Introduction
There is a need for both alternative and traditional meat production to fulfill the increasing demand of increasing population by the year 2050 (FAO, 2017). Examples of meat alternatives are cultured meat (CM), made utilizing stem cells to produce basic muscle proteins like actin, myosin, and troponin, in other words, myofibrillar proteins (MFPs; Post et al., 2012); hybrid meat (HBM) by replacing a portion of meat (Alam et al., 2024c), hybrid cultured meat (HCM; Alam et al., 2024b); plant-based meat analogs (Kumari et al., 2023); insect-based meat (Van der Weele et al., 2019); three dimensional printed meat with scaffolds (Alam et al., 2024a) etc. Among these alternatives, CM and HBM stand as a strong candidate to capture the global alternative meat market alongside traditional meat. Although CM research and development has spread globally through approximately 100 commercial organizations (Behera and Adhikary, 2023), the market still has a fairly restricted presence of CM products due to limited cm scale production in the laboratory.
Nevertheless, more intensive product development strategies need to be practiced to bring variation in CM products to attract consumer acceptance. As CM production is still negligible at the cm/gm scale, so, it is important to imitate CM with a similar kind of muscle till scalable production starts, with a focus on understanding the probable physicochemical characteristics of future CM products. As CM is mainly (75%–90%) composed of MFPs (Ben-Arye and Levenberg, 2019; Post et al., 2012). Thus MFP can be easily pretended as an imitation of CM for product development purposes to understand and modify future CM product characteristics in advance.
Improvement of HBM/HCM can be achieved by adding flavor enhancers, colorants, plant proteins, etc. Products derived from postmortem wet and dry aging can enhance the tenderness and flavor of meat, hence boosting palatability and increasing consumer preferences (Kim et al., 2022; Tuell et al., 2022). Enhancing the umami flavor and taste (Hossain et al., 2024) could be a unique value addition to the HBM/HCM for increasing the consumer base which can be achieved by adding dry aged beef crust (Ribeiro et al., 2024), 35% of which is discarded (Lee et al., 2019). Meat crusts have proven to be efficient functional components in ground beef products (Jeong and Kim, 2016).
Research must be conducted to imitate CM to understand the quality characteristics of future products and improvisation techniques. Thus the objective of this study was to make an imitated CM to produce imitated hybrid cultured meat patty (IHCMP) to assess the probable quality parameters in advance, compare characteristics between IHCMP, beef meat patty (BMP), and plant-based meat patty (PBMP), and incorporate dry-aged beef crust to improve the physicochemical and sensory quality of IHCMP in comparison to BMP.
Materials and Methods
The MFP extraction process is illustrated in Fig. 1. The frozen hanwoo meat sample was defrosted for approximately 12 hours at a temperature of 2°C. The hanwoo meat myofibrils were then prepared using a modified process based on previous findings (Chen et al., 2016). Visible connective tissue fat was removed from the muscles and grounded by a 3 mm fine grinding plate and 80 rpm applying an electronic mincer (KitchenAid, St. Joseph, MI, USA). The minced meat weighing 3.0±0.05 g, underwent four rounds of rinsing using cold deionized, distilled water at a temperature of 4°C. During each washing phase, the minced meat and water were mixed at a ratio of 1:10 (w/v). After an initial homogenization using the IKA T25 ULTRA-TURAX (IKA-Werke, Staufen, Germany) for 2 minutes, the homogenate was left to rest for 10 minutes. The sediment from each stage was subsequently gathered using centrifuging at a velocity of 18,000×g for 20 minutes, while maintaining a temperature of 4°C. During the third round of the washing stage, the suspension before centrifuge was strained through cheesecloths (grade #90 having 44×36 threads per square inch, Junggeun Medical Supplies, Siheung, Korea) to eliminate the connective tissue and lipid. Following the completion of the final process of washing and centrifugation MFP was collected as sediment. MFP was vacuum packed and sterilized at 100°C in a water bath for 30 minutes before further use.
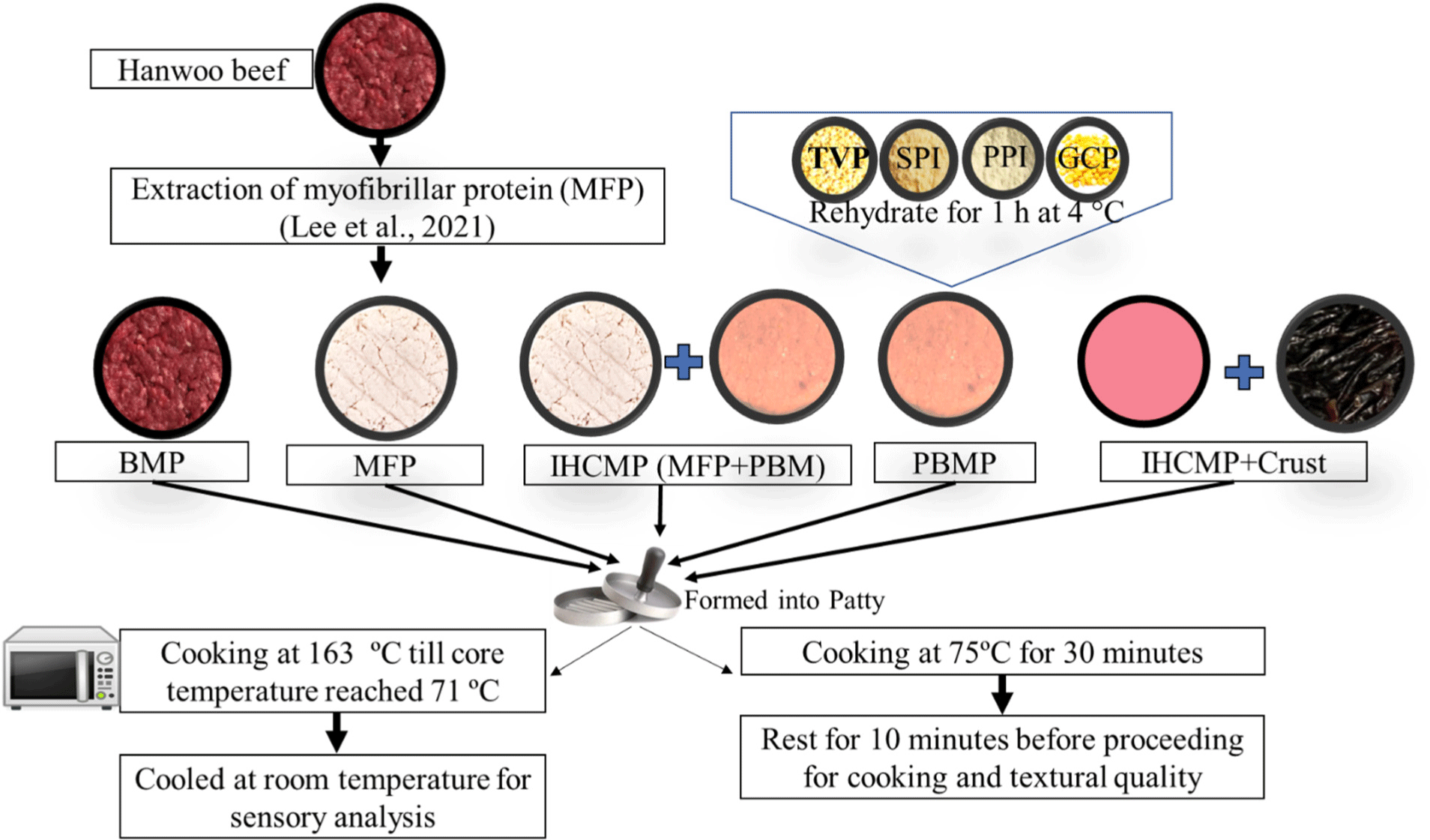
BMP (control), MFP, IHCMP, PBMP, and IHCMPCR (IHCMP with dry-aged beef crust) were produced as per the following formulation in Table 1.
