Introduction
Various oils are utilized as fat sources in ruminant animal feeds, including palm oil (Choi et al., 2013; Lough et al., 1993; Nestel et al., 1978; Solomon et al., 1992). In the U.S., 33% of feedlot nutritionists use plant oils as feed ingredients (Vasconcelos and Galyean, 2007) and in countries such as Korea in which palm oil is an agri-byproduct, palm oil may be a cost-effective feed additive. Oils derived from plants contain higher concentrations of polyunsaturated fatty acids (PUFA) than animal fats, which contain more saturated fatty acids (SFA). Addition of PUFA to animal feeds may promote fat oxidation, discoloration, and off-odors. These negative effects may be eliminated by using palm oil, which is one of the few highly saturated vegetable oils, containing approximately 40% palmitic acid (16:0) and 40% oleic acid (18:1n-9) (Truswell, 2012).
We recently demonstrated that the saturated fatty acid (SFA) palmitic acid and stearic acid (18:0) strongly promoted CCAAT enhancer binding protein-b (CEBPb) and carnitine-palmitoyl-CoA transferase-1ß (CPT1ß) gene expression in cell cultures of intramuscular (i.m.) preadipocytes (Choi et al., 2015). Therefore, we hypothesized that supplementing the diets of beef cattle with palm oil, rich in palmitic acid, would increase adipogenic gene expression in i.m. adipose tissues.
While this study was being conducted in Korea, a parallel study was completed in the U.S. with Angus cross-bred steers raised to a typical U.S. endpoint (Choi et al., 2013). In that study we demonstrated that, relative to soybean oil, feeding 3% palm oil to cattle increased subcutaneous (s.c.) adipocyte size and the activities of NADP-malate dehydrogenase and glucose-6-phosphate dehydrogenase (Choi et al., 2013). Because of the high concentration of palmitic acid in palm oil, we had predicted that supplementing a beef cattle finishing diet with palm oil would up-regulate SCD gene expression. However, supplemental palm oil, which also is rich in the monounsaturated fatty acid (MUFA) oleic acid, decreased the ratio of palmitoleic acid (16:1n-7) to stearic acid (an index of SCD activity; Choi et al., 2013), and decreased SCD gene expression in s.c. adipose tissue (Choi et al., 2016). In preadipocyte cell cultures, oleic acid and the PUFA linoleic (18:2n-6) and a-linolenic acid (18:3n-3) depressed stearoyl-CoA desaturase (SCD) gene expression, so we attributed the down-regulation of SCD in adipose tissue of palm oil-fed steers to the unsaturated fatty acids in palm oil.
In the current study, we documented the effects of dietary palm oil on specific gene expression in i.m. adipose tissue in Hanwoo steers that were fed a finishing ration for an extended period of time (10 mo). The genes CEBPß, SCD, carnitine palmitoyl transferase-1 (CPT1ß), peroxisome proliferator-activated receptor gamma (PPARg), and AMP-activated protein kinase alpha (AMPKa) were selected as genetic markers of adipose tissue differentiation and metabolism (Jeong et al., 2015). The CEBPß gene product binds to promoter regions of adipogenic genes (Cao et al., 1991), including PPARγ. Similarly, the PPARγ gene product is required for proper adipose tissue development (Tontonoz et al., 1994) and is expressed abundantly in differentiating bovine adipocytes (Grant et al., 2008; Smith et al., 2009). AMPKa activity inactivates energy-consuming processes such as fatty acid biosynthesis, while activating energy-producing processes such as fatty acid oxidation (Hardie, 2007). Because palm oil increases carcass adiposity and lipogenesis in s.c. adipose tissue (Choi et al., 2013), we hypothesized that palm oil would increase the expression of genes associated with adipogenesis (CEBPß, and PPARγ), and decrease SCD and AMPKa gene expression. Because palm oil could potentially increase PUFA content of beef, we also measured meat quality traits.
Materials and Methods
The experimental protocol describing the management and care of animals was reviewed and approved by the Animal Care and Use at the National Institute of Animal Science (NIAS). The control diet was formulated to meet the nutrient requirements recommended by the Korean Feeding Standard for Hanwoo (NIAS, 2007). Fourteen Hanwoo steers (average BW 383.5±7.3) were assigned randomly to either a control diet (Table 1) or, the basal dist supplemented with 5% palm oil (BDSP). Each treatment group was placed in a 7.0 × 10.0 m pen and fed its respective diet twice per day with an individual feeding stanchion. Steers were adapted to a corn-based finishing diet for 14 d and then fed either the control diet or BDSP. The fatty acid composition of palm oil was 43.6% palmitic acid, 4.6% stearic acid, 37.1% oleic acid, 10.4% linoleic acid and 0.2% α-linolenic acid. All animals had free access to water. Feed intake was recorded daily and animals were weighed monthly. Steers were fed the experimental diets for 10 mon until they attained an average BW of 753 ± 11.5 kg.
