Introduction
Enterococci are essential members of lactic acid bacteria (LAB) and can be found in a variety of food sources, such as meat, milk, cheese, vegetables, water surfaces, and plants (Jamet et al., 2012). They are also inhabitants of the gastrointestinal tract of human and animals (Foulquié Moreno et al., 2006; Carasi et al., 2014). There are 55 species, and 2 subtypes reported so far on the basis of 16S rDNA sequences (LPSN, 2017). These bacteria can play a beneficial role in food maturation processes as a starter or probiotic cultures (Klibi et al., 2013; Abouelnaga et al., 2016; Gaglio et al., 2016). In addition, some enterococci, mainly E. faecalis and E. faecium, produce inhibitory substances such as lactic acid, hydrogen peroxide, and bacteriocins, which are capable of inhibiting the growth of food pathogens and spoilage microorganisms (Raafat et al., 2016). In contrast to their importance in the food industry, they are not generally recognized as safe (GRAS) and their presence is often an indicator of faecal contamination (Frazzon et al., 2010). However, enterococci isolated from food have not been conclusively determined as direct causes of clinical infections (Carasi et al., 2014).
Enterococcus spp. have virulence genes and often exhibit high resistance to common antibiotics. They carry intrinsic as well as acquired resistance to most of the antibiotics used in humans (Sallem et al., 2016). The antibiotic resistance is conferred by horizontal transfer of resistant genes across different species and genus by conjugative plasmids and transposons (Belgacem et al., 2010; Jahan and Holley 2016). For the safety reason, an essential criterion for the selection of starter cultures in foods is the absence of transferable antibiotic resistance in enterococci (Frazzon et al., 2010; Oladipo et al., 2013). In addition, they have been found to exhibit increasingly multi-drug resistance in recent years. The aggregation substance, cell wall adhesin expressed in serum by E. faecium, collagen adhesion, cytolysin, hemolysin, enterococcal surface protein, gelatinase, and hyaluronidase are typical examples of virulence factors determined in enterococci (Aslam et al., 2012; Camargo et al., 2014; Abouelnaga et al., 2016). Some enterococcal species are recognized as important causes of nosocomial infections such as endocarditis, bacteremia, urinary tract, and central nervous system infections (Togay et al., 2010; Camargo et al., 2014; Abauelnaga et al., 2016). E. faecalis strains (80–90%) are the most prevalent Enterococcus spp. isolated from human infections, followed by E. faecium (5–20%), while the remaining Enterococcus spp. infrequently cause infections (Furlaneta-Maia et al., 2014).
The presence of antibiotic resistant enterococci in the foods of animal origin has been reported by several authors (Kasımoglu-Dogru et al., 2010; Aslam et al., 2012; Abouelnaga et al., 2016), posing a potential risk of transmission to humans (Carasi et al., 2014). Therefore, it has been suggested that enterococci isolated from foods should be tested in terms of potential antibiotic resistance (Togay et al., 2010; Klibi et al., 2013). Several studies have been focused on enterococcal antibiotic resistance isolated from dairy samples, but insufficient information is available about the prevalence, identification, and antibiotic resistance in enterococci isolated from pre-packaged chicken samples in Turkey. This study is the first report on the occurrence of antibiotic resistant enterococci in pre-packaged chicken samples in Ankara (Turkey).
This study consists of two parts: (i) isolate and identify new strains belonging to genus Enterococcus isolated from pre-packaged chicken samples and, (ii) evaluate them antimicrobial resistance using the disk diffusion method with twelve different antibiotic discs.
Materials and Methods
The enterococcal strains isolated in this study and the reference strains were grown in tryptic soy broth (TSB) (MerckTM, Germany) and brain hearth infusion (BHI) Broth (MerckTM, Germany), respectively, at 37°C for 24 h. The initial isolates were stored at –80°C in 30% (v/v) aqueous glycerol (MerckTM, Germany).
