Introduction
Lactobacillus plantarum is a member of human intestinal microorganisms; it can prevent and treat gastrointestinal disorders such as irritable bowel syndrome, ulcerative colitis, and diarrheal diseases (Adler and Lee, 2010). L. plantarum has been widely used as the starter to improve the flavor and texture of fermented foods (de Vries et al., 2006). Turchi et al. (2017) used L. plantarum and Streptococcus thermophiles as the starter to ferment donkey milk, and they found L. plantarum had good fermentation potential and also could promote the growth of S. thermophiles. In recent years, many researchers have found that L. plantarum is also used as the starter in fruits and meat (Endo et al., 2018; Luxananil et al., 2009; Palomino et al., 2015).
During the preparation of the starter, L. plantarum is usually dried into powder by freeze drying, whereas freeze drying spends lots of time and energy (Anandharamakrishnan et al., 2010). Compared with freeze drying, spray drying takes less time, consumes less energy, costs less, and more suitable for industry production (Palomino et al., 2015). During spray-drying process of starter, high temperature and dry dehydration will reduce the viability of probiotics. The high temperature will destroy the structure of proteins, nucleic acids, and other macromolecules, and breaks the connection between monomer units and eventually leads to the destruction of monomer units (Corcoran et al., 2008); Dry dehydration can change the fluidity and physical state of the cell plasma membrane, resulting in reduction of metabolic activity (Crowe et al., 1992; Garre et al., 2010; Leslie et al., 1995; Lievense and van 't Riet, 1994; Teixeira et al., 2010). Sodium alginate was used for embedding L. acidophilus NCIMB 701748 by spray drying, the viable counts was only 8.40 Log CFU/g (Yonekura et al., 2014). But, the viable counts of L. acidophilus was 10.60 Log CFU/g after freeze drying (Qin et al., 2013).
The adverse environmental conditions such as acid, heat, pressure, and oxygen will lead to a significant decrease in probiotic viability and limit the use of probiotics (Coghetto et al., 2016). The protective agent plays a vital role in protecting probiotics against adverse conditions and storing for a long time after drying (Agudelo et al., 2017). Whey protein (WP) is a by-product in the cheese production process, which accounts for 20% of total milk protein, and it has excellent gelling and film-forming properties (Perez-gago and Krochta, 2010). Pullulan is a neutral water-soluble extracellular polysaccharide that is produced by microbial and has lots of excellent properties such as heat resistance, solubility, non-hygroscopicity, and film-forming property (Singh et al., 2009). The L. plantarum was embedded by whey protein concentrate (WPC) and pullulan, and the viable counts was 7.24 Log CFU/g after spray drying (Zhang et al., 2019).
In this study, we used WPC, pullulan, trehalose, and sodium glutamate as the protective agent to improve the viable counts of L. plantarum after spray drying and optimized the proportion of protective agent and the spray-drying parameters. Besides, the optimal storage temperature of the starter was evaluated during storage.
Materials and Methods
L. plantarum (LP1406) was provided by the Jilin province Innovation Center for Food Biological Manufacture, Jilin Agriculture University. WPC (80%) was obtained from Fonterra Co., Ltd. (Auckland, New Zealand). Pullulan (99%) was purchased from Hayashibara Co., Ltd. (Tokyo, Japan). Trehalose was supplied by Baiwei Biotechnology Co., Ltd. (Hebei, China). Sodium glutamate was from Plum Blossom Essence Group Co., Ltd. (Hebei, China). MRS Broth was from Hope Bio-Technology Co., Ltd. (Shandong, China). Agar was from Biofroxx Bio-Technology Co., Ltd. (Guangdong, China).
L. plantarum was reactivated three times before use. The strain was incubated into the sterile MRS broth at 37°C for 18 h. The cells were harvested by centrifuging at 2,600×g for 10 min, 4°C and washed twice with sterile 0.85% (w/v) sodium chloride solution. The suspension, which contained approximately 1010 CFU/mL was obtained by resuspending bacterial sludge in the saline solution and stored at 4°C.
WPC, pullulan, trehalose, and sodium glutamate were dissolved with distilled water to prepare the protective agent solution, and the proportion was optimized. The solution was adjusted to pH 8.5 and then heated at 80°C for 30 min. The protective agent was cooled down to 25°C and mixed with the L. plantarum suspension. The mixed solution was spray dried by the spray dryer (SD-1500, Triowin, Shanghai, China). The ratio of bacteria to protective agents, inlet air temperature and feed flow rate were optimized. Finally, the dried L. plantarum starter was collected.
