Introduction
Whey protein, a by-product of cheese manufacturing, consists of bioactive ingredients including serum albumin, immunoglobulins, lactoferrin, lactoperoxidase, α-lactoglobulin, β-lactalbumin, phospholipoprotein, and enzymes (Madureira et al., 2010). Recently, this dietary protein has received considerable attention with regard to its health benefits on muscle mass, modulation of immune function, suppression of oxidative stress, and anti-cancer activities (Krissansen, 2007; Madureira et al., 2010). In particular, chronic ingestion of whey protein improves blood pressure and arterial stiffness in overweight and obese individuals (Pal and Ellis, 2010). Furthermore, whey protein hydrolysate attenuates tumor necrosis factor (TNF)-α-triggered endothelial inflammation by normalizing expression of TNF-α, endothelial nitric oxide synthase (eNOS), and vascular cell adhesion molecule (VCAM)-1 (Da Silva et al., 2017). Although whey protein contributes to multiple physiological functions, little is known about the molecules and pathways that mediate its effects.
Cellular senescence is a permanent and irreversible nonreplicating state characterized by growth arrest, increased expression of senescence-associated β-galactosidase (SA β-gal), and accelerated attrition of telomeres (Pazolli and Stewart, 2008). In primary cultured cells, DNA damage triggered by various oxidative stresses and chemical agents, including hydrogen peroxide, ultraviolet, and angiotensin (Ang) II, induces so-called stressinduced premature senescence (SIPS) (Toussaint et al., 2000; Touyz and Schiffrin, 2000). Recent work showed that Ang II triggers senescence of vascular smooth muscle cells (VSMCs) by triggering oxidative DNA damage (Herbert et al., 2008). In particular, accelerated senescence-associated phenotypic changes, such as oxidative DNA damage, are observed in VSMCs in atherosclerotic plaques (Matthews et al., 2006). On the other hand, inhibition of Ang II activity attenuates endogenous signals that induce vascular senescence, thereby inhibiting aging-associated vascular diseases such as atherosclerosis (Najjar et al., 2005). Hence, control of vascular senescence represents a potential strategy for treating aging-related vascular disorders. Among diverse anti-senescent factors, the NAD-dependent deacetylase SIRT1 is highly expressed in the vasculature and exerts a pivotal role in cardiovascular function (Potente et al., 2007). SIRT1 exerts beneficial effects in senescence-associated vascular diseases by preventing stress-induced vascular remodeling, neointima formation, and atherosclerosis (Gao et al., 2014; Kim et al., 2012; Li et al., 2011a). In human atherosclerosis, reduction in SIRT1 levels in VSMCs results in oxidative stress, exacerbating DNA damage and atherosclerosis (Gorenne et al., 2013; Zhang et al., 2008). In addition, we recently demonstrated that peroxisome proliferator-activated receptor d (PPARd)-dependent upregulation of SIRT1 inhibits premature senescence of human endothelial cells, as well as the migration and proliferation of VSMCs triggered by Ang II and oxidized low-density lipoprotein (oxLDL) (Hwang et al., 2016; Kim et al., 2012). Thus, the intervention of anti-senescence proteins such as SIRT1 may relieve or prevent pathological states in which cellular senescence is a primary causal factor (Gorenne et al., 2013; Ota et al., 2008).
Given its vascular bioactivities and its potential use as a dietary supplement, we therefore hypothesized that whey protein affects cardiovascular function by modulating the aging process of VSMCs. However, the potential effect of whey protein on vascular senescence induced by Ang II has not yet been explored. In this study, we demonstrated that whey protein suppresses Ang II-induced premature senescence of VSMCs by upregulating SIRT1 expression.
Materials and Methods
Ang II, MTT (3-(4,5-dimethylthiazol-2-yl)-2,5-diphenyltetrazolium bromide), polyclonal rabbit anti-β-actin antibody, resveratrol, SA β-galactosidase staining kit, sirtinol, and whey protein (W1500) were obtained from Sigma-Aldrich Co. (USA). The β-galactosidase enzyme assay kit and luciferase assay system were obtained from Promega (USA). Rabbit anti-SIRT1 polyclonal antibody and horseradish peroxidase (HRP)-conjugated IgG were obtained from Santa Cruz Biotechnology (USA).
