Introduction
Polar fats present in the milk fat globule membrane (MFGM), together with cholesterol and different proteins, are of nutritional and functional interest. The MFGM consists of a complex mixture of protein, polar, and nonpolar lipids, which constitute up to 90% of its dry weight (El-Loly, 2011). The most polar lipids in MFGM are phospholipids [phosphatidylcholine (PC), phosphatidylethanolamine (PE), phosphatidylinositol (PI), and phosphatidylserine (PS)], and sphingolipids (SM) (Bourlieu et al., 2018). Polar lipids are a major component of the cell membranes and are known to affect health as they play a fundamental role in cell membrane function. Dairy phospholipids (PLs) have been shown to reduce cholesterol, cardiovascular disease, inflammation and gastrointestinal infections, stress, and cancer, and are also known to affect neuronal differentiation (Contarini and Povolo, 2013).
The MFGMs containing polar lipids are released into the water phase by various mechanical treatments, such as heating, stirring, homogenization, or aeration during the manufacture of butter or cheese. Polar lipids in milk can be recovered from whey (Price et al., 2018), a by-product of cheese making, and butter serum (Rombaut et al., 2006b), a by-product of butter making.
Most of the recent commercial polar lipids are made from egg yolk and soybean. The low content of PL in dairy products makes it difficult to extract and concentrate PL at an industrial scale. Therefore, microfiltration and ultrafiltration are used to separate polar lipids from dairy products in MFGM fragments and lipoprotein particles. Polar lipids are easily separated from the serum phase by tangential micro- and ultrafiltration techniques that involve addition of water in a step by step manner to remove undesirable components, such as lactose, whey-proteins, and minerals (dialysis filtration) (Gassi et al., 2016). Supercritical fluid extraction has been reported to increase milk polar lipid content by selective removal of neutral lipids (e.g., triglycerides). To increase the polar lipid content of MFGM obtained through microfiltration, the use of supercritical carbon dioxide was employed, which reduced the concentration of neutral lipids from 21% to 4%, and the concentration of polar lipids increased from 9.6% to 19.7% (Astaire et al., 2003). However, these methods require a relatively high cost and have limitations on equipment, which makes industrial access difficult (Price et al., 2018).
Generally, for polar lipid extraction, it is suitable to use mixtures of hydrophobic and hydrophilic solvents. Hydrophobic solvent hexane or chloroform and hydrophilic solvent propanol or methanol are used (Le et al., 2011; Rombaut et al., 2005). Triglycerides require hydrophobic solvents for extraction, while amphiphilic polar lipid can be effectively extracted with a mixture of hydrophobic and hydrophilic solvents. However, non-polar solvents present a safety risk, and the use of solvents, like methanol, in the production of food materials is inadequate. Thus, a combination of water, preferably ethanol, is advantageous as a non-polar solvent and a polar counterpart.
Therefore, in this study, the extraction of butter serum and whey was evaluated to select suitable raw materials for polar lipid extraction. In addition, the optimum extraction process was established by comparing the polar lipid extraction rate according to the ethanol concentration and solid content. Finally, component analysis was performed and the anti-inflammatory activity of the polar lipids produced by the optimal extraction process was measured.
Materials and Methods
To prepare the phospholipid-enriched component, butter serums powder A and B were purchased from Corman (Limbourg, Belgium), and whey serum WPC60 and WPC70 were purchased from Agropur Inc. (Saint-Hubert, Quebec, Canada). Polar lipids extraction was performed using 85%, 90%, and 95% ethanol solution (60 L), instead of water, and powdered butter serums or whey serums (10 kg). Extraction was performed for 5 h, with stirring at 80–100 rpm at 60°C. After extraction, the mixture was separated into filtrate and precipitate using a 0.45 μm membrane filter (Whatman, Little Chalfont, UK). The filtrate was concentrated using a forced thin-film evaporator (EYELA, Tokyo, Japan). After concentration, the mixture was allowed to stand at 40°C for 30 min, and phospholipids and triacylglycerol were separated into an upper layer and a lower layer. The upper layer was used as the enriched polar lipid fraction.