The dry-aged beef crust was collected from a local beef processing company in the Republic of Korea. The vacuum-packed beef crust was stored at –20°C until incorporation in the IHCMP. TVP, PPI, and SPI (Nutricost, Vineyard, UT, USA) were used as PBMP/IHCMP base, methylcellulose (Pure, Santa Clara, CA, USA) was added as a binding agent and red beetroot powder (Charm Goods, Seongnam, Korea) used as a color enhancer. Other ingredients were used as described in Table 1.
The flow diagram for processing control and treatment patties is illustrated in Fig. 1. For the production of PBMP, TVP, SPI, and PPI were separately mixed with double distilled water (1:2) and allowed to hydrate at 4°C for 2 hours. Hydrated TVP, SPI, PPI, and CPP were blended with the materials indicated in Table 1 using a food mixer (KitchenAid) and then formed into 50 g patty using a patty press in triplicate. For the preparation of PBMP and IHCMP, all ingredients as per Table 1 were mixed in a Kitchen Aid mixer and formed into a 50 g patty in triplicate. MFP was mixed thoroughly with the ingredients listed in Table 1 following the above procedure to form a 50 g patty in triplicate. Hanwoo bicep femoris steak was collected from a hypermarket in Jinju, Republic of Korea. After removing extra fat and connective tissue beef was ground and mixed with the ingredients mentioned in Table 1 using the Kitchen Aid mixer. After proper mixing, a 50 g patty was formed in triplicate. For IHCMPCR, the same IHCMP process was followed but replaced 15% MFP with beef crust. All the patty was formed in a round shape of approximately 6 cm and a height of 2 cm.
The moisture content was assessed according to the AOAC (2002) standard. A sample weighing around 2±0.05 g sample was subjected to dehydration on an aluminum dish at 105°C for 16 hours using a scientific oven (BF-150C, BioFree, Buchen, Korea). The sample was then transferred to a desiccator, and the moisture content was estimated using the provided formula.
The color attributes of the patties were analyzed in triplicate by applying a colorimeter (Konica Minolta CR-300, Konica Minolta, Osaka, Japan). Before measuring CIE L*, CIE a*, and CIE b* at three separate regions of the samples, the colorimeter underwent calibration using a white plate yielding the following values: Y=93.5, X=0.3132, y=0.3198.
The pH of the patties was measured using a digital pH meter (Orion Star™ A211 Benchtop pH Meter, Thermo Fisher Scientific, Waltham, MA, USA). Before data collection, the probe underwent calibration at a temperature of 25°C using calibration solutions with pH values of 7.00, 4.01, and 9.99. Approximately 3±0.05 g of samples were combined with 27 mL of double distilled water and subsequently homogenized using an IKA T25 Ultra-Turax homogenizer (made by IKA-Werke) for 30 minutes.
Releasing water content (RW%) was determined in triplicate for the samples using the approach outlined by Joo (2018). Around 3±0.05 g of samples were deposited onto a Whatman filter paper measuring 10 cm in diameter and subsequently overlaid with two thin plastic film sheets. The patty samples were sandwiched between Plexiglass plates, together with filter paper and plastic film sheet, and put under an even pressure of 2.5 kg for 5 minutes to aid in the release of water. Ultimately, the RW was computed using the following formula:
The cooking loss (CL) of the samples has been evaluated in triplicate by computing the proportion of diminished weight. The experiment entailed placing a patty weighing around 25±0.05 g and having approximately 1.5 cm in depth, in a polypropylene bag. The samples were cooked in a hot water bath at 75°C for 30 min. Immediately, the samples were cooled at room temperature for 30 min to remove additional surface moisture. The calculation was executed on the subsequent formula:
The shrinking of the samples was assessed by measuring the alterations in diameter and thickness immediately before and following the cooking process. To ascertain the percentage of shrinkage in the patties, arbitrary observations were taken at four different points on both the raw and cooked samples as documented by Samard et al. (2021). The measurements were performed in triplicate. The percentage of shrinkage was determined using the following formula:
Right after the assessment of CL, samples went through Warner-Bratzler shear force (WBSF) testing to measure the shear force of the cooked samples. The testing was conducted using the established protocol outlined by the American Meat Science Association (AMSA, 1995).
Texture profile analysis (TPA) of the patties was assessed using a Sun Rheometer (Compact-100 II, Sun Scientific, Tokyo, Japan). The patty samples were produced with a dimension of 4 cm in length, 4 cm in width, and 1.5 cm in depth. They were then cooked at 75°C for 30 minutes in a preheated water bath. After cooking the samples were kept for cooling for 30 minutes. For analyzing TPA patty samples were compressed using a flat pressure adaptor (No.1) in an axial orientation rheometer. The patty samples were compressed using a two-cycle arrangement in consecutive order at a crosshead speed of 60 mm/min and a final strain of 60% while a 10 kg load was applied. The following characteristics of the texture were analyzed: cohesion, hardness, chewiness, gumminess, and springiness.
The samples’ crude fat (CF) content was determined using a modified method derived from the procedure outlined by Folch et al. (1957). To extract CF about, 3±0.05 g of patty sample was blended treating with 30 mL of Folch solution (chloroform: methanol=2: 1, v/v) in a homogenizer. The homogenate was then filtered using Whatman no.1 filter paper). Subsequently, 8 mL of NaCl solution with a concentration of 0.88% was added to the filtrate and well-blended using a vortex. After a 12-hour resting time, the top layer was extracted with a glass pipette using an aspirator. The lowermost layer was transferred to an aluminum dish that had been pre-weighed, to undergo the process of dehydration at 50°C in a hot air oven. The CF content was computed with the following formula.