Rat aortic VSMCs were isolated from thoracic aorta, as described previously (Hwang et al., 2016). Briefly, aorta dissected from male Sprague-Dawley rats was cut longitudinally, and the endothelial cells were removed. The residual medial membrane was cut into small pieces and maintained for 1 d. Following a change of fresh medium, the fragmented tissue was incubated for an additional couple of days. Migrated VSMCs were cultured in DMEM (Dulbecco’s modified Eagle’s medium) containing antibiotic and 10% FBS (fetal bovine serum) at 37°C in an atmosphere of 95% air and 5% CO2. Cells were used at passages 4-7 for all experiments.
To evaluate cell viability, aortic VSMCs in 24-well plates were incubated in the presence or absence of 600 μg/mL whey protein for the indicated time period, or with various concentrations of whey protein for 24 h. Thereafter, the cells were incubated for an additional 2 h in medium containing MTT solution (0.1 mg/mL). Following removal of the medium, the formazan crystals were dissolved in acidified isopropanol, and the absorbance at 570 nm was measured.
SA β-gal activity was detected using the SA b-galactosidase staining kit. Briefly, VSMCs plated on 60 mm culture dishes were pretreated with or without whey protein for 24 h, and then exposed to vehicle (DMSO) or Ang II for 72 h. Following washing with ice-cold phosphate-buffered saline (PBS), the cells were stained with SA β-gal staining solution (1 mg/mL X-Gal, 40 mM sodium phosphate, 150 mM NaCl, 40 mM citric acid, 5 mM potassium ferrocyanide, 2 mM MgCl2, 5 mM potassium ferricyanide, pH 6.0) at 37°C for 24 h. SA β-gal–positive cells were visualized on an Olympus JP/1X71 fluorescence microscope (Tokyo, Japan). Stained cells in four individual fields were counted by an independent observer. Data are represented as percentages of total cell number.
VSMCs exposed to the indicated reagents for the indicated period of time were washed with ice-cold PBS and then lysed in PRO-PREP Solution (iNtRON Biotechnology, Korea). Aliquots of the resulting whole-cell lysates were subjected to SDS-PAGE (polyacrylamide gel electrophoresis) and transferred onto a Hybond-P+ membrane (Amersham Biosciences Ltd., UK). Membranes blocked for 1 h at room temperature in Tris-buffered saline (TBS) containing 3% skim milk and Tween-20 (final 0.1%) were incubated overnight at 4°C with the indicated primary antibodies in TBS containing 0.05% Tween-20. After reaction with peroxidase-conjugated secondary antibody (1:5000 dilution) at room temperature for 1 h, membranes were extensively washed in TBS containing Tween-20 (final 0.1%). The immunoreactive proteins were then detected using WesternBright ECL (Advansta Co., USA).
For analysis of SIRT1 mRNA, total RNA was extracted and converted into cDNA using a reverse transcription kit (TOPscript RT DryMIX, Enzynomics, Korea). Real-time PCR was performed using equal amounts of cDNA in a 10 μL reaction volume containing primers and 1× SYBR PCR mix (Takara Bio Inc., Japan). PCR conditions were as follows: initial denaturation at 94°C for 20 min; followed by 40 cycles of 25 s at 95°C, 40 s at 58.5°C, and 35 s at 72°C. Primers were as follows: SIRT1, 5’-AGA ACC ACC AAA GCG GAA A-3’ and 5’-TCC CAC AGG AGA CAG AAA CC-3’; and GAPDH, 5’-TCA AGA AGG TGG TGA AGC AG-3’ and 5’-GCA TCA AAG GTG GAA GAA TGG G-3’. The SIRT1 mRNA level in each sample was normalized against the corresponding level of GAPDH mRNA.
The luciferase vector containing the mouse SIRT1 promoter (−2487 to −30 in pGL4) was a gift from Dr. Toren Finkel (NIH, USA). VSMCs plated in 6-well plates were co-transfected with 0.5 μg pSV b-Gal (SV40 b-galactosidase expression vector) and 1 μg SIRT1 luciferase reporter plasmid using SuperFect reagent (Qiagen, USA). Following incubation for 24 h, the cells were exposed to the indicated concentrations of whey protein for 72 h. The cells were then lysed by addition of luciferase reporter lysis buffer (Promega), and aliquots of the lysates were used to determine luciferase activity. Luciferase activity in each sample was normalized against the corresponding β-galactosidase activity to adjust for variations in transfection efficiency.
