Introduction
Nanotechnology is applied not only for medication and health, but also for cosmetics, food science, and packaging (Cao et al., 2016; Kango et al., 2013). Nano-delivery systems (NDSs), submicron-sized carriers with size about 1 to 200 nm, have been manufactured to carry bioactives by entrapping and protecting them from harsh environmental conditions, such as acidic gastric conditions during digestion and high heat treatment during food processing (Ha et al., 2013; Ha et al., 2017; Mohanraj and Chen, 2006; Ron et al., 2010; Zhang et al., 2010). The submicron size and surface charge of NDSs have a significant effect on bioavailability and stability of bioactives, which affects the intestinal absorption of NDSs containing bioactives due to their key roles in the interactions with intestial epithelial cells (Chen et al., 2006; Ha et al., 2015; Zhang et al., 2010). Recently, there have been various studies of NDSs entrapped with hydrophobic bioactives including quercetin, coenzyme Q10, and tea polyphenol to increase their bioavailability (Ha et al., 2013; Ha et al., 2018a; Lee et al., 2013; Liu et al., 2016).
Goat milk has been widely used to produce various milk products (Amigo and Fontecha, 2011; Lopez-Aliaga et al., 2010). Compared with cow milk, goat milk has lower allergenicity and higher digestibility due to differences in genetic types of amino acids, which lead to the changes in the digestibility (Haenlein, 2004; Park et al., 2007; Yangilar, 2013; Zhao et al., 2014). In addition, goat milk contains higher total protein and casein content than cow milk (Amigo and Fontecha, 2011). β-casein is present most abundantly (i.e., 0%–53.0%) in total casein content of goat milk (Amigo and Fontecha, 2011). Specifically, A2 β-casein is present most abundantly in the generic variants of goat milk β-casein (Amigo and Fontecha, 2011). The digestion of A1 β-casein results in the release of β-casomorphin-7, an opoids peptides that may be related to various human disease, such as type 1 diabetes, autism, schizophrenia, and heart diseases (Brooke-Taylor et al., 2017; Priyadarshini et al., 2018). However, β-casomorphin-7 is not be observed during A2 β-casein digestion due to the presence of proline (amino acid position 67) that can prevent the formation of β-casomorphin-7 (Brooke-Taylor et al., 2017; Priyadarshini et al., 2018). Therefore, it is neccessary to prepare NDSs with A2 β-casein for customers who have major health concerns related with β-casomorphin-7, which can be resulted from the digestion of A1 β-casein.
Chitosan is a natural cationic biopolymer and it is obtained by deacetylation of chitin (George and Abraham, 2006; Zhang et al., 2010). The chitosan has known to possess mucoadhesive property in vitro, which can be advantageous for the delivery of target bioactives to the gastrointestinal tract (George and Abraham, 2006). In this study, chitosan oligosaccharide (CSO) was selected as a delivery material of NDSs because CSO contains low molecular weight, low viscosity, and free amino group (i.e. D-glucosamine), and high solubility in water (Hamed et al., 2016; Zou et al., 2016). In addition, CSO has a non-toxicity, biodegradability, biocompatibility, and an ability to enhance the intestinal permeability of various bioactives, which make it an excellent delivery material (Baldrick, 2010; Bowman and Leong, 2006; Du et al., 2009; Ha et al., 2013; Ha et al., 2018b; Yuan et al., 2010).