The dry matter content was measured by the hot air oven method (IDF, 2010). Total lipid content was estimated gravimetrically using Röse-Gottlieb extraction (IDF, 1985). Protein content was measured using the Kjeldahl nitrogen determination method (IDF, 2001), and by using a protein conversion factor of 6.38. The ash content of the sample was obtained by ingesting 5 g of the sample at 550°C, cooling it to room temperature in a desiccator, and measuring the weight. Lactose was analyzed by the AOAC method and HPLC (Zygmunt et al., 1982). High-pressure liquid chromatography (HP 1100, Agilent, Massy, France) analysis was performed under the following conditions: the column was an Econosphere NH2 column (5 μm, 250 mm×4.6 m; Alltech Associate, Deerfield, IL, USA), the mobile phase comprised acetonitrile and water (75:25, v/v) at a flow rate of 1 mL/min and the column temperature was 35°C.
The HPLC- evaporative light-scattering detector (ELSD) analysis was carried out using an HPLC (Shimadzu, Kyoto, Japan) instrument equipped with two LC-10 Advp pumps, SCL-10 Advp gradient system, DGU-14 Advp module degasser, and Rheodyne manual injector with a 10 μL sample loop. The analytical column (150 mm×4.6 mm I.D. 3 μm) was packed with a silica normal-phase Spherisob SIL (Waters Technologies, Milford, MA, USA). Chromatographic separation was carried out using a linear binary gradient, according to the following scheme, 0 min: 4% B, 4 min: 12% B, 12 min: 94% B, and 17 min: 4% B. The total run time was 30 min per sample. Eluent A consisted of chloroform and eluent B consisted of methanol and acetic acid-triethylamine buffer (pH 4.5, acetic acid-triethylamine-water, 7.2/8.0/118, v/v/v). The flow rate of the eluent was 0.5 mL/min. An ELSD 2000 (Alltech, Deerfield, IL, USA) was used; the pressure of nebulizer gas (air) was maintained at 2.2 bar, and the drift tube temperature was set at 100°C.
Oxidation induction time was measured using Rancimat 743 (Metrohm, Herisau, Switzerland), in accordance with Cha and Choi’s method (1990), to determine the degree of rancidity upon heating of the whey protein concentrate (WPC). The sample (3 g) was oxidized by injecting air at a rate of 20 L/h at 100°C. The experiment was repeated three times to determine the degree of rancidity. In order to measure the oxidative stability of phospholipid from whey, 5% of whey protein phospholipid (WPL) was added to docosahexaenoic acid (DHA), and ASARCO oil (DSM Nutritional Products, Parsippany, NJ, USA), and egg phospholipids were used as control samples.
EVs were isolated from the culture supernatants of E. coli (KCTC 1039) and L. plantarum (KCTC 3108), as described previously (Lee et al., 2009; Kim et al., 2015). Briefly, E. coli and L. plantarum in nutrient broth was cultured at 37°C and centrifuged twice at 10,000×g for 15 min. Supernatants were filtered with a 0.22-μm vacuum filter. Then the resulting filtrate was subjected to ultracentrifugation at 150,000×g for 3 h at 4°C (Beckman Instruments, Fullerton, CA, USA). EVs were diluted in PBS and stored at −80°C.
The murine macrophage cell line, RAW 264.7 was obtained from the Korea Cell Line Bank (KCLB) and cultured in DMEM containing 10% FBS and 1% penicillin-streptomycin. The cells were incubated at 37°C and 5% CO2. The cell viability after WPL treatment was measured by MTT assay. Inflammation was induced by treating RAW 264.7 cells with 1 μg/mL of extracellular vesicles (EVs) from E. coli and 0.1–100 μg/mL of WPL for 24 h. Cytokines IL-6 and TNF-α secreted in the culture medium were quantified using an ELISA kit (R&D Systems, Minneapolis, MN, USA). For the detection of anti-inflammatory activity, RAW 264.7 cells were seeded onto 12-well plate and treated with either 1 μg/mL of EV from E. coli along with 0.1–100 μg/mL of WPL, or 1 μg/mL of EV from Lactobacillus plantarum and incubated for 24 h at 37°C. The cytokines IL-6 and TNF-α secreted in the culture medium were quantified using an ELISA Kit (R&D Systems).
All experiments were repeated three times, and the results were expressed as mean±SD. Statistical analysis was performed using the SPSS 10.0 program to determine the significance of each treatment (p<0.05) by using the analysis of variance (ANOVA) along with Duncan’s multiple range test.
Results and discussion
Table 1 shows the results of the component analysis of butter serum and whey powder, used as raw materials for the preparation of polar lipids. The fat content of butter serum was 27.5% and 12.5% for butter serum powder A and B, respectively, while WPC60 and 70 showed 25.3% and 20.3% fat, respectively. Butter serum powder (A 38.4% and B 46.8%) had higher lactose content than whey (WPC60 1.2% and WPC70 1.5%).