1Vitamin A, 2,650,000 IU/kg; Vitamin D, 3,530,000 IU/kg; Vitamin E, 1,050 IU/kg; Niacin, 10,000 mg/kg; Mn, 4,400 mg/kg; Zn, 4,400 mg/kg; Fe, 13,200 mg/kg; Cu, 2,200 mg/kg; I, 440 mg/kg; Co, 440 mg/kg; Butylated hydroxy toluene, 10,000 mg/kg. 2Crude Protein. 3Neutral Detergent Fiber. 4Total Digestible Nutrients. 5Net Energy, Carcass Weight.
Steers were fasted for 24 h and slaughtered at a commercial abattoir. Intramuscular adipose tissues were obtained from the 8th to 11th rib section as described previously (Choi et al., 2016) and immediately snap frozen in liquid nitrogen. Carcass yield and quality including marbling score were graded by meat graders using criteria provided by Livestock Quality Assessment (KAPE, 2007). Meat color attributes Hunter L (lightness), a (redness) and b (yellowness) and CIE L*a*b* were determined by Chroma Meter (CR-10, Minolta Corporation, Ltd., Japan). Each sample was measured at 4 different locations on the muscle surface and an average score was taken.
Real-time PCR was conducted as previously described (Park et al., 2009). Briefly, mRNA from i.m. adipose tissue was isolated using TRIzol reagent (Invitrogen, USA) and cDNA was made using the random primed cDNA synthesis. Real-time quantification of AMPKα, CTP1β, C/EBPβ, PPARγ, and SCD transcripts was performed with the 7500 Fast Real-Time PCR System (Applied Biosystems, USA). Final reaction mix included 1X Fast SYBR Green Master Mix (Applied Biosystems, USA). The relative amount of mRNA was first normalized to ribosomal protein S9 (RPS9) and then expression of each transcript from palm oil group was normalized with that from the control group. Target primer sets designed using Primer-BLAST (National Center for Biotechnology Information) for these genes were: forward 5’-ACC ATT CTT GGT TGC TGA AAC TG-3’ and reverse 5’- CAC CTT GGT GTT TGG ATT TCT G-3’ for AMPKα; forward 5’-TGG CTC TGG ATG AGG AGT CT-3’ and reverse 5’-GGA CTT GTC GAA CCA CCT GT-3’ for CPT1β; forward 5’-CCA GAA GAA GGT GGA GCA ACT G-3’ and reverse 5’-TCG GGC AGC GTC TTG AAC-3’ for C/EBPβ; forward 5’-ATC TGC TGC AAG CCT TGG A-3’ and reverse 5’-TGG AGC AGC TTG GCA AAG A-3’ for PPARγ; forward 5’-TGC CCA CCA CAA GTT TTC AG-3’ and reverse 5’-GCC AAC CCA CGT GAG AGA AG-3’ for SCD; and forward 5’-GAG CTG GGT TTG TCG CAA AA-3’ and reverse 5’-GGT CGA GGC GGG ACT TCT-3’ for RPS9. Relative standard curves containing 0.2-200 ng of cDNA were used to determine primer efficiency, which ranged from 97 to 106%.
Approximately 100 mg of intramuscular adipose tissue was isolated from loin muscle at slaughter. Total lipids were extracted by the method (Folch et al., 1957). Adipose tissue lipids were extracted in chloroform:methanol (2:1, vol/vol) and fatty acid methyl esters (FAME) were analyzed by gas chromatography according to 2013 (Choi et al., 2013). FAME were analyzed by GC-FID (model CP-3800 equipped with a CP-8200 auto-sampler, Varian Inc, USA). Separation of FAME was accomplished on a fused silica capillary column (100 m × 0.25 mm ID; model CP-3800, Varian Inc, USA) with the helium as carrier gas (flow rate = 1.7 mL/min). One microliter of sample was injected with the split ratio of 100:1 at 270°C. Oven temperature was set at 165°C for 65 min and then increased to 235°C (2°C/min) and held for 15 min. Flame ionization detector (FID) detected the signal at 270°C. An authentic standard (GLC 68D, Nu-chek Prep, USA) was used to confirm the identity of each peak.
Results
There was no significant difference in growth performance including BW and average daily gain (ADG) between treatment groups (Table 2). Carcass quality traits also were unaffected by supplemental of palm oil. Supplemental palm oil also did not affect meat color attributes (Table 3). There was no difference in feed intake for both control and palm oil groups (data not shown).