Results and Discussion
To assess the optimal concentration and treatment duration of whey protein, we performed MTT assays to determine cell viability. When VSMCs were treated with increasing concentrations (0, 50, 100, 200, 300, 400, 500, 600, 700, 800, 900, and 1000 μg/mL) of whey protein for 24 h, we observed no significant effect of whey protein on cell viability up to 600 mg/mL (Fig. 1A). Furthermore, high cell viability was maintained for up to 5 d in VSMCs treated with 600 mg/mL whey protein (Fig. 1B). Accordingly, we chose 600 mg/mL whey protein as the optimal concentration for subsequent experiments.
Next, we investigated whether whey protein could affect premature senescence of VSMCs triggered by Ang II. VSMCs exposed to Ang II for 3 d exhibited a significant increase in SA β-gal activity, a biomarker of cellular senescence, in comparison with control cells exposed to vehicle alone (DMSO). However, this increase was significantly attenuated in the presence of whey protein (Fig. 2A and 2B). These observations are in line with previous reports showing that whey protein from sheep protects endothelial cells from tert-butyl hydroperoxide (tBHP)-induced oxidative stress by regulating cellular levels of glutathione (GSH) (Kerasioti et al., 2016). In addition, whey protein downregulates the TNF-α-induced expression of inflammation-related genes in vascular endothelial cells (Da Silva et al., 2017). Moreover, whey protein lowers blood pressure in prehypertensive and mildly hypertensive individuals (Fekete et al., 2016). Although the molecular mechanisms underlying whey protein-mediated vascular homeostasis have not been fully elucidated, our observations clearly indicated that whey protein inhibits Ang II-induced premature senescence of VSMCs, suggesting that whey protein could be useful a dietary supplement to promote vascular health.
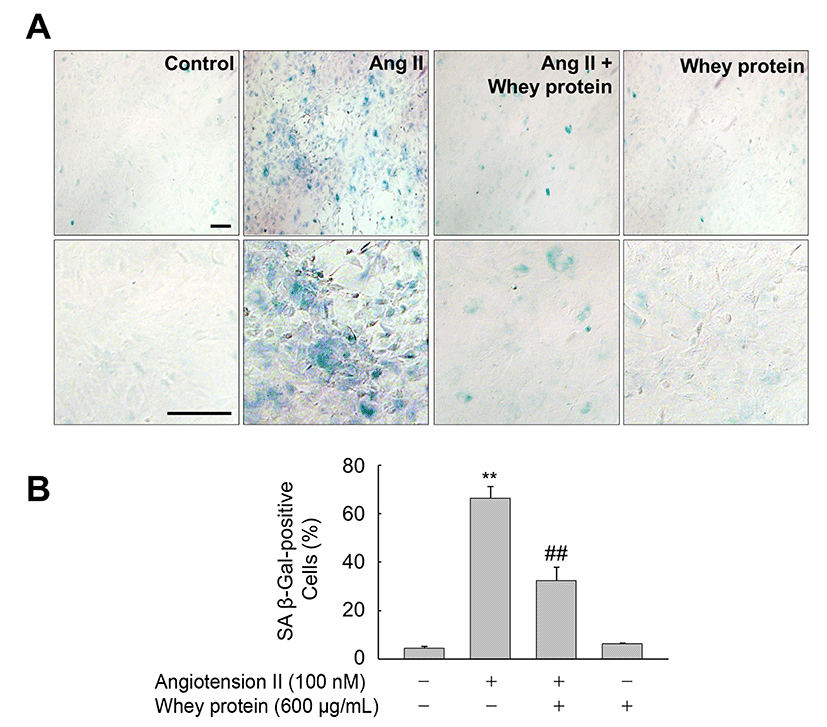
Because SIRT1 is a pro-longevity gene that plays an important role in the regulation of lifespan in organisms from yeast to mammals (Haigis and Sinclair, 2010), we investigated the effect of whey protein on SIRT1 expression in VSMCs. As expected, whey protein significantly increased the levels of SIRT1 protein in a concentrationand time-dependent manner. The maximal levels of SIRT1 protein were obtained after 72 h of exposure to 50-600 g/mL whey protein (Fig. 3A). When VSMCs were treated with 600 mg/mL whey protein, an increase in SIRT1 expression was detected at 12 h and persisted for up to 72 h (Fig. 3B). Consistent with the SIRT1 protein levels, exposure of VSMCs to whey protein for 72 h also significantly induced the expression of SIRT1 mRNA. A significant increase in the SIRT1 mRNA level was detected at 10 mg/mL whey protein, and expression further increased up to 600 mg/mL of whey protein (Fig. 3C).
