Introduction
Antimicrobial resistance is now recognized as one of the most serious global threats to human health in the 21st century, as antimicrobial agents contribute not only to human medicine but also veterinary drug and animal-derived food production (Kang et al., 2018; Prestinaci et al., 2015). Of concern, there are now statements by the WHO and US Centers for Disease Control and Prevention describing a global crisis and an impending catastrophe involving a return to the pre-antibiotic era (McKenna, 2013; WHO, 2014). Food-producing animals are known as an important reservoir of antimicrobial-resistant zoonotic bacteria (Michael et al., 2017). These antibiotic resistant bacteria may colonize the human intestinal tract and contribute resistant genes to human endogenous flora (Card et al., 2017; Zeng and Lin, 2017).
The dietary pattern for Korean has changed to Western food culture (animal origin food). The food consumption of animal products have increased 68 g/day (1998) to 108 g/day (2014). Moreover, chicken meat is the most frequently consumed food (0.8 times a week) in Korea (Park et al., 2016), because chicken meat contains a balance of protein, minerals, and vitamins and is the cheapest meat from food-producing animals (Ahmed and Gareib, 2016). Antimicrobials are widely used in animal production to prevent and treat diseases as well as to improve growth performance (Pagel et al., 2012). The use of antimicrobials for animal production in Korea was about 936 tons in 2012 (Kim et al., 2014) and antimicrobials are most used for chicken production (Lim, 2007). Many countries including Korea have banned the use of antimicrobials in animal feed, but antibiotic residues and antimicrobial-resistant bacteria are still detected in food-producing animals (Marshall et al., 2011; Rho et al., 2012; Yoon et al., 2014).
A few studies have reported on antibiotic residues such as benzylpenicillin and trimethoprim in chicken meats in Korea (Jang et al., 2015; Lee et al., 2014). Moreover, correlation studies between antibiotic residues and antimicrobial-resistant bacteria in food-producing animals are still limited in Korea. In this study, we investigated 17 antibiotic residues and 6 antimicrobial-resistant patterns of E. coli isolated from commercialized chicken meat in Korea to improve food safety.
Materials and Methods
To detect antibiotic residues and antibiotic resistance of isolates, a total of 58 chicken meats were collected from retail grocery stores in five provinces (Gyeonggi, Chungcheong, Gyeongsang, Jeonla and Gangwon) in Korea between February and June 2016. About 0.5–1 kg were collected for each chicken sample. The samples were purchased and transported to laboratory into a collection container with ice pack. The samples were chopped and kept frozen in sterile plastic bags at a set storage temperature (–20℃). The experiments were performed for three times for one sample.
All standards were of high purity grade (>90%) and were supplied by Sigma-Aldrich (Darmstadt, Germany), Fluka (Buchs, Switzerland) and Dr. Ehrenstorfer (Augsburg, Germany). HPLC grade acetonitrile (ACN) and methanol (MeOH) were obtained from Merck (Darmstadt, Germany). Ammonium formate (≥99%) and formic acid (≥95%) were purchased from Sigma-Aldrich (Darmstadt, Germany). Analytical grade primary secondary amine (PSA), octadecylsilane (C18), and ethylenediaminetetraacetic acid disodium salt (Na2-EDTA) were purchased form Agilent Technologies (CA, USA) and Merck (Darmstadt, Germany). Polyvinylidene difluoride (PVDF) filters (15 mm diameter, 0.2 μm, Teknokroma, Barcelona, Spain) were used for filtration of extracts.