Resveratrol is a polyphenolic compound and resveratrol belongs to stilbene class, which has health advantages including anti-oxidant, anti-cancer, and anti-inflammatory (Pangeni et al., 2014; Wenzel and Somoza, 2005). But, the food application of resveratrol may be problematic because its poor aqueous solubility, stability, and bioavailability (Ha et al., 2016; Pangeni et al., 2014; Summerlin et al., 2015; Wenzel and Somoza, 2005). Although many studies were conducted to reduce the limitation of resveratrol application to food using delivery systems (Bu et al., 2013; Gokce et al., 2012; Jeong et al., 2016; Sanna et al., 2012; Zu et al., 2014), there were some limitations; 1) Low bioavailability as microparticle of > 1,000 nm (Champagne and Fustier, 2007; Desai et al., 1996; Desai et al., 2010), 2) Destruction of bioactive materials through high-temperature treatment of >100°C (Ahmed et al., 2010; Estevinho et al., 2013; Kumar et al., 2004), 3) Toxic cross-linking agents, such as glutaraldehyde and synthetic polymers (Leung, 2001; Li et al., 2008). Therefore, it is neccessary to produce effective delivery systems for resveratrol with submicron size (< 10,000 nm), which are manufactured with low-temperature treatments and without using toxic cross-linking agents. Since an increase in the CSO concentration level and manufacturing temperature from 5°C to 35°C can enhance barrier effects against the diffusion of resveratrol and increase hydrophobic associations between A2 β-casein and resveratrol, respectively, it was hypothesized that CSO concentration levels and low-temperature treatment could play a key role in determining the physicochemical characteristics of CSO/A2 β-casein NDSs, such as particle size, size distribution, surface charges, and entrapment efficiency of resveratrol.
The objectives of this study were to form NDSs using food-grade delivery materials, CSO and A2 β-casein, and to study how CSO concentration level and low-temperature treatment affected the production and physicochemical characteristics of CSO/A2 β-casein NDSs.
Materials and Methods
CSO with molecular weight ~20 kDa was supplied by Amicogen Co. (Jinju, South Korea). A2 β-casein was provided by Sahmyook University. Resveratrol (3,4’,5-trihydroxy-trans-stilbene) and sodium tripolyphosphate (TPP) was obtained from Sigma-Aldrich (St. Louis, MO, USA). All other reagents were acquired from Sigma-Aldrich (St. Louis, MO, USA).
CSO/A2 β-casein nanoparticles were produced using modified ionic-gelation methods with TPP according to Ha et al. (2013) and Ha et al. (2018b). CSO solutions with various concentration levels starting from 0.2% to 0.6% (w/w) and 0.2% (w/w) of A2 β-casein solutions were made in distilled water. CSO and A2 β-casein solutions were mixed followed by stirring for 5 min at room temperature. CSO/A2 β-casein mixture solutions were adjusted to pH 3.5 by using 0.1 M HCl and then treated at 5°C, 20°C, or 35°C for 30 min followed by the addition of 0.1 mM TPP to prepare CSO/A2 β-casein nanoparticles. For the production of resveratrol-loaded CSO/A2 β-casein NDS, 100 μL of resveratrol solution in ethanol (5 mg/mL) was added to 9.9 mL of CSO/A2 β-casein nanoparticles. CSO/A2 β-casein mixtures were adjusted to pH 3.5 with 0.1 M HCl and incubated at 5°C, 20°C, or 35°C for 30 min followed by treatment with 0.1 mM of TPP. The manufacturing process of CSO/A2 β-casein NDSs was illustrated in Fig. 1. NDS suspensions were kept –80°C overnight and freeze-dried by the use of a laboratory-scale freeze-dryer (FD-1000, Sunileyela, Japan).
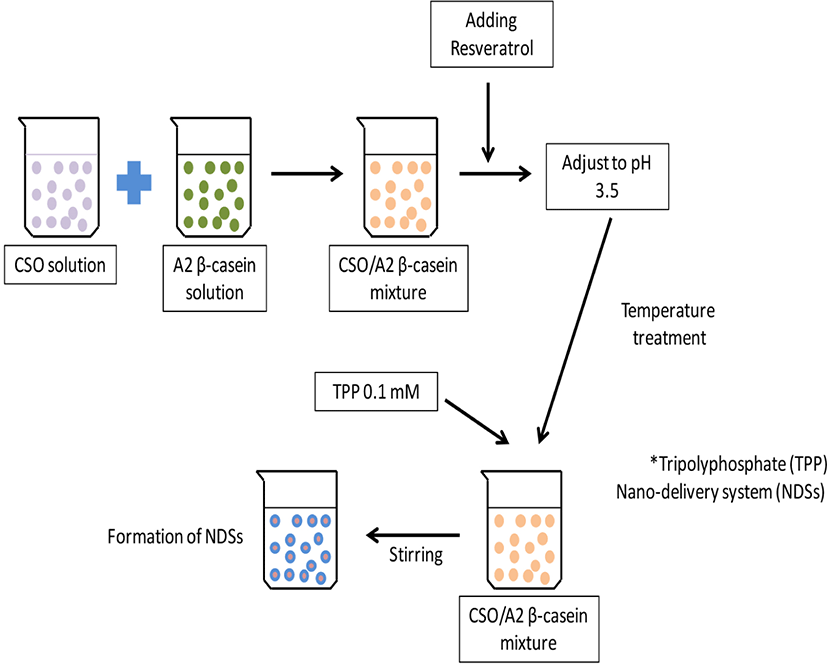
The structural properties of CSO/A2 β-casein NDSs were investigated by using transmission electron microscopy at 120 kV (EM, FEI Tecnai 12, Philips, Eindhoven, Netherlands). Twenty microliters of 10-fold diluted CSO/A2 β-casein suspension was deposited on a 200 mesh copper grid coated with carbon followed by staining with 2% phosphotungstic acid.