No previous study has demonstrated that whey protein is involved in the expression of SIRT1. Hence, we sought to verify that whey protein regulates the transcription of the SIRT1 gene. To this end, we transfected VSMCs with a luciferase reporter construct driven by the SIRT1 promoter. As shown in Fig. 3D, whey protein dramatically increased SIRT1 promoter activity in a concentration-dependent manner. This result was consistent with the observed increase in the levels of SIRT1 mRNA and protein, suggesting that whey protein transcriptionally modulates SIRT1 expression in VSMCs. Indeed, SIRT1 is implicated in the multiple biological processes, including aging, energy metabolism, inflammation, and stress responses (Brunet et al., 2004; Cohen et al., 2004; Feige and Auwerx, 2008; Yeung et al., 2004). Although caloric restriction induces the expression of SIRT1 in mammalian cells (Cohen et al., 2004), the expression of SIRT1 is also regulated by complex cellular networks, including diverse transcription factors such as p53, CREB (cAMP response element-binding protein), BRACA1 (breast cancer 1), TLX, the HIC:CtBP co-repressor complex, and E2F1 (Chen et al., 2005; Iwahara et al., 2009; Noriega et al., 2011; Wang et al., 2006; Wang et al., 2008; Yi and Luo, 2010). Recently, we showed that nuclear receptor PPARδ, a liganddependent transcription factor, promotes SIRT1 expression in human endothelial cells and rat VSMCs, thereby attenuating the cellular senescence, proliferation, and migration evoked by the atherogenic molecules Ang II and oxLDL, respectively (Hwang et al., 2016; Kim et al., 2012). Although these observations do not fully elucidate the precise mechanism involved in whey protein-mediated upregulation of SIRT1, our results clearly demonstrate that whey protein exerts anti-aging activities via upregulation of SIRT1. Further studies are necessary to identify which mechanism is responsible for upregulation of SIRT1 expression in VSMCs exposed to whey protein.
To investigate the direct impact of SIRT1 upregulation on the inhibition of Ang II-primed premature senescence, we monitored the levels of endogenous SIRT1 protein in VSMCs exposed to Ang II in the presence or absence of whey protein. When cells were exposed to Ang II, the levels of endogenous SIRT1 protein were markedly reduced in a time-dependent manner (Fig. 4A). However, addition of whey protein to Ang II-treated cells markedly restored the levels of endogenous SIRT1 protein, suggesting that SIRT1 is a critical factor in whey protein-mediated suppression of VSMC senescence by Ang II (Fig. 4B).
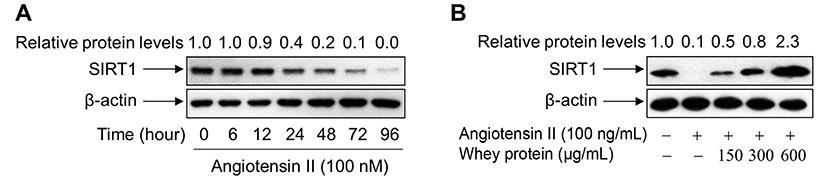
To further investigate whether whey protein-mediated upregulation of SIRT1 is important for inhibition of Ang II-induced VSMC senescence, we treated cells with chemicals that modulate SIRT1 activity. As shown in Fig. 5, sirtinol, a specific SIRT1 inhibitor, significantly counter-acted the inhibitory effects of whey protein on Ang II-induced premature senescence. On the other hand, activation of SIRT1 by resveratrol reduced the number of SA β-gal–positive cells after Ang II exposure to a degree similar to that of whey protein. Furthermore, addition of resveratrol to whey protein-treated VSMCs potentiated the inhibitory effect of whey protein. These results suggest that the inhibitory effect of whey protein on Ang II-induced premature senescence of VSMCs can be attributed, at least in part, to whey protein-mediated upregulation of SIRT1, a protein that has been shown to increase lifespan in mammals (Haigis and Sinclair, 2010). Although the molecular mechanism by which whey protein mediates upregulation of SIRT1 remains unclear, the results of manipulation of SIRT1 activity by sirtinol and resveratrol clearly demonstrated that whey protein functions in a SIRT1-dependent manner to suppress the Ang II-induced premature senescence of VSMCs. These beneficial effects in the vasculature are consistent with previous reports showing that a VSMC-specific SIRT1 transgene decreases Ang II-triggered vascular remodeling in mice (Gao et al., 2014). Furthermore, adenoviral-mediated overexpression of SIRT1 dramatically suppresses VSMC hypertrophy triggered by Ang II (Li et al., 2011b). In addition, VSMC-specific knockout of SIRT1 accelerates Ang II-induced formation and rupture of abdominal aortic aneurysms (AAAs) in ApoE-/- mice, along with age-related phenotypic changes such as vascular cell senescence (Chen et al., 2016). Several studies also demonstrated that vascular SIRT1 acts an anti-atherosclerotic (Yang et al., 2017; Yu et al., 2009; Zhang et al., 2016). Taken together, these observations indicate that whey protein is a promising dietary supplement that could be used to attenuate or prevent aging-associated vascular disorders.