Following the extraction of CF the aluminum dishes were heated at at 90°C on a heat block and then 1 mL Dichloromethane was added to the dish to dilute by rotating 5 turns on the palm. Immediately 1 mL supernatant was taken into the glass tube. Then 1 mL methanolic NaOH was added to the glass tube and heated for 10 minutes and after cooling 1 mL Boron trifluoride (BF3) and again heated for 10 minutes. Following cooling 3 mL hexane and 8 mL deionized water were added and vortexed till properly mixed. This solution was then centrifuged at 1,000×g for 15 minutes and a small quantity of Na2SO4 was added to dehydrate extra moisture for 1 minute. Then 2.5 mL of resultant fatty acid methyl ester (FAME) was separated in a glass tube and from there 1.5 mL was taken in a gas chromatograph (GC) tube for fatty acid analysis. To analyze FAME a GC HP6890N fitted with an automatic sampler arm HP7683 (Hewlett-Packard, Santa Clara, CA, USA) was utilized. A 100-m SP2560 (Supelco, Santa Clara, CA, USA) equipped with a capillary column (0.25 mm internal diameter and 0.20 μm film thickness) was used to estimate the FAME extractions. FAME from the patties was refined using a temperature catalog with nitrogen serving as the carrier gas at a flow rate of 1mL/min. A column oven temperature control was installed in the chromatograph, and it was programmed to raise the temperature over time. The GC process involved a sequence of reactions conducted at specific temperature intervals: 50°C–180°C with a heating rate of 10°C/min, 180°C–220°C at 5°C/min, and 220°C–240°C at 2°C/min. The process was then maintained at a constant temperature of 240°C for 20 minutes. The GC-equipped automatic sampler arm was utilized to perform eleven injections of 1 μL each. An estimation was made of the retention periods of fatty acid standards (Supelco 37 Components FAME Mix, Supelco). Ultimately, the FAME results were calculated as a percentage proportion of the overall fatty acids in the total peak area for corresponding fatty acids. The analysis was conducted in duplicates. The results were quantified as a percentage of the total fatty acid identified based on the total peak area. Atherogenic indexes were calculated by dividing the sum of saturated fatty acid (SFA) by the sum of unsaturated fatty acid [monounsaturated fatty acid (MUFA)+polyunsaturated fatty acid (PUFA)], using the formula developed by Ulbricht and Southgate (1991): AI (atherogenic index) = [C12:0 + 4(C14:0) + C16:0] / [MUFA + PUFA].
The visual attributes of the samples were acquired through the use of a digital camera (Nikon D7000, Nikon, Tokyo, Japan) and the variation in texture was observed and recorded.
The patty was fried in a preheated oven at a temperature of 163°C till the internal temperature reached 71°C according to the method described by AMSA (1995). The patty temperature was assessed using a digital thermometer. The samples were cooled to ambient temperature before conducting the sensory analysis. A group of eight skilled sensory analysts in the meat science laboratory at Gyeongsang National University assessed samples from each treatment. The panel assessed these samples based on their tenderness, juiciness, flavor, and overall acceptability using a 9-point hedonic scale (0 poor and 9 excellent). Water was provided to the panel to wash the mouth between samples. The approved consent procedure for sensory evaluation is Institutional Review Board (IRB): GIRB-E24-NY-0116.
An electronic tongue system (ETS; INSERT SA402B Electric Sensing System, Insent, Tokyo, Japan) was used to undertake the examination of the samples, implementing the technique exemplified by Ismail et al. (2020). The ETS system is primarily composed of sensor arrays, electrodes, a data analysis program, and specialized artificial lipid membranes. The ETS was employed to ascertain the attributes of sourness, bitterness, umami, and richness. The examination of each measured parameter was conducted once all membranes were stabilized in a standard meat taste (SMT) solution. The SMT solution was comprised of 0.01% lactic acid (sourness), 0.25% monosodium glutamate (umami), and 0.0005% quinine hydrochloride (bitterness). Approximately, 100±0.05 g of ground sample was mixed with 400 mL of hot double distilled water (95°C; 20 min). The blended solution was centrifuged for 15 min at 1,000×g, and the supernate was stored at –70°C for further analysis.
Statistical analysis of the revealed data was analyzed using analysis of variance for all variables using SAS software (version 9.4, SAS Institute, Cary, NC, USA), followed by Duncan’s multiple range test for multiple mean comparisons. The error terms used throughout this study are SE. Results are expressed as least square mean values of three independent replications, except for WBSF, TPA, and sensory test for which the average of five measurements was obtained using repeated measurements. A p-value≤5% was considered significant. The data for principal component analysis (PCA) was organized by placing the treatments in rows and all variables in separate columns using Metaboanalyst 6.0 software. The score plot demonstrated the distinction between the principal components based on the treatment effect. The biplot displayed the correlation between individual concentration correlation and their normal distribution, confirming their suitability for PCA analysis.
Results and Discussion
The physicochemical properties of control and treatment are shown in Table 2. Higher moisture content (p<0.05) was observed in the BMP and PBMP followed by IHCMCR, IHCMP, and MFP. High moisture in PBM is due to the absorption of excess water during the rehydration process in TVP, PPI, SPI, and methylcellulose exhibits a greater water retention capacity in comparison to meat (Amboon et al., 2012). RW was lowest (p<0.05) in IHCMPCR and BMP followed by MFP. Both IHCMP and PBMP had higher RW values. The low RW in meat samples is attributed to stronger bonds between protein molecules and water molecules (Yang, 2018). The low relative RW in IHCMPCR may attributed to the high rehydration potential, which enhances the affinity with water molecules in the patties (Park et al., 2020). This trait is typical of additives that have a high rehydratability.
Meat pH in raw BMP and IHCMPCR was lower (p<0.05) and MFP, and IHCMP had slightly higher pH values and PBMP showed a basic pH. These values increased after cooking in BMP, IHCMPC, IHCMP, and PBMP, except MFP which was reduced. Previous investigations have indicated meat pH rises when it is subjected to the external environment during heat-induced protein denaturation (Forrest et al., 1975; Lee et al., 2022). The higher pH seen in the PBMP and IHCMP is likely a result of the increased alkalinity and variety of the plant protein utilized in the formulation (De Marchi et al., 2021). These results are in line with those reported in a recent study by Kamani et al. (2019) comparing chicken sausages to plant-based sausages made from soy protein isolate (De Marchi et al., 2021). The patty samples were darker and reddish (p<0.05) in BMP followed by IHCMPCR and IHCMP. MFP and PBMP were lighter and lacked in CIE a*. The CIE b* was higher in the PBMP. After cooking all the samples turn darker but a similar value trend is sustained. CIE L* and CIE b* decreased as CIE a* increased in beef and IHCMPCR because of the rise in the myoglobin concentration (the red-colored component) in meat products (Kim et al., 2019). The CF was higher (p<0.05) in IHCMPCR and BMP than in other samples. The crust of Hanwoo beef has high fat content (Lee et al., 2022), therefore, there is a prevailing belief that a significant amount of fat is present in IHCMPCR can be obtained via the addition of crust. Huber et al. (2016) found that pea protein has a limited ability to retain fat, likely because it contains a resistant starch portion and it correlates with the present study where PBMP and IHCMP had lower CF levels as well. This observation was supported by Park et al. (2023) on beef patty produced with mushrooms and plant protein. Our previous study on hybrid pork steaks also revealed higher pH, CIE L*, and CIE b* in samples formulated with plant ingredients (Alam et al., 2024c).