Milk PLs can be recovered from whey, or butter serum, the by-products of cheese, or cream-making, respectively. In the production of butter, MFGM fragments damaged by mechanical treatment are recovered in buttermilk or butter serum (Vanderghem et al., 2010). The MFGM fragments obtained as by-products of milk processing include PLs (glycerophospholipids and sphingolipids), neutral lipids (triacylglycerols), proteins, and glycoproteins (Dewettinck et al., 2008; Lopez, 2011). Buttermilk and butter serum has been reported to contain about 2 and 8 g/L of phospholipids, respectively (Rombaut and Dewettinck, 2006). According to a previous report (Boyd et al., 1999), commercial whey powders contain high proportion of lactose, ash, and calcium, but contain low proportion of protein and total lipids, and low protein-to-lipid ratios. The difference in composition is due to differences in the manufacturing processes or in the sources of whey.
Phosphorus content of the polar lipid-enriched fraction, obtained by extracting polar lipids from the by-products with alcohol, was measured (Table 2). The phospholipid content of the enriched fraction obtained from butter serum (A 36.7% and B 45.1%) was higher than that of whey powder (WPC60 30.5% and WPC70 31.0%). The yields were 21.0% and 12.0% for butter serum A and B, respectively, and 20.0% and 18.0% for WPC60 and WPC70, respectively (Table 2). Due to its high yield and phospholipid content, butter serum powder A was considered to be suitable for the preparation of polar lipid-enriched fraction. Whey powder did not have a detectable lactose content in the polar fraction, and the protein content was lower than that of butter serum. Although WPC60 showed slightly lower phospholipid content and yield, it was selected as a raw material for the production of polar lipid-enriched fraction, in consideration of the economic aspects. For the preparation of polar lipid fractions from MFGM fragments, it is preferable to use butter serum (Rombaut et al., 2006a) and whey (Rombaut et al., 2007a), which contain no or low amounts of casein.
The effect of ethanol extraction concentration with the change of the solids content was measured to determine the ethanol concentration and the amounts of solids for the extraction of polar lipids (Fig. 1). As the solid content of whey powder for extraction increased, the amount of polar lipid extraction increased. As the water content in ethanol increased, the content of polar lipids increased, and the phospholipid content increased. The main lipids contained in the polar lipid fraction were SM and PE, followed by PC (Fig. 1). The major components of polar lipids that make up MFGM are phospholipids and sphingolipids (mainly sphingomyelin, SM) (Mather, 2000; Ye et al., 2002).
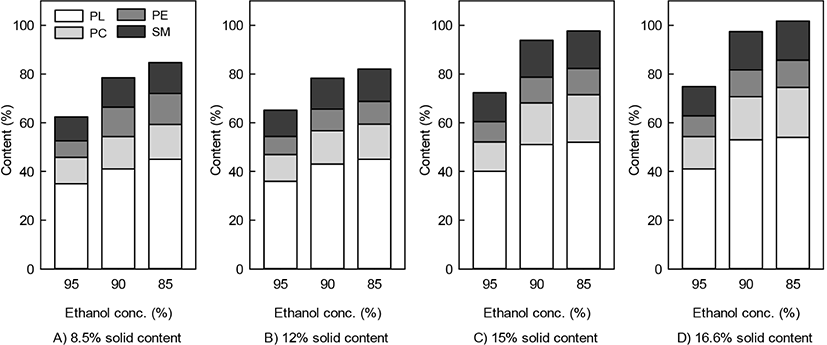
In particular, the highest PL content previously reported in dairy products was 45.8% on a dry basis in whey protein extracted with 70% ethanol at 70°C treatment (Price et al., 2018). According to the results presented in Fig. 1, the addition of 15.0% and 16.6% of whey powder and the use of 85% alcohol as the extraction solvent are the most suitable conditions for the extraction of 51.1% and 53.3% of PL, respectively.
After the selection of whey powder and extraction solvent, the extraction time and temperature were determined to be 60°C and 30 min, respectively, to establish an optimal process (data not shown). Fig. 2 shows an optimized extraction process for polar lipid WPL. The optimal production process (Fig. 2) yielded 40.26 kg of polar lipid product, WPL, using 200 kg of whey powder.