The chicken samples were chopped and homogenized with a blender and stored at −20℃ prior to analysis. Blank sample was fortified with the target compounds during the optimization and validation procedure. Target compounds were extracted from chicken meat using an extraction procedure based on the QuEChERS methodology (Lopes et al., 2012). The procedure was as follows: 2 g of sample was weighed in a 50 mL polypropylene centrifuge tube, followed by the addition of 1 mL of 50 mM ammonium acetate buffer in 0.1 M Na2-EDTA (pH 4.0) and 9 mL of acetonitrile containing 2 mM ammonium formate and 20% water solution. Then, samples were placed on a mechanical rotary shaker for 5 min at 200 rpm, centrifuged at 10,000×g for 10 min, and refrigerated at 4℃. An aliquot (10 mL) of the supernatant was transferred into a fresh polypropylene centrifuge tube containing PSA (250 mg) and C18 (250 mg) and then vortex-mixed for 30 s prior to centrifugation at 10,000×g for 10 min and refrigeration at 4℃. The lower layer (5 mL) was then transferred into a fresh centrifuge tube and dried under nitrogen at 40℃ until a volume of 1 mL remained. Finally, extracts were filtered through a PVDF syringe filter, and the filtrate was analyzed by LC-MS/MS (Waters, Milford, MA, USA). The analytes were separated on an XSELECT C18 column (2.1 mm×150 mm, 3.5 µm, Waters, Dublin, Ireland). The injection volume was 5 µL, and the temperature of the column oven was maintained at 40℃. The mobile phases were (A) 0.1% (v/v) formic acid in water and (B) 0.1% (v/v) formic acid and 2 mM ammonium formate in ACN with the following linear gradient flow program: 0 min, 10% B; 1 min, 10% B; 6 min, 40% B; 10 min, 95% B; 15 min, 95% B; 15.1 min, 10% B; 20 min, 10% B. The instrument was operated using electrospray ionization (ESI) in positive and negative modes. Data collection was implemented in multiple reaction monitoring (MRM) mode using MassLynx software.
The method was verified according to the Codex Alimentarius Commission (CODEX, 2009) guidelines, including requisite values for accuracy, precision, limit of detection (LOD), limit of quantification (LOQ), and linearity (CCRVDF, 2012). The validation level of each antibiotic was performed based on Korean MRLs in fishery products (Supplementary Table 1). The accuracy, expressed as recovery, was determined by comparing the calculated amounts of analytes spiked into the samples using the standard solutions. Precision was expressed in terms of the intraday relative standard deviation (RSD). The LODs and LOQs were calculated as three and 10 times the signal-to-noise ratio, respectively. Linearity was determined from matrix-matched calibrations constructed from six different concentrations.
The samples (25 g each) were aseptically added to 225 mL of EC broth (Oxoid, Basingstoke, UK) in a whirl-pak. The packs were stomached using a BagMixer 400 (Interscience, Paris, France) for 2 min. The mixed packs were incubated at 37℃ for 24 h. After enrichment, 10 µL of broth was streaked onto eosin-methylene blue agar (Difco, Detroit, MI, USA). The plates were then incubated at 37℃ for 24 h, and typical colonies were biochemically characterized using the VITEK MS system (Biomerieux, Marcy l’Etoile, France). For antibiotic resistance of the isolates, minimum inhibitory concentrations (MIC) were determined by broth microdilution using the KRNV4F Sensititre panel (Trek Diagnostic System, Cleveland, OH, USA) according to the manufacturer’s instructions. The MIC and antimicrobial resistance were determined according to the Clinical and Laboratory Standard Institute (CLSI) guidelines (CLSI, 2010). The used antibiotics were ampicillin (AMP), amoxicillin/clavulanic acid (AMC), ceftiofur (XNL), ciprofloxacin (CIP), trimethoprim/sulfamethoxazole (SXT), and tetracycline (TET).