The CL of meat products is influenced by the degree of cross-linking between salt-soluble proteins, water molecules, and fat globules (Park, 2011), which are determined by the structure, quantity, protein content, and physical qualities of meat (Mittal and Usborne, 1985). Cooking qualities are important parameters for meat and related products and CL values in the present study are illustrated in Table 3. The CL was elevated (p<0.05) in BMP followed by lower values in PBMP, IHCMPCR, IHCMP, and MFP respectively. Diameter reduction was higher (p<0.05) in BMP and IHCMP, whereas thickness reduced mostly in BMP, MFP, and PBMP. The low CL of MFP is attributed to stronger bonds among protein molecules and water molecules (Yang, 2018). Reduced CL in IHCMPCR may be due to high rehydration potential which strengthens the bond with water molecules in the patties which is characteristic of highly rehydratable additives (Park et al., 2020). The diameter and thickness reduction ratios of the patties are shown in Table 3. The lowest (p<0.05) diameter and thickness reduction were observed in IHCMPCR and in other samples had variable shrinkage. These results were consistent with previous findings that showed that non-meat proteins, such as wheat germ and soy proteins, added to patties resulted in lower CL and shrinkage (Brown and Zayas, 1990). IHCMP and PBMP in this had smaller shrinkage, which is supported by Choi et al. (2015), where pork patties prepared with isolated soy protein increased the bond strength between water and fat, thus minimizing CL and shrinkage. Nevertheless, the addition of crust in IHCMPCR improved water retention in patties and cooking-related properties.
WBSF share force value revealed harder texture (p<0.05) in BMP, IHCMPCR, and IHCMP with a much softer trend in PBMP. MFP protein in meat products undergoes gelation when heated and helps in consistent texture and water retention (Guo et al., 2019; Xia et al., 2018).
The TPA values are illustrated in Fig. 2. The analysis revealed a harder (p<0.05) BMP followed by IHCMPCR and MFP and softer in PBMP and HCM (Fig. 2A). Gumminess values (Fig. 2B) were higher (p<0.05) in BMP followed by IHCMPCR, MFP, and IHCMP. In overall observation, PBMP demonstrated a softer textural pattern among all the samples. Chewiness was better in BMP and IHCMPCR followed by IHCMP and MFP patties. Chewiness (Fig. 2D) was the least in PBMP. Springiness (Fig. 2E) was higher in BMP followed by IHCMPCR and lowest was in PBMP followed by IHCMP and MFP. Typically, a beef patty has a higher hardness score because of the inherent strength of muscle tissue. Our previous study confirmed that PBM had lower hardness than meat-based steaks (Alam et al., 2024c). A similar characteristic was observed in this study whereby the beef patty had greater hardness than PBM. Kamani et al. (2019) conducted a thorough investigation of the concept of hardness. According to their findings, sausages with more meat necessitate a greater amount of grinding force compared to sausages without meat. Furthermore, it has been proposed that the increased hardness of animal-based patties is a result of the denaturation of muscle proteins, leading to the meat becoming harder (Sakai et al., 2022). Therefore, the decreased hardness and chewiness measurements of the IHCMP and PBMP could be attributed to a less robust MFP network (Alam et al., 2023), thus the product has lower comprehensive resistance compared to BMP, MFP, and IHCMPCR. Similarly, a trend of noticeable decline was observed in the springiness, cohesiveness, and gumminess attributes of IHCMP and PBMP compared to BMP, IHCMPCR, and MFP. The present study confirms the findings of prior research conducted by Chiang et al. (2021) on meat substitutes including soy protein and wheat gluten. The study showed that these substitutes had reduced levels of chewiness, cohesiveness, and springiness compared to steam cooked chicken meat.
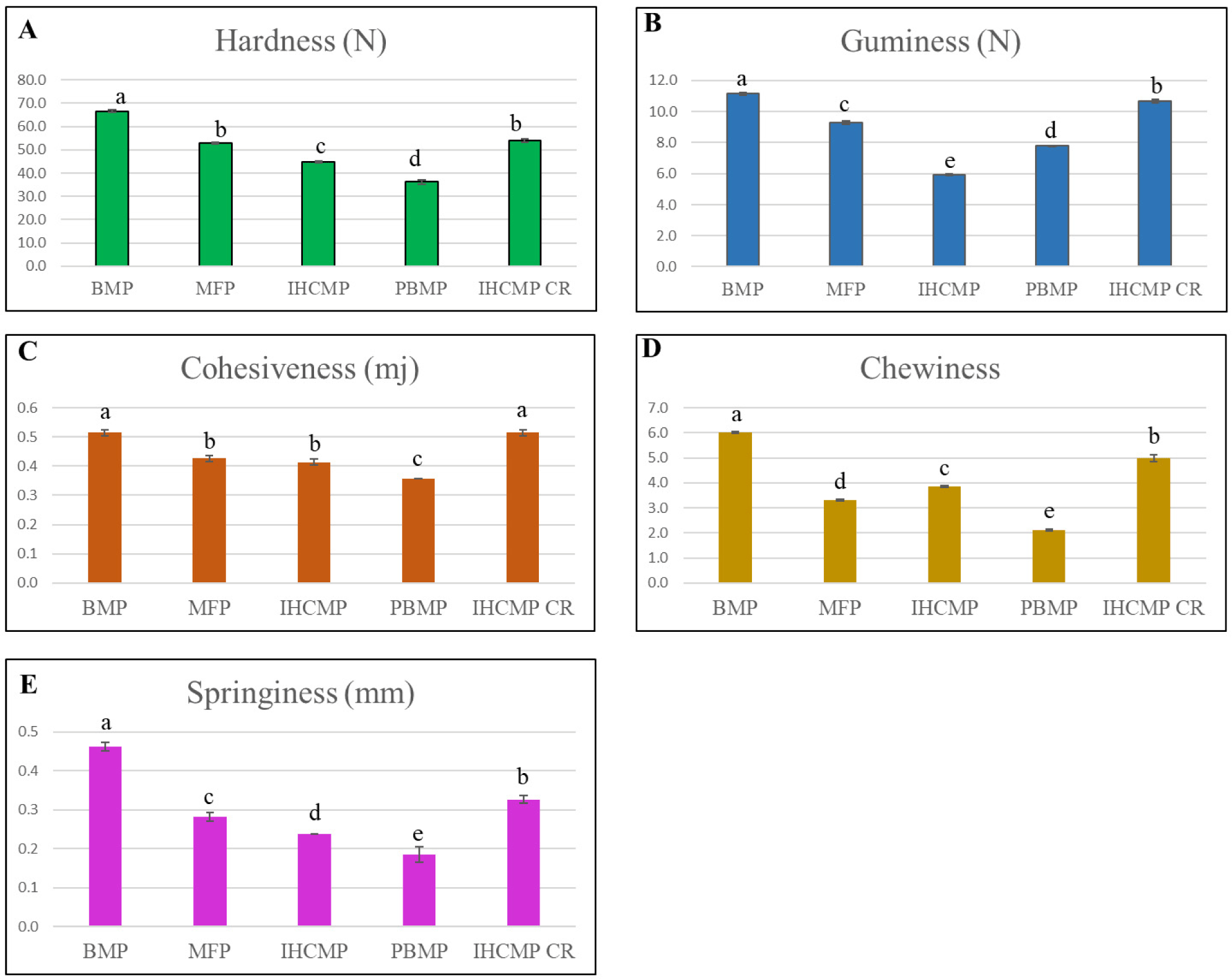
The physical appearance of the patties is shown in Fig. 3. The raw, cooked, and cross sample of the cooked samples illustrates the difference among the samples. The visual color and texture characteristics are visible in the images.