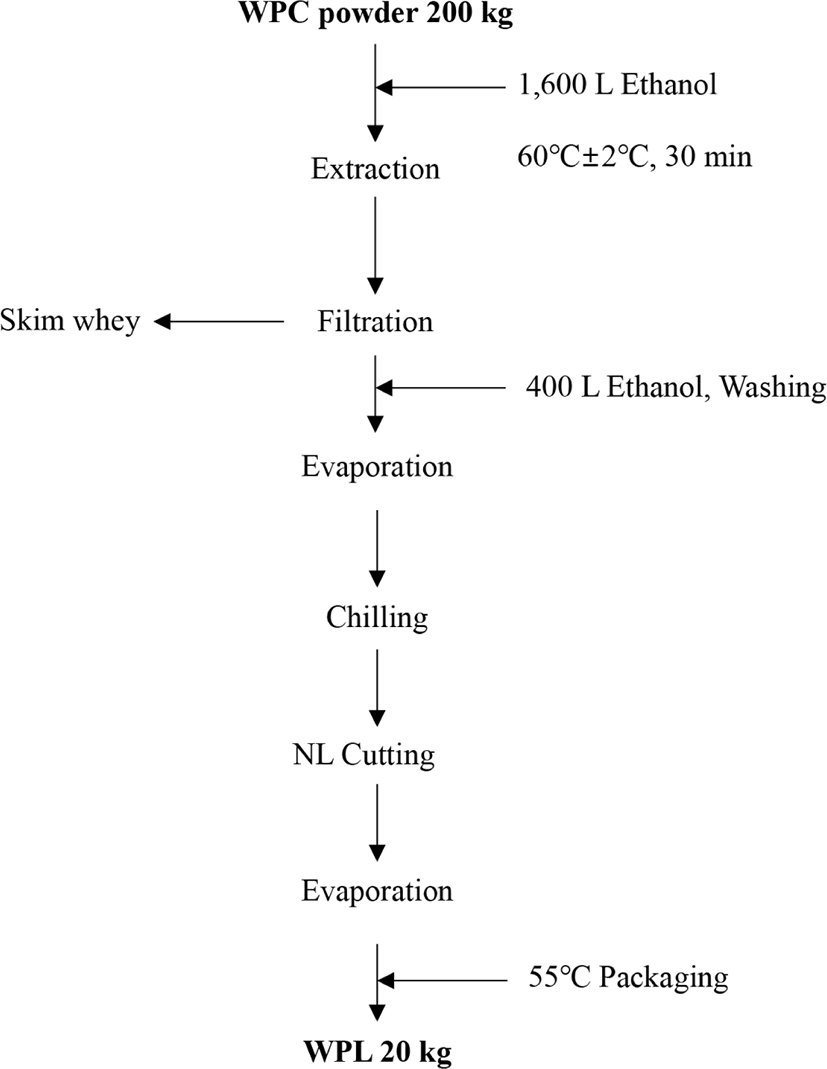
The lipid composition of WPL is shown in Table 3. The polar lipid content was 38.6%, and PC, SM, and PE showed 14.6%, 10.7%, and 10.1%, respectively. The PS and PI were found in low levels, 1.4% and 1.8%, respectively (Table 3). Polar lipid WPL is composed of phospholipids (mainly PC and PE) and sphingolipids (mainly SM). It also contains small amounts of glycolipids (Cerebroside: 9.79 mg/g, LacCer: 5.50 mg/g, GM3: 0.88 mg/g, GD3: 1.35 mg/g) in Table 4.
Sample | PS (%) | SM (%) | PC (%) | PE (%) | PI (%) | PL (%) |
---|---|---|---|---|---|---|
WPL (PL-enriched fraction) | 1.36 | 10.74 | 14.56 | 10.08 | 1.82 | 38.56 |
Sample | Concentration (μg/mg) | |||
---|---|---|---|---|
Cerebroside | LacCer | GM3 | GD3 | |
WPL | 9.79±0.78 | 5.50±0.35 | 0.88±0.01 | 1.35±0.01 |
It has been reported that the concentration of polar lipids in crude oil is between 9.4 and 35.5 mg/100 g. The main milk phospholipids are PE (19.8%–42.0%, w/w), PC (19.2%–37.3%, w/w), PS (1.9%–10.5%, w/w), and PI (0.6%–11.8%, w/w) (Rombaut et al., 2005; Rombaut and Dewettinck, 2006). The main milk sphingolipids are GluCer (2.1%–5.0%, w/w), LacCer (2.8%–6.7%, w/w), and SM (18.0%–34.1%, w/w). Monosialoganglioside 3 (GM3) and disialoganglioside 3 (GD3) are the major glycolipids of milk and these gangliosides have been reported to contain 0.14 to 1.10 mg/100 mL (Pan and Izumi, 2000). Analysis of polar lipids of whey (Rombaut et al., 2007b), a by-product of mozzarella cheese production, revealed the presence of 40.6% PE, 19.1% PC, and 15.7% SM. In addition, analysis of LacCer content revealed a high level in lipids (8.5%). The content was slightly different from that of WPL. This is likely due to differences in raw milk and differences in the manufacturing process of whey obtained as a by-product.