Results and Discussion
In this study, we validated the analytical method for 17 antibiotic residues in chicken meat based on previous studies (Lopes et al., 2012; Shin et al., 2018). All values obtained for antibiotics were suitable for monitoring studies based on CODEX guidelines (CODEX, 2009). The accuracy, precision, LOQ and linearity of the calibration curve were calculated to verify the analytical method (Supplementary Table 2). Accuracy and precision were expressed as recovery and RSD. The recovery rates for chicken meat were over 90%, except in the cases of amoxicillin (82.4%–99.5%), ceftiofur (89.4%–94.6%), clindamycin (85.9%–94.0%), erythromycin (81.0%–104%), and tylosin (84.3%–87.05%). The lowest recovery was 79.3%–81.5% for tiamulin. The RSD was below 10%, except for amoxicillin (9.79%–15.6%). The LOQ of ceftiofur, chlortetracycline, enrofloxacin, tetracycline, and tylosin were 0.15 µg/kg, 0.02 µg/kg, 0.003 µg/kg, 0.02 µg/kg, and 0.07 µg/kg for chicken meat, respectively. Amoxicillin, ampicillin, benzylpenicillin (penicillin G), ciprofloxacin, and sulfamethoxazole showed LOQ of 0.01 µg/kg for chicken meat. Most of the observed recoveries exceeded 80%, and most RSD values were below 15%. Linearities, expressed as the square of the correlation coefficients, were greater than 0.98. The interfering peaks were not observed in chromatograms of chicken meat. Compared to previous studies, the recovery rate and RSD were satisfactory for majority of tested antibiotics in chicken meat, with high sensitivity and low detection limit (0.01 µg/kg) despite the complexity of the food matrices (Dasenaki and Tomaidis 2015; Dubreil et al., 2017; Shin et al., 2018).
The occurrence of antibiotic residues in the chicken meats is shown in Table 1. The total detection rate was 45% (26 out of 58). The most detected antibiotics were amoxicillin (1.43–3.41 μg/kg; 9 samples at 15.5%), followed by enrofloxacin (0.35–0.73 μg/kg; 7 samples at 12.1%), sulfamethoxazole (0.03–0.37 μg/kg; 6 samples at 10.3%), and ceftiofur (1.00–9.25 μg/kg; 5 samples at 8.6%). Residues of ciprofloxacin, tetracycline, and ampicillin were 0.28–0.61 μg/kg (3 samples at 5.2%), 0.32–0.41 μg/kg (3 samples at 5.2%), and 0.51–0.53 μg/kg (2 samples at 3.4%), respectively. However, no compounds exceeded the MRLs in chicken established by the MFDS (MFDS, 2015).
Antibiotics are essential for treating infectious diseases, and many classes of antibiotics are important for livestock (FAO, 2007; Pagel et al., 2012). Enrofloxacin was detected in seven out of 58 chicken meats (12.1%), and the residue level of ciprofloxacin was 0.28–0.61 μg/kg in chicken. These results are in agreement with previous reports on antibiotic residues that 4.2% of chicken meats in Vietnam were contaminated in enrofloxacin from 2012–2013 (Yamaguchi et al, 2015). Ciprofloxacin residue level in chicken meat ranged from not detected to 0.1 μg/kg in Egypt (Amany et al., 2016). Benzylpenicillin residue level ranged from 0.01 to 1.00 μg/kg in chicken meats. This result is in contrast with a previous report that the residue level of benzylpenicillin was 0.001–0.003 μg/kg in chicken (Lee et al., 2014). The residue levels and detection rates of antibiotics in the current study are considered to be somewhat higher than in previous reports. Enrofloxacin was most used in hospitals and fields related with livestock between the years of 2014 and 2016, followed by ampicillin, sulfamethoxalzole, tetracycline, amoxicillin, tylosin, ceftiofur, penicillin, and sulfamethoxazole (KAHPA, 2018). Amoxicillin, enrofloxacin, and sulfamethoxazole were most detected in chicken meats. These results indicate that antibiotic residues of chicken meats were associated related with antibiotic usage.
In a total of 58 chicken meats, 51 E. coli strains were isolated and identified. All isolates were analyzed to determine resistance patterns to appropriate antimicrobial agents among detected antimicrobial residues based on MIC. As shown in Fig. 1, E. coli isolates from chicken meats showed the highest resistance to ampicillin (75%), followed by tetracycline (69%), ciprofloxacin (65%), trimethoprim/sulfamethoxazole (41%), ceftiofur (22%), and amoxicillin/clavulanic acid (12%).