The single-factor test was performed mainly to assess the effect of WPC, pullulan, trehalose, and sodium glutamate on viable counts of L. plantarum and identify their suitable ranges for response surface method (RSM). During this process, the ratio of bacteria to protective agents was 1:1 (v:v), the feed flow rate was 300 mL/h, and the inlet air temperature was 120°C.
The optimal ranges of WPC (A), pullulan (B), trehalose (C), and sodium glutamate (D) were established based on the single-factor test. A Box-Behnken design based on the four main factors with three levels was used for exploring the optimal proportion of the protective agent and the interactions of these variables. The entire design includes 29 experiments with three replicates. The factors and levels were shown in Table 1. The fitness is evaluated by the quadratic polynomial equation, and the correlation coefficient is calculated by the following formula:
Levels | WPC (g/L) | Pullulan (g/L) | Trehalose (g/L) | Sodium glutamate (g/L) |
---|---|---|---|---|
A | B | C | D | |
−1 | 20 | 10 | 5 | 30 |
0 | 30 | 20 | 51 | 40 |
1 | 40 | 30 | 25 | 50 |
Where, Y, α0, αi, αii, αij are the predicted response, constant coefficient, linear coefficient, quadratic coefficient and cross-product coefficient, respectively. X are independent variables.
Experiment design, graphs construction and results analysis were carried out by Design of Expert (DOE Version 8.5, Stat-Ease. Inc, Minneapolis, MN. USA). To calculate the regression equation, analyze the contour graph and 3D surface plots, the optimal value of each variable was obtained.
The single-factor test was performed mainly to assess the effect of the ratio of bacteria to protective agents, the feed flow rate, and the inlet air temperature on viable counts of L. plantarum, and to identify their suitable ranges for orthogonal test. The protective agent was composed of 24.6 g/L WPC, 18.8 g/L pullulan, 16.7 g/L trehalose, and 39.3 g/L sodium glutamate during this process.
Based on the single-factor test, the optimal ranges of the ratio of bacteria to protective agents (A), feed flow rate (B), and temperature (C) were established. An orthogonal test of four factors and three levels was formed to optimize the spray-drying parameters. Table 2 shows the orthogonal test L9(3)4 parameters. Each treatment was performed in triplicates.
Levels | Ratio of bacteria to protective agents (v:v) | Feed flow rate (mL/h) | Temperature (°C) |
---|---|---|---|
A | B | C | |
1 | 2:1 | 210 | 110 |
2 | 3:1 | 240 | 115 |
3 | 4:1 | 270 | 120 |
Viable counts of L. plantarum before and after spray drying were determined by plate colony counting method in triplicates. 1.0 g L. plantarum starter was dissolved in 9 mL trisodium citrate solution (3%, w/v), the suspension was diluted ten times continuously with 0.85% saline solution and plated on MRS agar. The plates were incubated at 37°C for 48 h before counting.
The moisture content of L. plantarum starter was measured immediately after spray drying by oven drying. 0.5 g powders were dried at 105±5°C to constant weight. The initial and final weight was used to calculate the moisture content. The equation was as follow:
Where W0 is the weight of L. plantarum starter before oven drying and W1 is the weight of L. plantarum starter after oven drying.
The samples were placed on a conductive adhesive attached to a circular aluminum specimen stub and then coated vertically with gold under vacuum. The surface morphology of samples was characterized by scanning electron microscope (SEM) (Hitachi S-4200-Tokyo, Japan) at an accelerating voltage of 5 kV.
Samples of L. plantarum starter were stored at −20°C, 4°C, and 25°C for 360 days. The viable counts of L. plantarum was determined as described in the viable counts of L. plantarum in starter at 0, 3, 7, 15, 30, 60, 90, 120, 180, 240, 300, and 360 d.
All the experiments were conducted in triplicate and the results were expressed as Mean±SD. ANOVA and Tukey's multiple comparison test were applied to analyze statistical significance using SPSS 16.0 (SPSS Inc., Chicago, IL, USA). When significant p<0.05, statistical difference was assumed.