In general, after induction, oil rapidly increases the oxygen absorption rate and oxidation product. Accordingly, rancidity is caused by various physical and chemical changes in oil. Therefore, when the induction time is long, it can be said that the oxidation stability is excellent (Nam et al., 2007). In order to evaluate WPL oxidation stability, DHA and WPL or ARASCO oil or egg phospholipids were mixed, and oxidation induction time was measured by rancimat test (Fig. 3). The oxidation induction time is the time until the conductivity curve reaches the inflection point. Induction times of DHA alone, DHA-ARSCO oil mixture, and DHA-egg phospholipids oil mixture were 0.66 h, 1.91 h, and 4.20 h, respectively. However, induction time was not measured in DHA and WPL oil mixture.
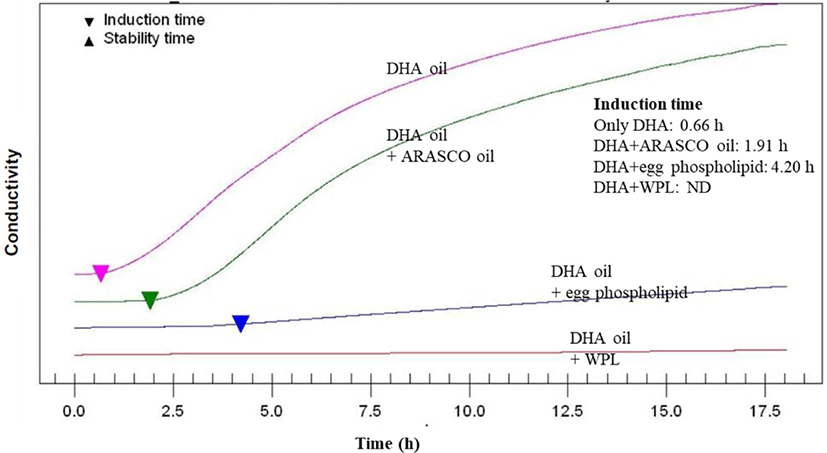
The oil mixture of DHA and WPL has a high induction time due to the effect of WPL addition to DHA. There are many studies on the antioxidant properties of PL (Jung et al., 2001; King et al., 1992; Segawa et al., 1995), and not only PL but especially PE and PC can give excellent oxidation stability. This is because the primary amino group of PE contained in polar lipid reacts with the reactive carbonyl produced during heating to produce pyrolyzed phospholipids and heterocyclic moieties with anti-oxidative properties. In addition, the amino groups of PE and PC can promote hydrogen or electron donation to tocopherols (Ramadan et al., 2003). In the previous study, the sum of PE and PC contents extracted from ethanol from whey protein was 32.4% and 21.1%, respectively, of 80% and 90% ethanol (Price et al., 2018). In this study, the sum of PE and PC contents of polar lipid fraction extracted from WPL was 33.9%. Therefore, it was confirmed that the oxidation stability was excellent due to the high content of PE and PC as well as PL.
The MTT assay was performed to determine the cytotoxicity of WPL in mouse macrophages, RAW 264.7. Treatment with increasing concentrations of WPL did not affect cell viability (Fig. 4). Therefore, the subsequent experiments, performed with 100 μg/mL WPL, did not affect the cell viability. This suggests that the anti-inflammatory effect of WPL is not due to a decrease in cell viability, but due to the inherent activity of WPL. Treatment with 0.1 μg/mL of EV for inducing inflammation in RAW 264.7 cells resulted in increased production of IL-6 and TNF. It was confirmed that inflammation was caused by EV. However, when WPL was treated with macrophages, IL-6 and TNF were hardly produced, indicating that WPL had no inflammatory effect.