Enterococcus faecalis ATCC 29212, Enterococcus faecalis DMG 2708, Enterococcus faecium ATCC 19434, Escherichia coli LMG 3083 (ETEC), and Staphylococcus aureus ATCC 6538 were obtained from the culture collection of Prokaryote Genetics Laboratory, Department of Biology, Faculty of Science, Ankara University.
About 122 new brand pre-packaged chicken samples kept at +4°C were randomly purchased from various local markets, supermarkets and butcher shops in Ankara, Turkey. All of the chicken samples were taken in manufacturer-sealed packaging and collected from different companies without discrimination. The samples were collected under aseptic conditions and transported to the laboratory under cold conditions on the sampling day and processed immediately.
For the isolation of enterococci, 10 g of each chicken sample was homogenized in 90 mL of buffered peptone water (MerckTM, Germany) in a sterile stomacher bag, using a Seward 400 laboratory stomacher at medium speed for 2 min. Specific serial dilutions of the homogenates were prepared up to 10–5 in 0.85% (w/v) NaCl (MerckTM, Germany). Of each dilution, 100 µL was inoculated on kanamycin aesculin azide (KAA) agar (MerckTM, Germany) and then incubated at 37°C for 48 h. Two typical colonies of Enterococci on KAA were randomly selected from the highest dilution of each sample for further analysis (as detail below).
The pure cultures were identified to the genus level, using Gram staining, catalase production, growth in the presence of 6.5% NaCl, at pH 9.6, esculin hydrolysis on bile esculin azide agar (MerckTM, Germany), and optimum growth at 10−45°C (Abouelnaga et al., 2016). In addition, all isolates were identified to the species level using the API 20 STREP system (bioMerieuxTM, France). The results were also confirmed by the 16 S rDNA gene sequencing. E. faecalis ATCC 29212, E. faecalis DMG 2708, and E. faecium ATCC 19434 were used as positive control in the corresponding API 20 STREP system and PCR reactions. 100–200 µL of each strain grown overnight in TSB broth (approximately 107 CFU) were pelleted by centrifugation at 12.500×g for 5 min. The pellets were washed twice with sterile water in a clean 1.5 mL microcentrifuge tube and re-pelleted by centrifugation. Total DNA was extracted from washed cell pellets by a standard alkaline lysis method as previously reported described (Cancilla et al., 1992). The DNA was stored at –20°C. The PCR procedures used in this study have been described previously (Blaiotta et al., 2002). The primer pair 907r (CCGTCAATTCMTTTRAGTTT) and 27f (AGAGTTTGATCMTGGCTCAG) proposed by Beasley and Saris (2004) were used to amplify 16S rDNA gene. The PCR procedure was performed in a total reaction volume of 50 µL that included 3 µL bacterial DNA template, 34.75 µL RNase/DNase free water, 0.25 µL Taq DNA polymerase in the reaction buffer, 1 µL of 2 mM each dNTP, 4 µL of 25 mM MgCl2, 1 µL of each primer and 5 µL of 1× PCR buffer. The amplification reactions were carried out in a Thermocycler (Techne TC–512, Staffordshire, UK). The PCR conditions were as follows: (1) initial hold of 2 min at 95°C, (2) denaturation step at 95°C/45 s, annealing at 55°C/45 s, extension at 72°C/2 min and (3) final extension step at 72°C/7 min. The PCR products purified using a GeneJET PCR purification kit (Thermo ScientificTM) were confirmed using 1% agarose gel electrophoresis, stained with 0.1 µg/mL ethidium bromide solution and visualized under UV light.
Hemolytic activity was determined on TSA containing 5% (w/v) sheep blood plates and incubated at 37°C for 24–48 h under anaerobic conditions (Valenzuela et al., 2009). The β-hemolysis isolates caused complete cell lysis and were identified by a clear zone of hydrolysis around the inoculated colonies. The test was performed in duplicate. E. coli LMG 3083 (ETEC) and S. aureus ATCC 6538 were used as controls.