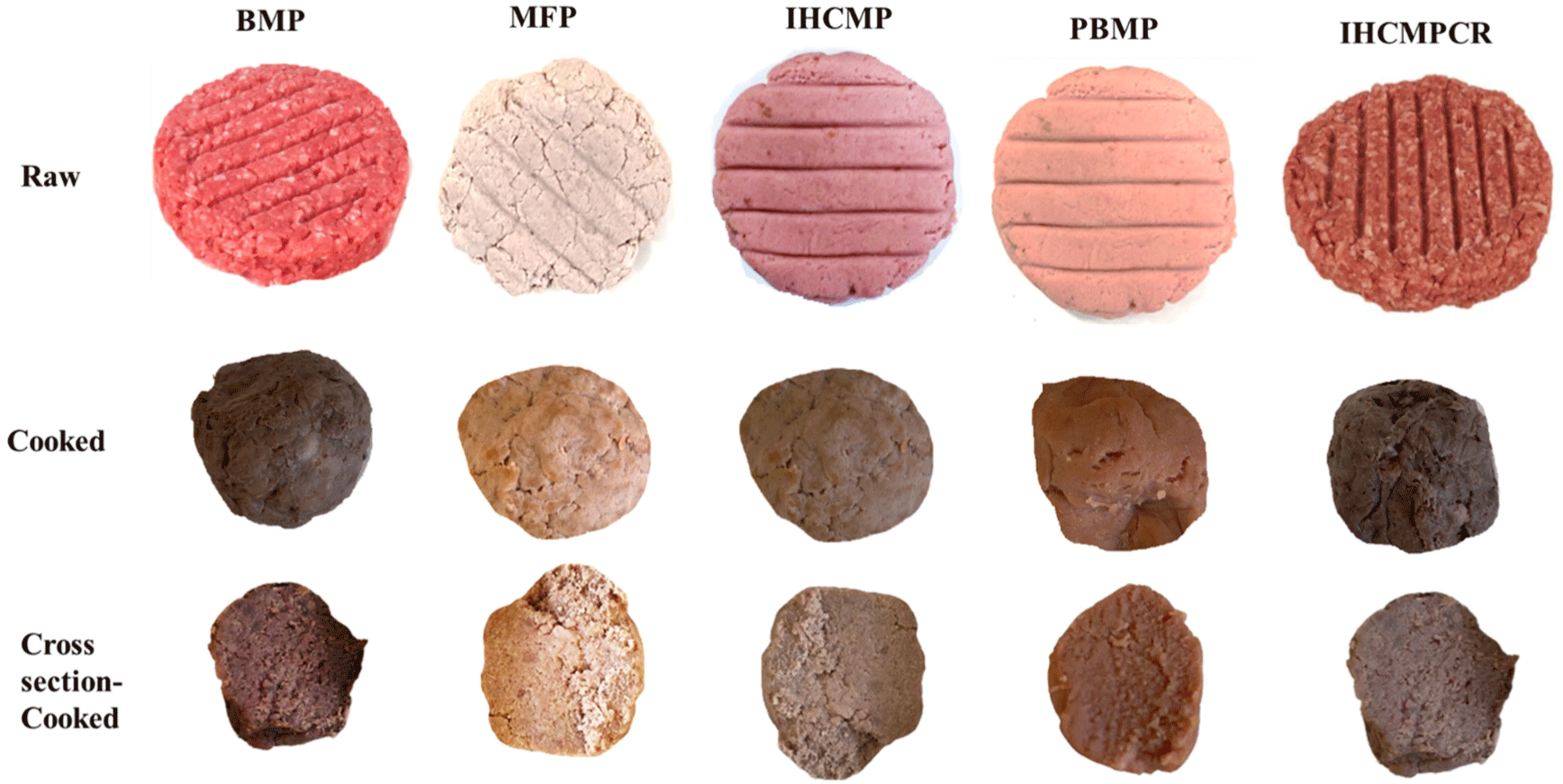
The result of the sensory panel evaluation of patties is illustrated in Fig. 4. The panel found higher juiciness and tenderness were better in BMP and IHCMPCR compared to other samples. Considering sensory attributes IHCMPCR was closer to BMP and stood out from other samples. The sensory panel provided a higher overall acceptance (p<0.05) in BMP, IHCMPCR, and IHCMP compared to MFP and PBMP. Furthermore, PBMP showed a lower score in all parameters with an overall lower acceptance. The detailed values of sensory panel evaluation are provided in Table 4 for better understanding. A similar lower acceptance of PBMP was observed in our previous study (Alam et al., 2024c), where pork hybrid pork steak was formulated with carrot, red cabbage, and ground chickpea.