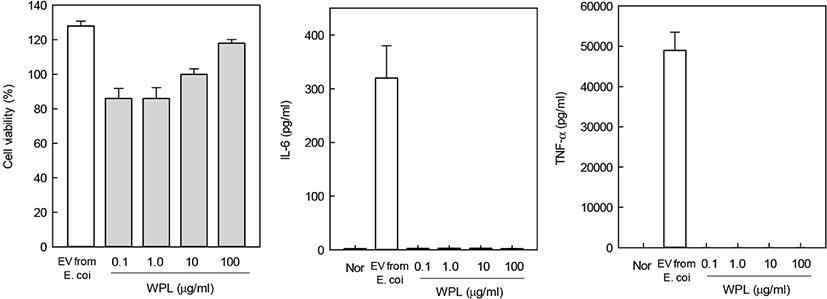
During the inflammatory reactions, nitric oxide, TNF-α, and IL-6 are produced, which play an important role in defense against early infection (Higuchi et al., 1990). Prokaryotic or eukaryotic cells secrete EVs, and the secreted EVs have been reported to have several functions. Extracellular vesicles secreted by gram-negative bacteria contain lipopolysaccharides (LPS) and bacterial proteins. The EVs from gram-negative bacteria are known to induce inflammatory diseases (Lee et al., 2007; Lee et al., 2009).
Inflammatory cytokines play a significant role as indicators of inflammation. Therefore, the effect of WPL on the production of inflammatory cytokines (IL-6 and TNF-α) induced by EVs from E. coli in RAW 264.7 cells was measured (Fig. 5). The EVs produced by L. plantarum, having anti-inflammatory activity, were used as a positive control, which decreased the production of IL-6 and TNF-α. In addition, IL-6 and TNF-α increased upon treatment with EVs from E. coli and decreased along with increasing concentration of WPL. Thus, WPL was confirmed to have anti-inflammatory activity. The effect of EVs from L. plantarum in the prevention or inhibition of inflammation has already been reported in many studies (Kim et al., 2018; Molina-Tijeras et al., 2019).
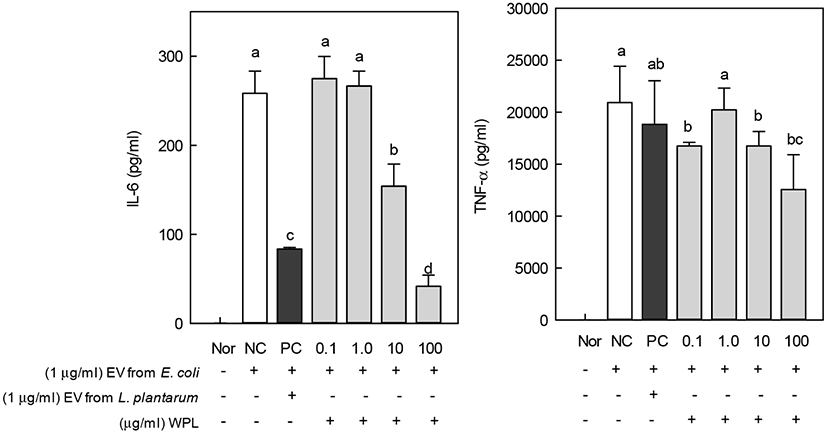
Phosphatidylserine, a type of polar lipid, has been reported to act as an endogenous modulator of immune and anti-inflammatory responses (Gaitonde et al., 2011; Yamazaki et al., 1997). The anti-inflammatory effects of PC and LysoPC on chronic inflammatory ulcerative colitis have also been reported (Toekes et al., 2010). Milk fats containing a large amounts of MFGM have been reported to have an anti-inflammatory effect on LPS-induced inflammation in the gastrointestinal tract (Snow et al., 2011). There are increasing reports on the regulation of inflammatory responses and treatment of inflammatory diseases through PL intake.
Conclusion
In this study, a solvent extraction and fractionation process was developed to concentrate polar lipids from whey and butter processing derived by-products. In addition, it has been shown that the corresponding polar lipids can greatly contribute to enhancing the oxidation stability of the functional polyunsaturated fatty acids, represented by fish oil. It is believed that by investigating the anti-inflammatory effects in macrophages, milk-derived polar lipids can be effectively used for promoting intestinal health and alleviating inflammatory diseases. Milk-derived polar lipids, unlike the commonly distributed plant-derived polar lipids, contain substantial amounts of sphingomyelin, glycosyl-ceramide, and phosphatidylserine. In the future, diverse innovations would be required, such as 1) the development of an active material for topical product through conversion of sphingomyelin and 2) the development of a cognitive functional product using sphingomyelin and phosphatidylserine.