The physicochemical characterisitcs of CSO/A2 β-casein NDSs, such as particle size, polydispersity index (PDI), and zeta-potential value, were assessed using Zetasizer Nano ZS (Malvern Instruments, Malvern, UK). CSO/A2 β-casein NDSs were 20-fold diluted with distilled water at room temperature. The scattering angle of Zetasizer Nano ZS measurement was 173° and a laser beam at 633 nm. The Smoluchowski equation was performed to get zeta potential values from the electrophoretic mobility (velocity of a particle in an electric field, μm/s) of CSO/A2 β-casein NDSs.
EE of resveratrol in CSO/A2 β-casein NDSs was investigated using HPLC as described by Ha et al. (2013). Final concentration of added resveratrol in CSO/A2 β-casein NDSs was 0.05 mg/mL. To separate unentrapped resveratrol, CSO/A2 β-casein NDSs containing resveratrol were centrifuged at 10,000×g at 20°C for 30 min. To assess the amount of unentrapped resveratrol in supernatant, 25 μL of resveratrol supernatant was injected into the HPLC system (Agilent 1260 Series, USA). A reverse phase C18 column (3.5 μm, 4.6×150 mm, Waters Company, USA) at a flow rate of 1.0 mL/min was used to analyze unentrapped resveratrol. The mobile phase was the mixture of MeOH and water (52:48, v/v) adjusted with 0.05% acetic acid. The detection wavelength was set at 303 nm. The calibration curve was acquired using standard resveratrol (0.01 to 0.05 mg/mL) with a correlation coefficient of 0.999. The standard resveratrol was used to estimate the amount of entrapped resveratrol through the amount of resveratrol that was not obtained in the supernatant. The entrapment efficiency of resveratrol was computed by the next equation.
The physical stability of CSO/A2 β-casein NDSs during heat treatment and storage in model milk and yogurt were evaluated by measuring the size and polydispersity index. Freeze-dried CSO/A2 β-casein NDSs were reconstituted in deinoized water at the concentration of 1 mg/mL and heated at 90°C for 30 min or 125°C for 10 min followed by cooling to room temperature in iced water. CSO/A2 β-casein NDSs were also reconstituted in model yogurt (deionized water adjusted to pH 4.8) and milk (deionized water adjusted to pH 7.0) at concentration of 1 mg/mL and stored at 4°C for 14 days.
All data were demonstrated as mean±SD from three replicates. One-way analysis of variance (ANOVA) with Tukey’s honest significant difference (HSD) test was applied to measure the significant impacts of CSO concentration level and low-temperature treatment on physicochemical characteristics of CSO/A2 β-casein NDSs and entrapment efficiency. Statistical analysis was accomplished with SAS software package (Version 9.4, SAS Institute Inc., USA). The level of significance was set at 5% level (p<0.05).
Results and Discussion
The production and morphological characteristics of CSO/A2 β-casein NDSs were determined using TEM (Fig. 2). In TEM illustrations, round-shaped particles with a size ranging from 150 to 250 nm were homogeneously dispersed indicating that CSO/A2 β-casein NDSs were successfully manufactured. Moreover, it seems that the size of CSO/A2 β-casein NDSs were increased with an increase in CSO concentration level (Fig. 2).