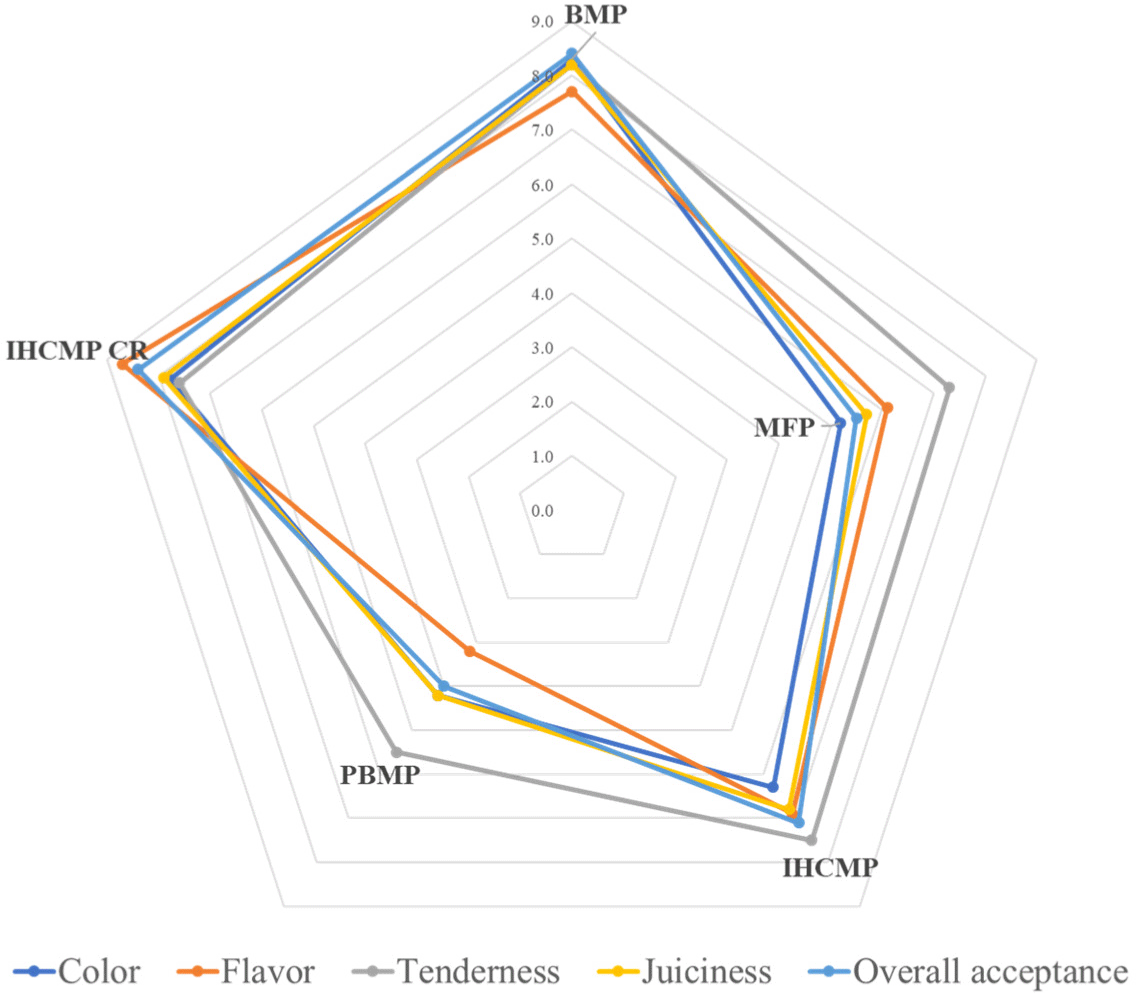
Electronic tongue sensor response values from patties are shown in Fig. 5. ETS revealed negative sourness values (Fig. 5A) for BMP, and MFP and lower values persist in PBMP, IHCMP, and IHCMPCR. PBMP patties had slight bitterness (Fig. 5B) followed by IHCMP and IHCMPCR. The umami intensity was considerably higher (p<0.05) in IHCMPCR followed by IHCMP and BMP (Fig. 5C). A tendency toward lower umami attributes was detected in MFP, PBMP, and IHCMP. IHCMPCR stands out in richness (Fig. 5D) attributes followed by BMP, IHCMP, MFP, and PBMP. Sourness and bitter taste characteristics are influenced by carbohydrate metabolism and the production of acids when being stored (Chen et al., 2021). Additionally, higher levels of soy-based TVP, PPI, SPI, GCP, and some incorporated elements like red beetroot and black pepper used in the PBMP and IHCMP could be responsible for higher sourness and bitterness attributes. Previously, Kobayashi et al. (2010) reported that sourness and bitterness were linked to tannins that come from plant protein. Moreover, saponins, phenolic compounds, alkaloids, peptides, and free amino acids in plant proteins are involved in bitterness and/or astringency (Roland et al., 2017), which is confirmed in the present observation as different plant proteins used in the formulation of PBMP and IHCMP (Table 1). The increased umami and richness intensity in IHCMPCR followed by BMP was similar to a study by Park et al. (2018), where beef patties supplemented with dry-aged crust obtained higher scores for taste, flavor, juiciness, and overall acceptability compared to the control beef patty. During the aging process, the decomposition of meat protein and fat leads to an increase in inosine 5’ monophosphate that stimulates umami sensation (Lee et al., 2022). Thus, the addition of crust improved the taste and acted as an umami enhancer. Furthermore, it also provided a dry aging flavor that enhanced the sensory qualities of IHCMPCR as detected by the sensory panel.