Antibiotic resistance was determined in all isolates using the disk diffusion method following the Clinical Laboratory Standards Institute guidelines (CLSI, 2011). The following twelve different antibiotic discs (BioanalyseTM, Turkey) were tested: penicillin G (10 µg/disc), kanamycin (30 µg/disc), ampicillin (10 µg/disc), rifampicin (5 µg/disc), chloramphenicol (30 µg/disc), erythromycin (15 µg/disc), gentamicin (120 µg/disc), tetracycline (30 µg/disc), vancomycin (30 µg/disc), nalidixic acid (30 µg/disc), streptomycin (300 µg/disc), and ciprofloxacin (5 µg/disc). E. faecalis ATCC 29212, E. faecium ATCC 19434, and S. aureus ATCC 6538 strains were used as the quality control strains. According to the inhibition zone measured, the strains were categorized as susceptible, intermediate or resistant by taking into account the criteria of the CLSI.
Results
A total of 122 pre-packaged chicken samples collected from various markets, supermarkets and butcher shops in Ankara were screened for presumptive enterococci, 97 of them (79.50%) with positive results (data not shown). All of the isolates showed optimum developmental characteristics at pH 9.6, 6.5% NaCl and 10–45oC. In addition, 97 isolates were also identified as Gram (+), catalase (−), and esculin hydrolysis (+). In the API 20STREP identification, the most prevalent enterococcal species were E. faecium, which represented 86.59% (84 of 97) of the isolates followed by E. faecalis (13.41%, 13 of 97). The molecular identification using PCR universal primers for 16S rDNA genes indicated that 61.85% isolates (60 of 97) were E. faecium and 38.15% isolates (37 of 97) were E. faecalis. PCR screening of 16S rDNA gene from Enterococcus species is shown Fig. 1. E. faecium was found to be the most prevalent species in chicken samples followed by E. faecalis. When the identification results obtained with API 20 STREP and 16S rDNA sequence analysis were compared, it was interesting to note that 28 out of 84 E. faecium isolates and 4 out of 13 E. faecalis isolates were not correctly identified at species level by API 20 STREP. API 20 STREP identification of 65 isolates was in agreement with 16S rDNA sequencing as can be seen in Table 1.
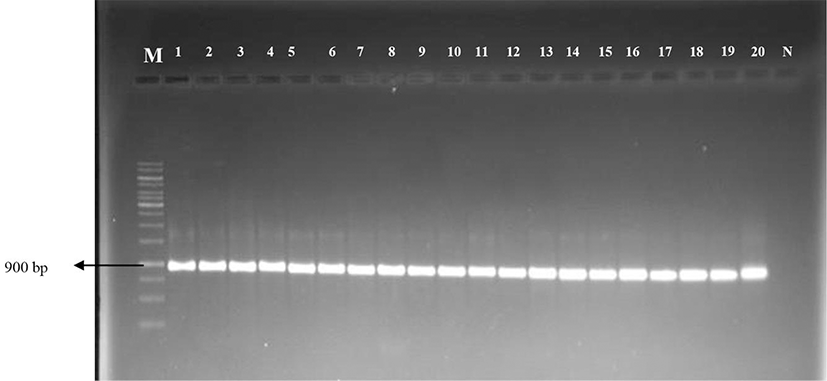
Hemolytic activity of enterococcal strains is shown in Table 1. Of the 97 isolates tested for hemolytic activity, 12.37% enterococcal strains (12 of 97) showed β-hemolytic character. While 13.40% enterococcal strains (13 of 97) exhibited γ-hemolytic character, 74.23% other enterococcal strains (72 of 97) were found to be α-hemolytic. β-Hemolysin was most prevalent among E. faecium (58.33%, 7 of 12) compared to E. faecalis (41.66%, 5 of 12).