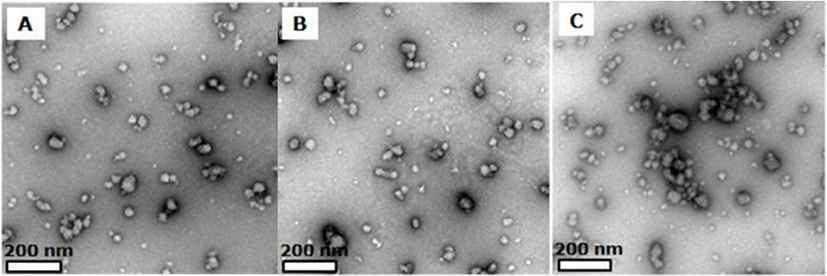
Effects of CSO concentration level on the physicochemical characteristics of CSO/A2 β-casein NDSs, such as size, polydispersity index, and zeta potential, were exhibited in Fig. 3. The size of CSO/A2 β-casein NDSs was significantly (p<0.05) enhanced from 162 to 246 nm as CSO concentration level was increased from 0.1% to 0.3% (w/v) (Fig. 3A). An increase in CSO concentration level may lead to an increase in the intermolecular associations between positively charged CSO and A2 β-casein through ionic interactions with a negatively charged cross-linking agent, TPP, which may result in the formation of bigger CSO/A2 β-casein NDSs. Similar results were reported that an increase in the chitosan concentration level from 0.05% to 0.15% (w/w) resulted in an increase in the size of chitosan/sodium caseinate nanoparticles (Anal et al., 2008). Polydispersity index (PDI) values demonstrate the size distribution of NDSs. As the PDI value is close to 0, it implies that nanoparticles are assumed to be homogeneously distributed (mono-dispersed) (Jeon et al., 2016). In all CSO concentration levels, NDSs had PDI values below 0.3 with no significant differences implying that CSO/A2 β-casein NDSs had homogeneous size distributions (Fig. 3B).
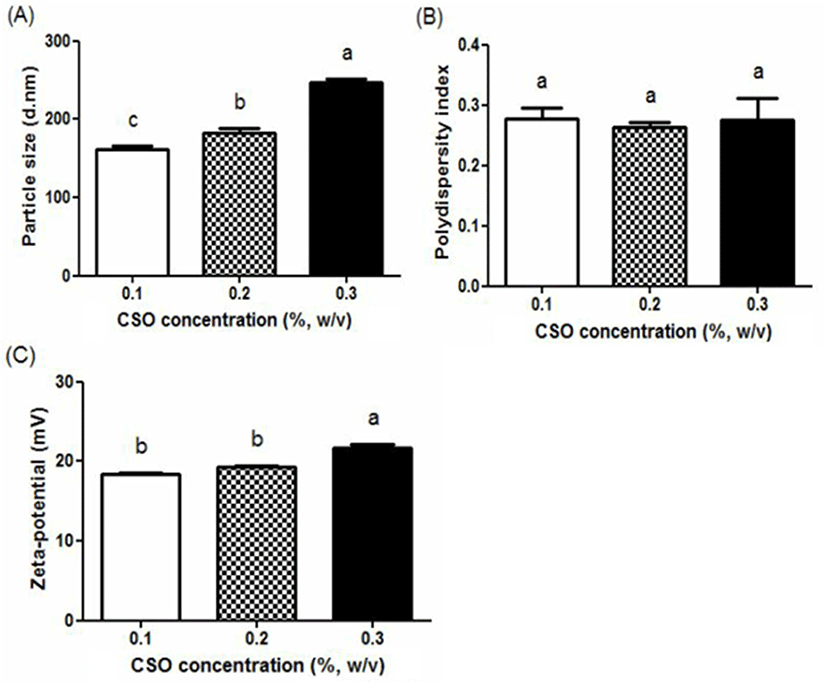
Zeta-potential value is an indicator of surface charges of NDSs and can be used as an important parameter to predict the potential stability of NDSs (Zhang et al., 2008). The zeta-potential values of CSO/A2 β-casein NDSs were significantly (p<0.05) increased from +18 to +21 mV as CSO concentration levels were increased from 0.1% to 0.3% (w/v) (Fig. 3C). It indicates that NDSs with positive surface charges were formed and had good colloidal stability. Since pKa of chitosan was known to be about 6.3 (Bhattarai et al., 2010), CSO molecules are positively charged at pH 3.5, where NDSs were produced. An increase in CSO concentration level may lead to an increase in the association of positively charged CSO molecules to NDSs, which could increase in the number of positive surface charges of CSO/A2 β-casein NDSs. Therefore, CSO/A2 β-casein NDSs manufactured with higher CSO concentration level had more positive surface charges. Similar results were reported by Anal et al. (2008), who found that an increase in the zeta-potential value of chitosan/sodium caseinate NDSs was noticed with an increase in chitosan concentration level from 0.01 to 0.10% (w/w).