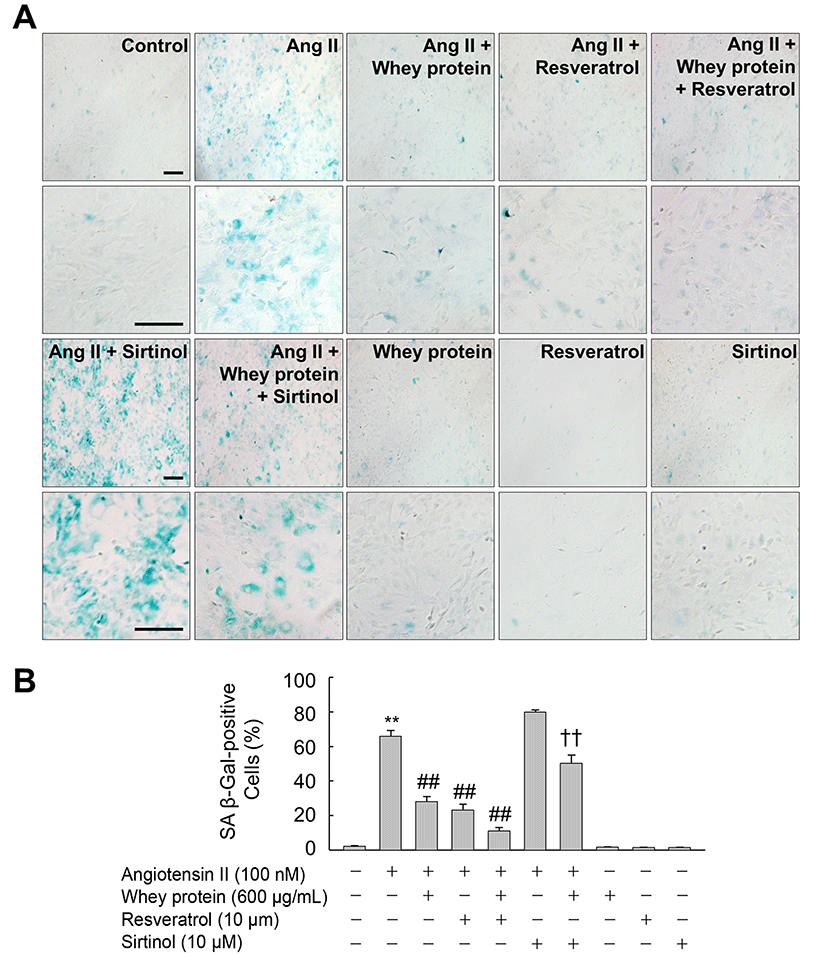
In summary, the present findings demonstrate that whey protein modulates premature senescence of VSMCs triggered by Ang II through transcriptional upregulation of SIRT1 expression. To our knowledge, this study provides the first evidence that whey protein-mediated upregulation of SIRT1 prevents vascular aging. This novel finding provides insights into the anti-senescence effects of whey protein, as well as the molecular mechanisms underlying SIRT1 expression. The anti-aging effect of whey protein in vasculature supports the use of dairy-based functional foods to maintain and improve vascular homeostasis.