Results and Discussion
The single-factor test showed that the appropriate concentrations of WPC, pullulan, trehalose, sodium glutamate were 20, 30, and 40 g/L; 10, 20, and 30 g/L; 5, 15, and 25 g/L; and 30, 40, and 50 g/L, respectively (Fig. S1). Based on single-factor test results, RSM was carried out.
The proportion of protective agent was analyzed by the Box-Behnken design. In this study, different combinations of WPC, pullulan, trehalose, and sodium glutamate were done 29 times and each experiment conducted in triplicate. The data of Table S1 in the supplementary material were analyzed by multivariate regression analysia, and the results were shown in Table 3. The final obtained regression equation based on the experimental factors by software analysis was as follows:
Where Y is the viable counts of L. plantarum, A, B, C, and D are the concentration of WPC (%), pullulan (%), trehalose (%), and sodium glutamate (%), respectively.
The F-value of the model was 43.58 and the p-value was less than 0.0001, indicating that the model was stable and statistically significant. In the same way, A, B, C, D, AB, AC, AD, BC, BD, CD, A2, B2, C2, and D2 were reported. The correction coefficient r2, which was calculated to be 0.9733, explained the variability of the response values through the experimental parameters and their interactions, and it implied the model could explain 97.33% of the variation. The adjustment coefficient r2 obtained in this experiment was determined to be 0.9551, and the difference from the correlation coefficient r2 was logically acceptable. Therefore, this model was suitable to analyze and predict the optimum proportion of the protective agent.
WP, including WPC and WPI, has high nutritional value, excellent gel properties and biocompatibility. Heating caused WP to aggregate and form a spatial network structure (Mulvihill and Kinsella, 1998). The WP gel had excellent pH sensitivity, controllable permeability, and high gel strength, thereby improving the viability of probiotics during spray drying (Chen and Subirade, 2006; Hebrard et al., 2006). The survival rate of L. plantarum A17 increased from 37.7% to 91.7% along with WPI concentration increased from 0.6% to 20% (Khem et al., 2016). Many studies showed that the synergistic effect of WPC and other compounds could better protect the viability of L. plantarum during spray drying (Lu et al., 2016; Rajam and Anandharamakrishnan, 2015).
In our study, we used WPC, pullulan, trehalose, and sodium glutamate as the spray-drying protective agent for L. plantarum and optimized their proportion by RSM (Fig. 1). The curved properties of respond plots showed the interaction between variables and responses (Betiku and Adesina, 2013). The contour plots were put into supplementary materials (Fig. S2). The elliptical shape in Fig. 1a showed the significant effect of WPC and pullulan on improving L. plantarum cell viability. At low concentrations, the viable counts increased with the addition of WPC and pullulan; when the concentration of WPC and pullulan was 30 g/L and 20 g/L, respectively, the viable counts of L. plantarum reached the maximum; then the concentration of WPC and pullulan continued to increase, the viable counts decreased. The result coincides well with the research conducted by Cabuk and Tellioglu Harsa (2015) which evaluated the protective effect of WPI/pullulan microcapsule on the viability of L. acidophilus NRRL-B 4495 under vitro gastrointestinal conditions. Fig. 1b showed significant interaction between WPC and trehalose. The viable counts of L. plantarum increased and reached the maximum and approached the central point at 30 g/L WPC and 15 g/L trehalose, and then decreased with the increase of WPC and trehalose. The elliptical shapes in Fig. 1c showed a significant interaction between WPC and sodium glutamate in response values. As the concentration of WPC and sodium glutamate increased, the viable counts of L. plantarum increased; the protective effect tended to decline after WPC and sodium glutamate reached the optimal concentration 30 g/L and 40 g/L, respectively. It was also found that the interaction between pullulan and sodium glutamate promoted the model (Fig. 1d). The elliptic curve indicated the promotive effect of pullulan and sodium glutamate on the cell viability of L. plantarum, but when the concentration of pullulan and sodium glutamate were more than 20 g/L and 40 g/L respectively, viable counts declined. The interactions between pullulan and trehalose, trehalose and sodium glutamate were shown in Fig. 1e, f, respectively. Although the viable counts couldn't be enhanced significantly, the synergistic protection of pullulan and trehalose still promoted the viability of L. plantarum in spray-drying process to a certain extent.