The morphological characteristics of CSO/A2 β-casein NDSs produced with various manufacturing temperatures from 5°C to 35°C were presented in Fig. 4. In TEM micrographs, spherically-shaped CSO/A2 β-casein NDSs with a size about 100 to 250 nm were dispersed homogeneously. When manufacturing temperature was increased from 5°C to 35°C, the size of NDS was increased. In addition, more aggregations of NDSs were observed visually as the manufacturing temperature was increased from 5°C to 35°C.
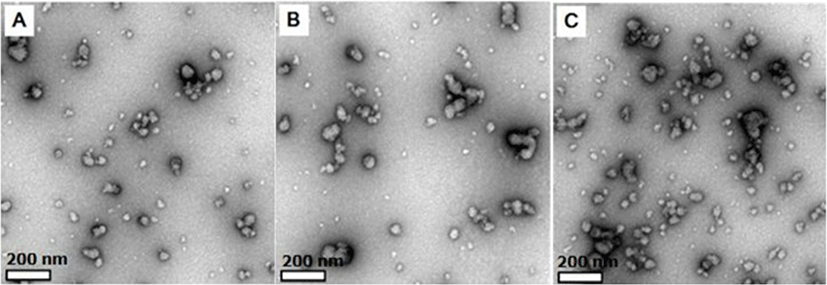
Effects of low-temperature treatment on the size of CSO/A2 β-casein NDSs were presented in Fig. 5A. A significant (p<0.05) increase in the size of CSO/A2 β-casein NDSs from 126 to 257 nm were observed as manufacturing temperature was increased from 5°C to 35°C (Fig 5A), which exhibited similar trend in TEM images (Fig. 4). It was reported that the hydrophobicity of β-casein was significantly (p<0.05) enhanced with an increase in temperature from 0°C to 40°C (Horne, 1998). Threfore, there could be an increase in the hydrophobicity of A2 β-casein with an increase in the manufacturing temperature from 5°C to 35°C. It could contribute to an increase in the intermolecular hydrophobic attractions between A2 β-casein moleules, which may lead to the production of bigger NDSs at higher manufacturing temperature. Moreover, it was reported that the size of β-casein aggregates was significantly affected by temperature (Faizullin et al., 2013). Bachar et al. (2012) reported that the diameter of micelles increased from 25 to 40 nm as the temperature of β-casein increased from 10°C to 60°C.