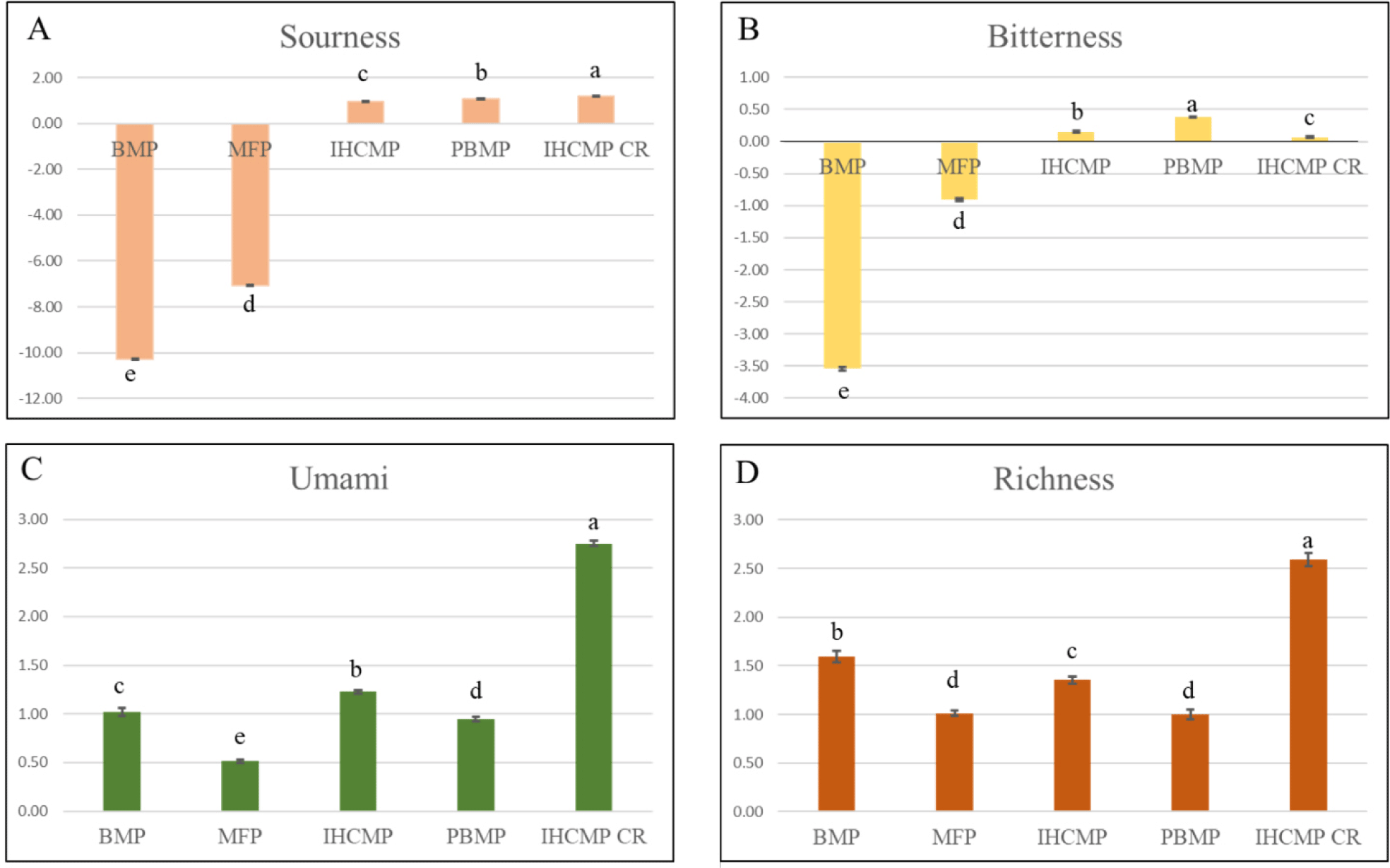
The results from the fatty acid analysis of the patties are given in Table 5. Oleic acid content was higher (p<0.05) in BMP and IHCMPCR, whereas linolenic acid content was higher in IHCMP and PBMP. Only BMP and IHCMPCR contained a very low quantity of omega fatty acids within the patty samples. Fatty acid analysis revealed higher (p<0.05) SFAs and MUFAs in IHCMPCR and BMP compared to IHCMP and PBMP. PBMP and IHCMP contain higher PUFA than the other patty samples. Similar higher SFA and MUFA especially palmitic acid (C16:0) and oleic acid (C:18:1n9c) was observed in hanwoo meat (Lee and Joo, 2022). In the same study, omega 3 and 6 fatty acids were present but in very low quantities in beef. The higher concentration of palmitic (C16:0) and stearic (C18:0) acids in BMP and IHCMPCR than in PBMP and IHCMP could be attributed to the abundant presence of these fatty acids in the adipose and muscular tissues of animals (Wood et al., 2008). The main fatty acids found in plant-based meat analogs [C16:0, C18:0, oleic acid (C18:1), C18:2, and C18:3] revealed in a study by Bohrer (2019), are quite similar to the PBMP and IHCMP in the present study. In a previous study sausages prepared with pork back fat contained the main SFAs C16:0 and C18:0, the main MUFA was C18:1, and the main polyunsaturated fatty acids were C18:2 and C18:3 (Asuming-Bediako et al., 2014). The heatmap (Fig. 6) created separate clusters for BMP and IHCMPCR having higher SFA, MUFA, C14:1 (myristoleic acid), C 16:1 (palmitoleic acid), and omega 3 and 6 fatty acids. The other clusters separated IHCMP and PBMP having higher quantities of PUFA, C:20:0 (ecosenoic acid), and 18:3n3/18:3n6 (lioleic acid). Depending on the fatty acid content the dendrogram separated MFP, BMP and IHCMPCR, IHCMP and PBMP in three separate groups for clarity in understanding the differences between the samples.
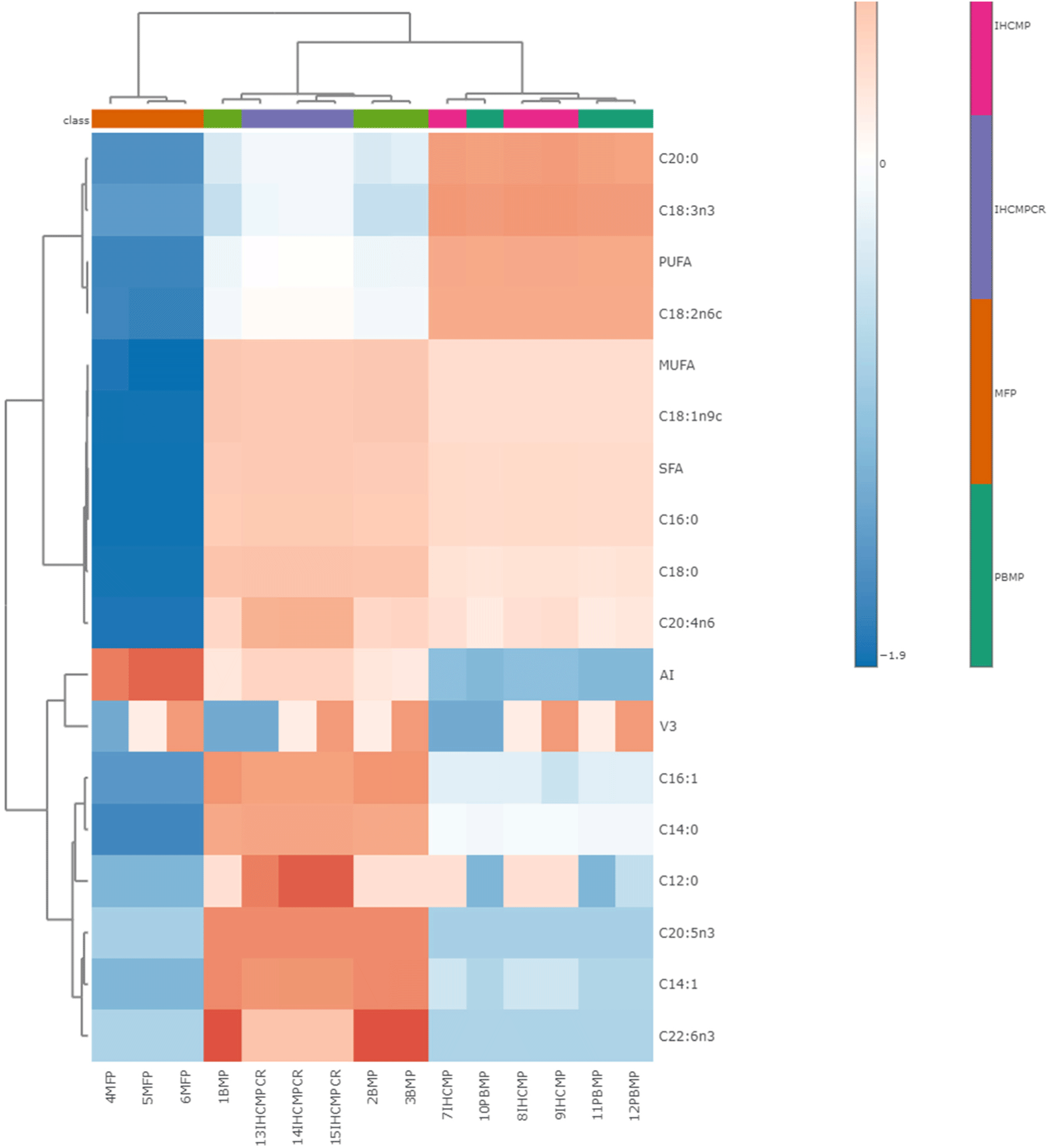