A summary of the resistance among the Enterococcus isolates is reported in Table 2. All of the Enterococcus strains used in this study were resistant to nalidixic acid (100%). Of the 97 enterococcal species detected, most isolates exhibited resistance to kanamycin (98.96%, 96 of 97) followed by rifampicin (80.41%, 78 of 97) and ampicillin (60.82%, 59 of 97). The resistance to erythromycin (38.14%, 37 of 97) and ciprofloxacin (34.02%, 33 of 97) was also observed. In contrast, very few isolates (≤10 per antimicrobial) were resistant to tetracycline (9.27%, 9 of 97), penicillin G (8.24%, 8 of 97), chloramphenicol (3.09%, 3 of 97), gentamicin (2.06%, 2 of 97), and streptomycin (1.03%, 1 of 97). In addition, none of the isolates was resistant to vancomycin.
a The diameters of the zones were compared with the diameters specified by the Clinic Laboratory Standards Institute (CLSI, 2011).
The multi-drug resistance defined as the resistance to three or more antimicrobial agents was found in 97.93% (95 of 97) of Enterococcus strains. The profiles of multiple resistance are presented in Table 2. A total of 97 Enterococcus strains displayed resistance to at least two antibiotics (from 2 to 6 out of the 12 antibiotics tested). Only two E. faecalis strains were resistant to kanamycin and nalidixic acid. Eighteen isolates were resistant to three antibiotics, 32 isolates were resistant to four antibiotics, and 34 isolates were resistant to five antibiotics. Moreover, 11 Enterococcus spp. (5 E. faecalis and 6 E. faecium) were resistant to six antibiotics. The majority of enterococcal isolates were resistant to five antibiotics followed by four antimicrobials. In total, 60 E. faecium strains (100%, 60 of 60) showed resistant profiles against three or more tested antibiotics, but for the same parameter, only 35 E. faecalis strains (94.59%, 35 of 37) were resistant. When the antibiotic resistance levels of the enterococcal species were compared, E. faecium strains showed a more resistant phenotype than E. faecalis strains. The data of the resistance in E. faecium and E. faecalis strains against antibiotics were statistically significant (p>0.05).
Discussion
This study was firstly focused on the isolation and identification of new strains belonging to genus Enterococcus in pre-packaged chicken samples and then to determine their antibiotic resistance. Detection of antibiotic resistance in pre-packaged chicken products is the first study in Ankara. In this study, enterococci were isolated from 79.50% of chicken samples. This was consistent with the previous studies, which reported that the percentage of positive samples of enterococci in poultry was 95% in the USA (McGowan et al., 2006), 52.5% in Brazil (Gomes et al., 2008), 78% in Turkey (Kasımoglu-Dogru et al., 2010), 94% in Canada (Aslam et al., 2012), 80% in Tunisia (Klibi et al., 2013), and 100% in Brazil (Camargo et al., 2014), Pasevento et al. (2014) detected the lower percentage of enterococci than that reported by us. These differences might be attributed to the regional discrepancies or isolation methods.
It was revealed by PCR that the most prevalent species was E. faecium followed by E. faecalis. In line with the earlier study (Kasımoglu-Dogru et al., 2010), E. faecium was the most frequently isolated species from chicken in Turkey. On the other hand, some authors reported E. faecalis as the predominant species (McGowan et al., 2006; Frazzon et al., 2010; Aslam et al., 2012; Jahan et al., 2013; Klibi et al., 2013; Pasevento et al., 2014). When comparing the identification results obtained by API20 STREP and 16S rDNA sequence analysis, API20 STREP identification of 32 isolates was not in agreement with 16S rDNA sequencing. Our findings indicated that API identification gave erroneous identification results in some cases. Similar results were previously reported by Lavova et al. (2014) and Elmacı et al. (2015).