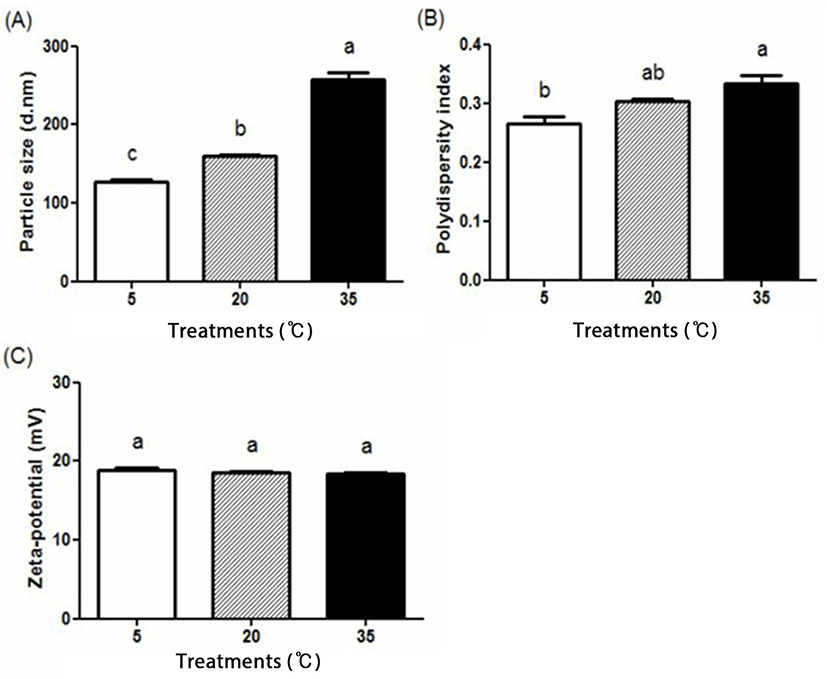
A significant (p<0.05) increase in the PDI values of CSO/A2 β-casein NDSs from 0.265 to 0.333 was observed with an increase in manufacturing temperature from 5°C to 35°C (Fig. 5B) implying that less uniform (polydispersed) particles were manufactured at 35°C. A similar trend was noticed in TEM micrographs (Fig. 4). Compared with CSO/A2 β-casein NDSs treated at 5°C and 20°C, CSO/A2 β-casein NDSs formed at 35°C had less homogeneous particles with broader size distribution (Fig. 4).
The zeta-potential values of CSO/A2 β-casein NDSs manufactured with various low-temperature treatment were presented in Fig. 5C. All NDSs had zeta-potential values about +18 mV siginifying that CSO/A2 β-casein NDSs had positive surface charges and good colloidal stability. No significant differences in zeta-potential values of NDS were observed in all manufacturing temperature conditions (p<0.05).
Impacts of CSO concentration level and low-temperature treatment on the EE of resveratrol in CSO/A2 β-casein NDSs were exhibited in Fig. 6. The EE of resveratrol in CSO/A2 β-casein NDSs was above 87%, which could be much higher than previous resveratrol EE results (40%–75%) (Bu et al., 2013; Gokce et al., 2012; Jeong et al., 2016; Sanna et al., 2012; Zu et al., 2014). CSO concentration level did not significantly affect the EE of resveratrol in CSO/A2 β-casein NDSs while a significant (p<0.05) increase in the EE of resveratrol from 87% to 90% was noticed with an increase in manufacturing temperature from 5°C to 35°C (Fig. 6). It is belived that major driving forces for interactions between hydrophobic bioactives and biopolymers are hydrophobic interactions (associations) (Ha et al., 2013; Ishihara and Mizushima, 2010). Since CSO is hydrophilic and A2 β-casein is hydrophobic in nature, the entrapment of hydrophobic resveratrol in CSO/A2 β-casein NDSs may be driven by interaction with A2 β-casein rather than CSO. Therefore, CSO concentration level did not affect the EE of resveratrol in CSO/A2 β-casein NDSs (Fig. 6A). On the other hand, hydrophobicity of β-casein is known to be increased with an increase in temperature (Bachar et al., 2012; Faizullin et al., 2013; Horne, 1998). An increase in the hydrophobicity of A2 β-casein with an increase in manufacturing temperature may lead to enhance the binding of resveratrol with A2 β-casein via hydrophobic interactions (associations). Therefore, it may result in an increase in the EE of resveratrol in CSO/A2 β-casein NDSs at higher manufacturing temperature (Fig. 6B). Esmaili et al. (2011) reported that an increase in the temeprature from 25°C to 37°C led to an increase in the binding constant of hydrophobic curcumin with β-casein about 46 times due to an increase in the hydrophobic associations among β-casein and curcumin.