1Control feed was formulated based on Korean Feeding Standard for Hanwoo. 2Pooled standard error of the mean over all samples (n= 14). 39 = most abundant, 1 = devoid.
1Standard error of the mean
Supplemental palm oil increased (p<0.05) AMPKα and CPT1β gene expression in i.m. adipose tissue relative to control i.m. adipose tissue (Fig. 1). C/EBPβ expression was lower and PPARγ expression was higher (both p<0.05) in i.m. adipose tissue of palm oil-fed steers than in i.m. adipose tissue of control steers. SCD gene expression was not affected by palm oil supplementation (p>0.05).
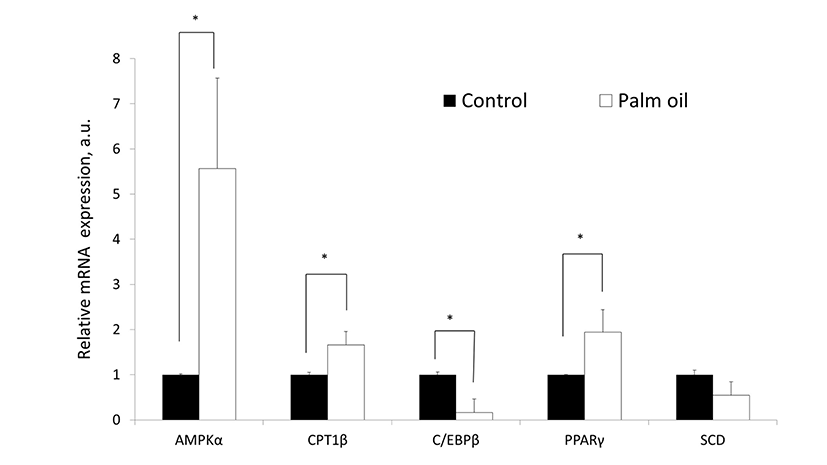
Myristic acid (14:0), palmitic acid, stearic acid, oleic acid, cis-vaccenic acid (18:1n-7), linoleic acid, γ-linoleic acid (18:3n-6), and α-linolenic acid were unaffected by supplemental palm oil (Table 4; p>0.05). There also was no difference in eicosenoic acid (20:1n-9) or arachidonic acid (20:4n-6) between treatments. The proportion of total PUFA was higher (p<0.05) in i.m. adipose tissue of palm oil-fed steers than in control adipose tissue. Intramuscular adipose tissue of palm oil-fed steers had greater (p<0.05) levels of ω3 fatty acids but lower (p<0.05) ω6:ω3 ratio than control group.
1Control feed was formulated based on Korean Feeding Standard for Hanwoo. 2Pooled standard error of the mean (n=14). *p<0.05, significantly different from control.
Discussion
The production of Hanwoo beef cattle differs from beef cattle production in the U.S. in that Hanwoo cattle are fed to heavier BW (approximately 750 kg vs 550 kg), and energy density of the diet is increased in stages in the Korean beef cattle production system to ensure adequate i.m. fat accumulation. However, the long-term feeding of Hanwoo beef cattle results in poorer feed efficiencies and increased feed costs. It is possible that agri-byproducts such as palm oil could reduce feed costs for the Hanwoo beef cattle industry, but there is little information available about the effects of palm oil on growth, carcass composition, and meat composition of beef cattle.
Because it is readily available, soybean oil has been tested extensively in beef cattle production systems. Engle et al. (2000) reported that 4% supplementary soybean oil had no effect on average daily gain (ADG), average daily feed intake (ADFI), or gain-to-feed ratio (G:F), but depressed marbling scores. Ludden et al. (2009) later reported that 5% supplemental soybean oil had no effect on production or carcass traits of Gelbvieh x Angus crossbred steers. In a study with Korean Hanwoo cattle, Song et al. (2010) reported that 7% supplemental soybean oil (DM basis) depressed feed intake, ADG, and G:F, and we subsequently reported that 3% soybean oil depressed ADG, and the G:F in Angus crossbred steers (Choi et al., 2013). It is likely that the high concentration of PUFA in soybean oil depresses ruminal fermentation, reducing its efficacy as a feed supplement for beef cattle.
Previous studies with lambs demonstrated that supplemental palm oil increased backfat thickness (Lough et al., 1993; Solomon et al., 1992). Relative to soybean oil-fed steers, palm oil-fed Angus crossbred steers had greater marbling scores and slightly greater 12th rib fat thickness (Choi et al., 2013). Supplemental palm oil promoted lipid synthesis in vitro and increased lipogenic enzyme activities and adipocyte sizes in s.c. adipose tissue (Choi et al., 2013; Nestel et al., 1978). In the current study, palm oil supplementation had no effect on carcass quality traits or feed efficiency of Hanwoo steers, nor did it affect meat color attributes.