In this study, a higher frequency of α-hemolysin (74.23%) than β-hemolysin (12.37%) was observed, in agreement with Gomes et al. (2008), Barbosa et al. (2010), and Camargo et al. (2014). We also found that β-hemolysin was more prevalent among E. faecium compared to E. faecalis. This finding was similar to that reported by Camargo et al. (2014), but did not agree with the results of Gomes et al. (2008), who found that E. faecalis strains (38.7%) were dominant to produce β-hemolysins. Nevertheless, the absence of β-hemolytic activity in enterococci does not mean that these bacteria are not virulent (Barbosa et al., 2010).
Among the 97 enterococcal strains encountered in this study, resistance to nalidixic acid was the highest. Most enterococci possess intrinsic resistance to nalidixic acid (Miller et al., 2014). The incidence of resistance to nalidixic acid in all enterococcal isolates was similar to that reported by Khan et al. (2005) and Dada et al. (2013) but was higher than that reported by Furlaneto-Maia et al. (2014). Lower resistance to streptomycin and gentamicin was noticed in this study (1.03% and 2.06%, respectively). Streptomycin resistance was identified in one E. faecium isolate that also exhibited resistance to kanamycin, gentamicin, tetracycline, and nalidixic acid. In addition, resistance to gentamicin (3.8%) was observed in two strains (one E. faecalis and one E. faecium). Only one strain of E. faecium exhibited resistance to both gentamicin and streptomycin. These antibiotics have a synergistic effect when used together as a cell wall active agent (Miller et al., 2014). The resistance rates of both streptomycin and gentamicin were noted to be low since the use of them in poultry has been banned in Turkey. For the antimicrobials tested, the highest levels of the resistance were found against kanamycin and rifampicin (98.96 % and 80.41%, respectively). Kanamycin resistance is chromosomally encoded. In this study, only one E. faecium strain was sensitive to kanamycin. The incidence of resistance to rifampicin in all enterococcal isolates was similar to that reported by Barbosa et al. (2010), but in contrast to our results, lower levels of resistance to kanamycin were reported in a previous study (Guerrero-Ramos et al., 2016). Among other antimicrobials tested, no resistance was found to vancomycin. This result was in agreement with previous studies that noted the absence of acquired vancomycin resistance in enterococci (VRE) from poultry (McGowan et al., 2006; Barbosa et al., 2010; Kasımoglu-Dogru et al., 2010; Jahan et al., 2013; Klibi et al., 2013). However, some authors have detected VRE from chicken in Spain (Robredo et al., 2000), Germany (Sting et al., 2013), Turkey (Çetinkaya et al., 2013), Italy (Pesavento et al., 2014), and Iran (Talebi et al., 2015). The incidence of VRE in different countries could be due to the different policies regarding the antibiotic use in animal husbandry. The first incidence of VRE was reported in European countries in 1988 (Aslam et al., 2012; Iosifidis et al., 2013) and then it has frequently been detected in other countries. Avoparcin is a similar glycopeptide of vancomycin, which is used as a growth promoter in animal production. The prevalence of VRE has considerably decreased as a consequent of prohibiting the use of avoparcin in 1997 in European countries (Barbosa et al., 2010) and in Turkey in 1999 (Kürekçi et al., 2016). The lower incidence of erythromycin and tetracycline resistance was observed among the enterococcal isolates (38.14% and 9.27%, respectively). These antibiotics are commonly used in animal husbandry (Klibi et al., 2013). Tetracycline resistance is generally associated with the presence of the gene tetM and tetQ; however, the active efflux mechanism is conferred by tetK and tetL efflux pump genes. Furthermore, tetracycline resistance has largely been identified in clinical isolates (Garrido et al., 2014). In contrast to our study, Frazzon et al. (2010), Klibi et al. (2013), Pesavento et al. (2014), Guerrero-Ramos et al. (2016), and Raafat et al. (2016) found a much higher incidence of resistance to tetracycline as 60%, 41%, 35.3%, 67.3%, and 53%, respectively. The resistance to erythromycin is a matter of concern