli, S. Typhimurium, L. monocytogenes, and S. aureus at rates of 29.41%, 38.10%, 51.72%, and 50.47%, respectively. It exhibited 23.31% adherence to the epithelial surface. These results demonstrate that P. pentosaceus KI62 has potential as a probiotic with anti-diabetic effects.