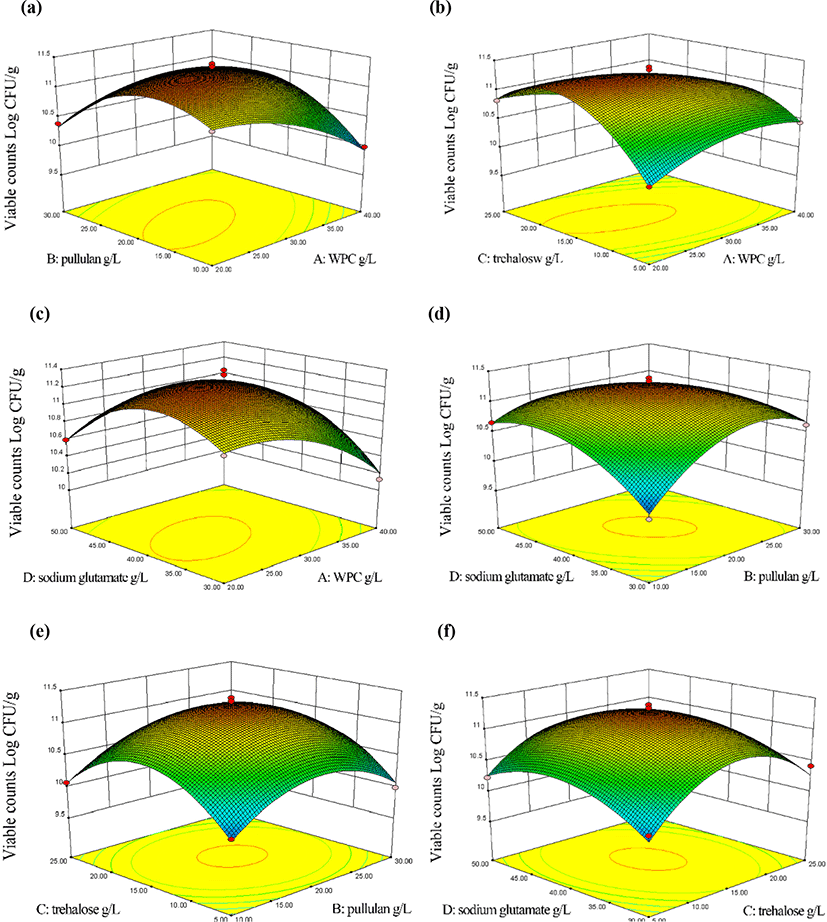
Under certain conditions, WP and polysaccharides aggregated to form regular network structures for protecting biologically active substances (Coughlan et al., 2010). As a kind of non-reductive stable polysaccharide, pullulan with strong oxygen resistance has high mechanical strength and low permeability compared with other saccharides (Yuen, 1974). The use of WP and pullulan gel for preparing lactic acid bacteria microcapsules has been reported (Zhang et al., 2019). Trehalose could be used as an excipient in the drying process to stabilize protein structure because of its stable efficacy and excellent processing properties (Mensink et al., 2017). And trehalose had a high glass transition temperature which could limit molecular interactions by forming glass structures (Patist and Zoerb, 2005). Besides, trehalose as a prebiotic could improve the survival rate of probiotics by improving their adaptability to adverse environmental conditions (Darjani et al., 2016). Studies showed that trehalose was an excellent spray-drying protective agent (Adler and Lee, 2010; Lipiainen et al., 2018). During the spray-drying process, the loss of water would lead to a significant reduction of the viable counts of L. plantarum. The close action of sodium glutamate and water made the dry powder remain an appropriate amount of water, meeting the minimum requirements of microorganisms (Morichi et al., 2006). Besides, studies showed that sodium glutamate also had an antioxidant effect, inhibiting the oxidation of triacylglycerol and the formation of free radicals, to prevent irreversible damage to the cell membrane (Morichi et al., 2006; Polomska et al., 2012).
In the process of optimization, the excessive addition of WPC, pullulan, trehalose, and sodium glutamate in the protective agent increased the osmotic pressure, the cells dehydrated, and cell membrane was damaged (Wood, 2015). On the other hand, the viscosity of the mixed system increased as the concentration of pullulan and trehalose increased. After a certain concentration, the release of cell was affected by high system viscosity after rehydration. Therefore, the viable counts of L. plantarum decreased.