because it is used as an alternate in patients with penicillin allergy (Barbosa et al., 2010). Therefore, the high resistant rate of erythromycin determined in this study is worrying. The incidence of erythromycin resistance was lower than that of reported by Kasımoglu-Dogru et al. (2010), Jahan et al. (2013), and Pesavento et al. (2014). Enterococci are considered intrinsically resistant to β-lactam antibiotics like ampicillin and penicillin owing to their prolonged use in the treatment of enterococcal infections (Barbosa et al., 2010; Garrido et al., 2014). In our study, resistance to ampicillin (60.82%) was higher than that to penicillin G (8.24%). Eight strains were determined as resistant to penicillin G. In agreement with the McGowan et al. (2006), Jahan et al. (2013), Pesavento et al. (2014), Bulajic et al. (2015), and Gaglio et al. (2016), we detected low resistance to penicillin G. The higher levels of ampicillin resistance in enterococci are achieved by the higher levels of penicillin-binding protein 5(PBP5) expressions (Vrabec et al., 2015). This antibiotic is frequently used for the treatment of enterococcal infection (Frazzon et al., 2010). Therefore, this phenotype in chicken is alarming. Of the 97 strains tested, only three strains of Enterococcus spp. were resistant to chloramphenicol (3.09%). The use of chloramphenicol for human treatment is low due to its side effect (Barbosa et al., 2010). The low prevalence of chloramphenicol observed in this study may be explained by the fact that, in Turkey, the use of chloramphenicol for animal husbandry was banned in 2002 (Kasımoglu-Dogru et al., 2010). In contrast, Barbosa et al. (2010), Jahan et al. (2013), and Yuksel et al. (2015) found 94.5%, 10.3%, and 46.6% of enterococci to be chloramphenicol resistant. Ciprofloxacin is a group of antibiotics belonging to fluoroquinolones. It exhibits only a weak effect on enterococci (Barbosa et al., 2010). Of the 97 isolates investigated, 34.02% enterococcal strains demonstrated resistance to ciprofloxacin. This may be associated with the use of antibiotics in human and veterinary medicine. The incidence of ciprofloxacin resistance was higher than that reported by Jahan et al. (2013) and Pesavento et al. (2014).
Among all isolates, 97.93% enterococcal strains showed resistance to three or more antimicrobial agents. The results similar to those reported in this study were also obtained by Jahan et al., (2013) (59%), Abouelnaga et al. (2016) (83%), and Rafaat et al. (2016) (78%). In contrast, a lower incidence (24.59%) was found by Bulajic et al. (2015). The majority of enterococcal isolates were resistant to five antibiotics (35.05%) followed by four antimicrobials (32.98%). In addition, E. faecium strains showed a more resistant phenotype than E. faecalis strains, as reported earlier (Delpech et al., 2012). Previous studies (Gomes et al., 2008; Frazzon et al., 2010; Aslam et al., 2012; Pesavento et al., 2014; Yuksel et al., 2015; Kürekçi et al., 2016) showed that E. faecalis strains have a more resistant phenotype than E. faecium. Interestingly, when the antibiotic resistance levels of the enterococcal species identified were compared, E. faecium strains showed a more resistant phenotype than E. faecalis strains. Similar findings were also reported by Serio et al. (2007), Furlaneto-Maia et al. (2014), and Delpech et al. (2012).
Conclusion
This study has been focused on the isolation and identification of new strains belonging to genus Enterococcus isolated from chicken retailed in Turkey and then to determine their antibiotic resistance. This study was the first report on the occurrence of antibiotic resistant enterococci in pre-packaged chicken samples. The results of this study indicated that E. faecium is the dominant Enterococcus species present in chicken. Our results clearly indicated that E. faecalis and E. faecium strains isolated from pre-packaged chicken in Ankara could be considered a potential source for the dissemination of antibiotic resistance. In addition, the high prevalence of multi-drug resistance among Enterococcus species isolated from raw chicken samples is a serious threat to public health. Controlled use of antibiotics in animal husbandry is highly suggestive in Turkey.