AMPKα, C/EBPß, CPT1ß, PPARg, and SCD were selected as genetic markers for differentiation and metabolism of adipose tissue based on previous research (Smith et al., 2012). As in our study with Angus crossbred cattle (Choi et al., 2016), supplemental palm oil decreased C/EBPß gene expression and caused a small decrease in SCD gene expression. The marked increase in AMPKα gene expression in i.m. adipose tissue in response to supplemental palm oil is of interest because AMPK regulates many metabolic processes including glucose uptake, fatty acid synthesis, and lipid oxidation (Park et al., 2009). AMPK activity decreases malonyl-CoA concentrations by phosphorylating and thereby inhibiting acetyl CoA carboxylase, the rate-limiting enzyme of malonyl-CoA synthesis, and by enhancing the degradation of malonyl-CoA via malonyl CoA decarboxylase (Thomson and Winder, 2009). Malonyl-CoA is an allosteric inhibitor of CPT1, the enzyme that controls the transfer of long chain fatty acyl-CoA into the mitochondria. The markedly increased AMPKα and CPT1ß gene expression caused by palm oil supplementation should have promoted fatty acid oxidation, but there was only a small, nonsignificant reduction in back-fat thickness in the palm oil-fed steers relative to the control steers. We recently demonstrated that SFA strongly up-regulated AMPKα gene expression in cultures of i.m. preadipocytes and 3% supplemental palm oil increased plasma palmitic acid over 40% in beef cattle (Choi et al., 2016). However, unlike the results of the current study, supplemental palm oil decreased AMPKα gene expression in s.c. adipose tissue of Angus crossbred steers relative to control steers (Choi et al., 2016), which was consistent with the small increases in 12th rib fat thickness and marbling scores observed in these cattle (Choi et al., 2013).
In the current study, C/EBPβ gene expression was decreased but PPARγ gene expression was increased in i.m. adipose tissue by palm oil. The lack of significant effects of palm oil on carcass adiposity indicated that 5% supplementary palm oil administration can alter adipogenic gene expression, but not sufficiently to elicit changes in adipose tissue accretion. This is in spite of the fact that the Hanwoo steers were fed supplemental palm oil for 10 mo.
Palm oil supplementation caused only a small decrease in SCD gene expression in the current study, but this was of similar magnitude to the depression in SCD gene expression in s.c. adipose tissue caused by palm oil in Angus crossbred cattle (approximately 25%; Choi et al., 2016). Similarly, supplemental palm oil decreased the SCD index (ratio of palmitoleic acid:stearic acid) from 0.49 to 0.32 in the previous study (Choi et al., 2016) and from 0.39 to 0.34 in the current study. Adipose tissue is the primary site for SCD activity (St John et al., 1991) and SCD gene expression increases with time on a finishing diet (Adams et al., 2010). Rams fed a diet containing palm oil had significantly more SFA in their i.m. lipid compared to control lambs, mainly due to the high concentration of palmitic acid in palm oil (Solomon et al., 1992). However, the previous and the current study have demonstrated that palm oil has little effect on the proportions of SFA in bovine adipose tissue (Partida et al., 2007). However, palm oil supplementation to Hanwoo steers increased i.m. adipose tissue PUFA, which might be responsible for the small depression is SCD gene expression.
Conclusions
It is not possible to make direct comparisons of the current study to our previous studies of the effects of palm oil on gene expression in adipose tissue of Korean Hanwoo and U.S. beef cattle. In previous studies (Choi et al., 2013; Choi et al., 2016), Angus crossbred steers were fed 3% palm oil for 10 wk, rather than 10 mo, and the Angus crossbred steers weighed approximately 570 kg at slaughter, rather than 750 kg for the Hanwoo steers. Adipose tissue MUFA are higher in Hanwoo steers than in U.S. domestic cattle (Smith et al., 2009), and adipose tissue MUFA increase with time on a finishing diet in beef cattle (Chung et al., 2006; Kim et al., 2016). For these reasons, i.m. adipose tissue from Hanwoo steers of the current study contained over 55 g MUFA per 100 g total fatty acids, versus 40 g MUFA per 100 g total fatty acids in i.m. adipose tissue of Angus crossbred steers (Choi et al., 2016). Similarly, it has not been possible to modify carcass composition with supplemental palm oil, even though this and our previous studies documented significant changes in adipogenic gene expression. It is likely that other factors, such as time on feed and other dietary components, attenuated any effects on carcass adiposity suggested by the observed changes in adipose tissue gene expression. We can conclude, however, that palm oil, an agri-byproduct in Korea, can be used as a feed supplement without negative effects on Hanwoo beef cattle production or carcass characteristics.