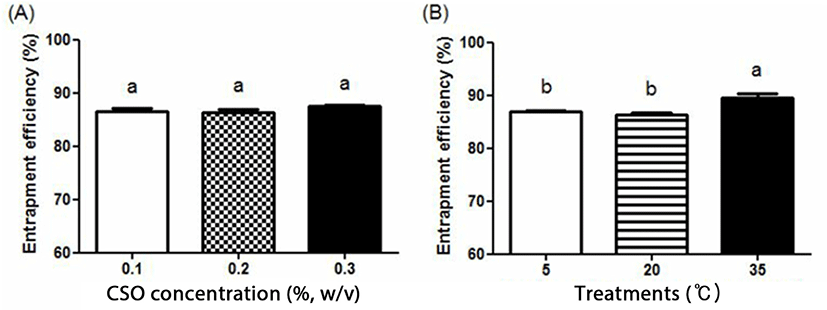
For long-term storage, CSO/A2 β-casein NDSs were freeze-dried. The size and polydispersity index value of freeze-dried CSO/A2 β-casein NDSs (size of 130.9±7.4 and polydispersity value of 0.268±0.016) was not significantly (p<0.05) different compared with CSO/A2 β-casein NDSs before freeze-drying (size of 126.9±6.0 and polydispersity value of 0.265±0.020) (data not shown). It indicates that CSO/A2 β-casein NDSs had excellent stability against freeze-drying. For the application of NDSs to dairy foods and foods for special dietary use, freeze-dried CSO/A2 β-casein NDSs were assessed under food processing and storage conditions (i.e., heat treatment and pH) (Figs. 7 and 8). For dairy food application, heat treatment at 90°C for 30 min, a process condition used to produce goat milk yogurt, was used. In addition, heat treatment at 125°C for 15 min was also applied as accelerated heating condition because the most foods for special dietary use are retort processed. Heat treatments at 90°C and 125°C did not significantly affect the size and polydispersity index value of CSO/A2 β-casein NDSs (Fig. 7A and B), which imply that CSO/A2 β-casein NDSs were very stable to high heat treatment. On the physical stability of CSO/ A2 β-casein NDSs in model yogurt (pH 4.8) and milk (pH 7.0) during storage for 14 days, no significant (p<0.05) changes in the size and polydispersity index value of CSO/A2 β-casein NDSs were observed (Fig. 8) indicating the that CSO/A2 β-casein NDSs possess remarkable physical stability under dairy food storage conditions.
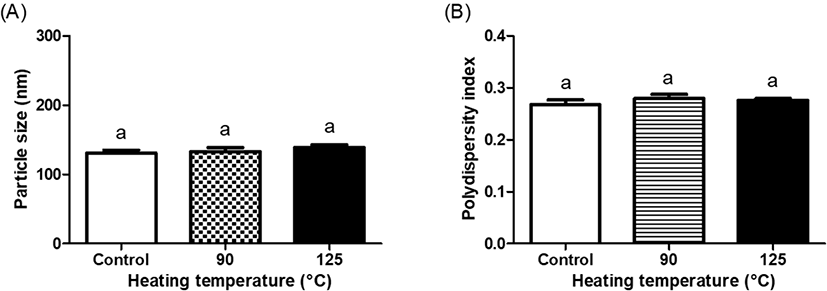
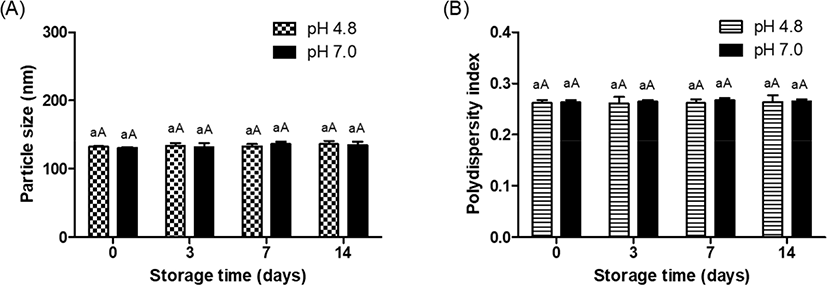
Conclusions
It was concluded that NDSs containing resveratrol were successfully produced with CSO and A2 β-casein extract from goat milk using an ionic gelation method with TPP. In this study, we found that CSO concentration level and low-temperature treatment were the key parameters affecting the formation and physicochemical characteristics, such as particle size, PDI, zeta-potential values, of CSO/A2 β-casein NDSs. More than 87% of resveratrol were successfully entrapped in CSO/A2 β-casein NDSs indicating that the modulation of low-temperature treatment could be an effective way to enhance the EE of resveratrol in NDSs. CSO/A2 β-casein NDSs had excellent physical stability under diary food processing and storage conditions indicating that CSO/A2 β-casein NDSs are highly potential to be applied to dairy foods.