According to RSM, WPC, pullulan, trehalose, and sodium glutamate of 24.6 g/L, 18.8 g/L, 16.7 g/L, and 39.3 g/L respectively were obtained as the most desirable concentration for the optimum spray-drying protective agent. Under these predicted conditions, the viable counts of L. plantarum was 11.29 Log CFU/g. In order to verify the optimized process parameters, three validation experiments were carried out based on the suggested model, and the viable counts of L. plantarum was 11.25 Log CFU/g. The experimental values, mean of three trials were found to be in close agreement with the predicted values, and the difference of them was within the acceptable limits. A reasonable model was selected. Romano et al. (2018) used crystalline inulin and amorphous inulin to coat L. plantarum, the viable counts of L. plantarum after spray drying were 9.74±0.20 and 9.93±1.61 Log CFU/g. Whey, permeate and whey retentate were used to protect L. plantarum ATCC 8014, and the viable counts after spray drying were 10.26±0.06, 9.98±0.01, 10.00±0.08 Log CFU/g, respectively (Eckert et al., 2017). Higher viable counts in this paper indicated that L. plantarum was effectively protected by WPC, pullulan, trehalose, and sodium glutamate as the protective agent.
The single-factor test showed that the appropriate ratios of bacteria to protective agents, feed flow rates, and inlet air temperatures were 2:1, 3:1 and 4:1 (v:v); 210, 240, and 270 mL/h; 110°C, 115°C, and 120°C, respectively (Fig. S3). Based on single-factor test results, orthogonal test was carried out.
The orthogonal test was used to optimize the spray-drying parameters. Three factors were evaluated by an orthogonal L9(3)4 test design. The results (Table 4) showed that the influence level of these factors on the viable counts of L. plantarum in order was as follows: temperature (C)>ratio of bacteria to protective agents (A)>feed flow rate (B). The optimal spray-drying parameters for the viable counts of L. plantarum were the ratio of bacteria to protective agents 3:1 (v:v), the feed flow rate 240 mL/h, the inlet air temperature 115°C (A2B2C2). The viable counts of L. plantarum was 12.22±0.27 Log CFU/g (survival rate 85.12%), and the water content was 4.7%. Gum arabic, maltodextrin, and gelatin were used to protect L. reuteri LR92 by spray drying, and the viable counts were 8.40, 7.96, 8.73 Log CFU/g (Guergoletto et al., 2017). L. acidophilus La-5 was spray-dried with trehalose as a protective agent, and the viable counts was 11.04±0.07 Log CFU/g (Nunes et al., 2018). When used maltodextrin and trehalose as the protective agent, the survival rate of L. plantarum was 57.70% after spray drying (Lapsiri et al., 2012). The proportion of protective agent and spray-drying process parameters used in this study could protect L. plantarum better.
The scanning electron micrographs of L. plantarum starter were observed (Fig. 2). The powder particles were spherical or ellipsoidal and varied sizes between 1–10 μm. The L. plantarum was wrapped by the protective agent. And the surface on the particles had some concavities, which were typical features of spray-drying products, the same as Fritzen-Freire et al. (2012) observed. In addition, the surface of particles appeared visible cracks. These cracks might facilitate the escape of heat from the interior of particles after spray drying, causing less heat injury to the embedded microorganisms. Thus, this might be one of the reasons that contributed to the higher survival of L. plantarum with the protective agent comprising WPC, pullulan, trehalose, and sodium glutamate after spray drying (Ranadheera et al., 2015).