Multivariate analysis using PCA and spectroscopic data is nowadays used for precise differentiation between samples by plotting larger data sets (Hashem et al., 2022). Samples were projected on the first two principal components (PC1 and PC2), where PC1 explained around 82.5% of the variability in the dataset. In addition, samples were colored based on their treatments (Fig. 7A). The PCA biplot (Fig. 7B) indicated that sourness and bitterness were substantially negatively correlated with the PCA 1, whereas umami, richness, hardness, cohesiveness, gumminess, and moisture showed a high positive correlation with PC1. On the other side, sourness and bitterness were highly correlated with PCA 2. The primary occurrence of sample separation was observed in PC1. Thus, as highlighted in Fig. 7A, there was a clear separation between the clusters of the BMP, IHCMP, IHCMPCR, and PBMP. The VIP score plot (Fig. 7C) explains the differentiation between the samples was mainly because of the bitterness and sourness of the samples. On the other hand springiness, moisture, and cohesiveness were the less important variables in the sample differentiation.
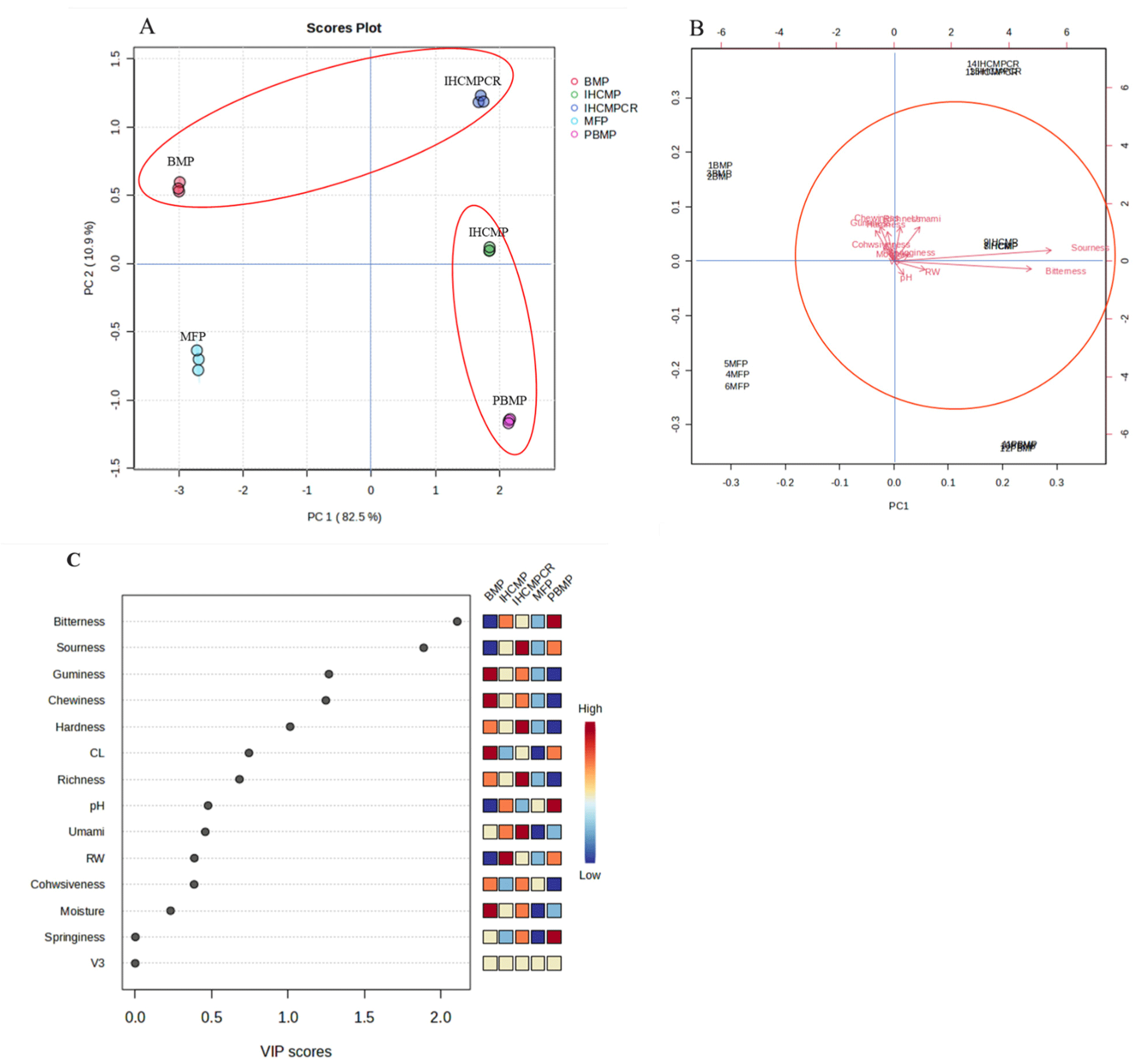
Conclusion
This study involved the imitation of CM using MFP having similar muscle characteristics for the production of an imitation of probable HCM patty termed in the future when scalable production is available. Additionally, in IHCMP dry-aged beef crust was added for taste and flavor improvement. The comparison was done between BMP, MFP, IHCMP, PBMP, and IHCMPCR. Using MFP gave an idea about the physicochemical and rheological quality of IHCMP and may guide us for further R&D in real CM product development. The dry-aged beef crust can be efficiently used to produce any sort of HBM product to improve quality parameters flavor and taste. It is suggested to produce hybrid products in combination with plant materials and dry-aged beef crust to fulfill the increasing demand for meat and as a healthy choice for consumers and a safe environment.