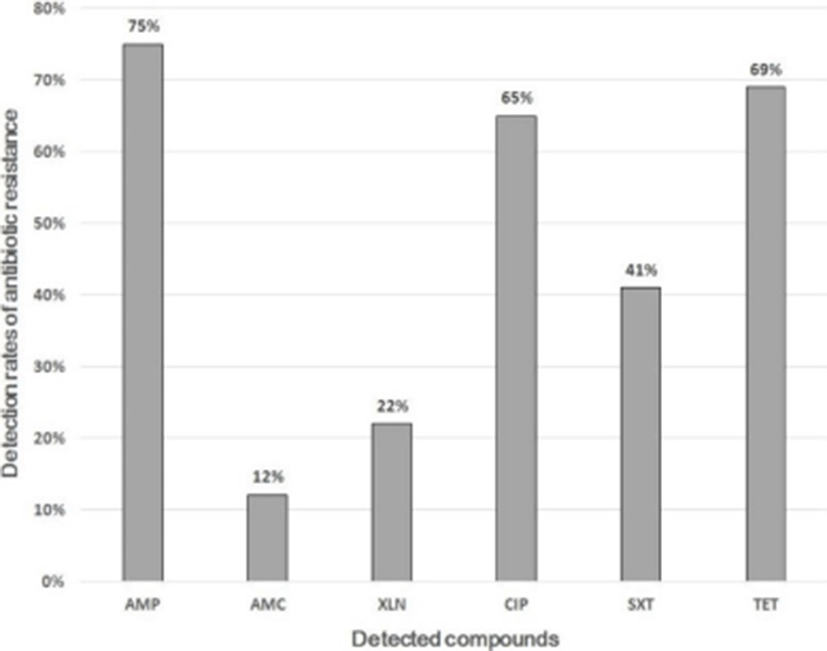
Antimicrobial resistance has increased for decades and now constitutes a threat to the efficient treatment of bacterial, viral, parasitic, and fungal infections (Newell et al., 2010). In addition, increasing antimicrobial resistance contributes to morbidity, mortality, and increased health care costs resulting from treatment failures and longer hospital stays (Cheah et al., 2013). Therefore, public health faces difficulties to treat antimicrobial-resistant bacteria (McGowan, 2001). Studies on bacterial antimicrobial resistance of retail meats are important, as antimicrobial-resistant bacteria could be transferred to the human microbiome through retail meat. E. coli isolated from chicken meat presents the highest resistance to ampicillin (Bortolaia et al., 2010). Resistance to ampicillin is mainly due to β-lactamases such as TEM-1 and SHV-1 enzymes, which hydrolytically cleave the β-lactam ring (Matagne et al., 1998). Plasmid-encoded derivatives of β-lactamases, which show an enhanced spectrum of catalytic activity, have been known since the early 1980s (van den Bogaard et al., 2001). Tetracycline resistance in different bacterial species, both Gram-negative and Gram-positive, is most commonly determined by a large group of efflux proteins, e.g. TetA to TetL belonging to the major facilitator superfamily (MFS) and by another group of proteins, such as TetM to TetW, responsible for actively blocking of the tetracycline target site in the 30S ribosomal subunit (Roberts, 2011). The presence and frequency of tetracycline resistance in E. coli in this study agrees with findings of other studies on antibiotic resistance in E. coli (Sayah et al., 2005). Our results show that antibiotic resistance of the E. coli isolates can be related with antibiotic residues because of usage of antibiotics for animal production.
The detected antibiotics, residue concentrations, and resistance of antibitotics were presented Table 2. Antibiotic residues and resistances were detected in 13 out of 26 samples detected antibiotics in chicken meat (Supplementary Table 3). Our results showed that ciprofloxacin is most detectable compound because enrofloxacin/ciprofloxacin were widely used in livestock farm in Korea (KAHPA, 2018). Our findings suggested that antibiotic residues of chicken meats and antibiotic resistance of isolates from chicken meats were closely related with antibiotic uses.
Our study had several limitations. First, the detection rate and concentration of antibiotics was low to evaluate correlation between residues and resistances, because chicken samples were collected at retail stage after the compliance with withdrawal period. Second, our results have presented limited sample numbers of antibiotic residues and antibiotic resistances. Finally, we have only showed basic information on residues and resistances without the statistical correlations. Nevertheless, our study was the first study to find the relationship between the antibiotic residues and the resistance in chicken meat in Korea. Further studies are needed to assess correlation among the antibiotic residues, the antibiotic resistance from various bacteria, and use amount of antibiotics in animal food production.