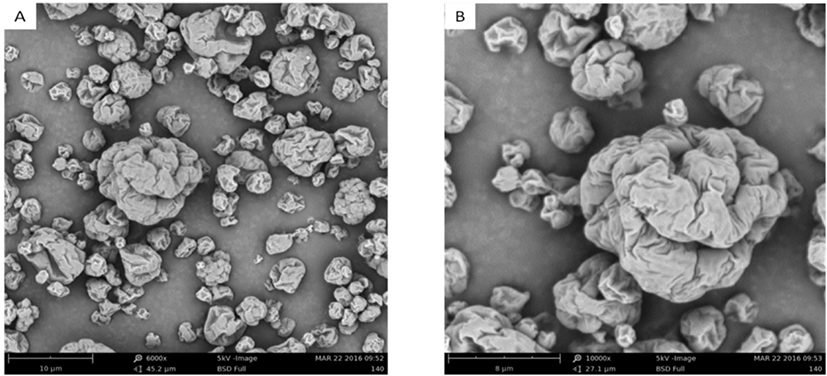
The storage period of probiotics starter is mainly determined by the storage temperature (Holkem et al., 2017). At low temperatures, probiotics are prone to keep active (Etchepare et al., 2016). In this study, during storage of L. plantarum starter, the viable counts of L. plantarum starter decreased at −20°C, 4°C, and 25°C (Fig. 3). There were significant differences between different storage temperatures. There was the most considerable damage on the viability of L. plantarum in starter at 25°C and L. plantarum completely lost its viability at 25°C after 90 days. After storing at −20°C and 4°C for 200 d, the viable counts of L. plantarum were 11.29±0.29 and 10.74±0.36 Log CFU/g, −20°C was considered as the optimum temperature to store L. plantarum starter. Relative low temperature limited probiotics kinetic energy, resulting in lower molecular motion (Bei et al., 1989). Besides, slower bacterial metabolism produced less waste, and chemical reactions that were harmful to bacteria were also limited (Chen et al., 2017). A similar study used trehalose as the protective agent, the viable counts of the L. acidophilus was 8.31±0.08 Log CFU/g after storing for 120 days at −18°C (Nunes et al., 2018). In this study, under low-temperature storage conditions, the protective agent composed of WPC, pullulan, trehalose, and sodium glutamate could protect L. plantarum better.
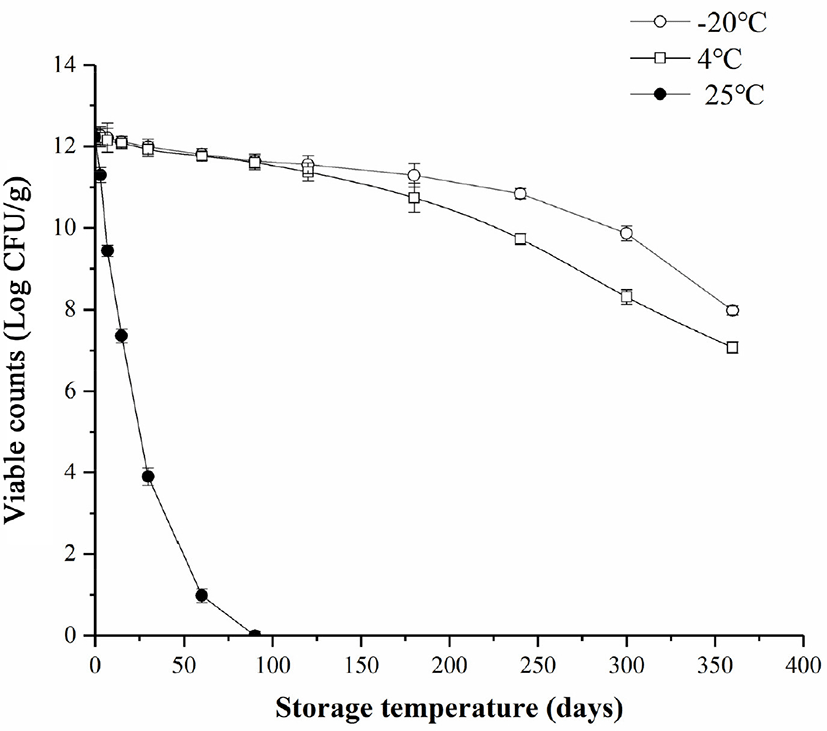
Conclusion
The optimal proportion of protective agent for L. plantarum starter was 24.6 g/L WPC, 18.8 g/L pullulan, 16.7 g/L trehalose, and 39.3 g/L sodium glutamate. The optimal spray-drying parameters for L. plantarum starter were ratio of bacteria to protective agents 3:1 (v:v), feed flow rate 240 mL/h, the inlet air temperature 115°C with the max viable counts of L. plantarum (12.22±0.27 Log CFU/g), and the survival rate was 85.12%. The viable counts of L. plantarum in starter was 11.29±0.29 Log CFU/g when L. plantarum starter stored at −20°C for 200 days. This study provides a new protective agent with a great protective effect on L. plantarum after spray drying and storage. This protective agent has great significance for protecting L. plantarum in production.