Introduction
Camel has contributed to providing nutritious food (milk and meat) to the nomads and used as a source of transportation. Currently, camel attracted great attention in several parts of the world and rear as important sustainable livestock species due to their multipurpose role and unique ability to adapt to the harsh environment (FAO, 2020; Konuspayeva and Faye, 2021). Taxonomically, domestic camels are divided into two major species: single-humped camel (Camelus dromedarius), also known as Arabian camels, and two-humped camels (Camelus bactrianus). Domestication of C. dromedarius started from five to six thousand years ago in the Arabian region (Ali et al., 2019; Burger, 2016). However, the Bactrian camels were domesticated in Asia’s deserts and cold areas. According to the current statistics of the Food and Agriculture Organization (FAO), the world camel population is approximately 29 million; out of these numbers, it is estimated that 95% are one-humped (dromedary) camels (Trinks et al., 2012). The estimated lactation period of camels is approximately 9–18 months, depending on several factors, including geographical conditions, animal health status, breed, living conditions, and environmental scenarios (Almathen et al., 2016). The worldwide camel milk production is growing at the rate of 2.45% per year (FAO, 2020) due to multiple reseasons: (1) contributed to food security under harsh environmental conditions, (2) increasing market demand owing to the exceptional functional properties, and (3) development of camel dairy industry which could be beneficial for camel owners (Konuspayeva and Faye, 2021).
Camel milk has played a significant economic and dietary role for centuries in several countries in Asia and Africa. Nomadic and indigenous tribes such as the Tuareg, Fulani, Borani, Maasai, and others have consumed camel milk for centuries (Sikkema et al., 2019). Subsequently, it was not considered a commodity, and its sale was often taboo. In the current decade, the demand for camel milk and its dairy products has increased and attracted the great attention to consumers and researchers due to the best alternative of bovine milk because of its superior therapeutic and health-promoting potential (Arain et al., 2022; Izadi et al., 2019). Additionally, camel milk did not undergo any processing treatment except the fermentation method, which was used to increase the shelf life in a harsh environment where the availability of a cold chain is impossible (Berhe et al., 2017; Marsh et al., 2014). Conversely, due to the unavailability of processing technologies, climatic conditions, and low economic status of nomadic peoples, the commercial production and processing of camel milk in several countries of Asia and Africa have been growing at a very slow pace (Agyei et al., 2020). Milk is the most important and widely used animal derived food in the world’s dry and poor regions, especially in Africa and Asia, but the intake is very low (Pica-Ciamarra et al., 2014). The per capita milk consumption of nomadic peoples living in Africa and other countries is approximately 30 liters/year, compared to the world milk consumption of 214 liters/year (Agyei et al., 2020). The lower consumption and production of milk in these regions attributed to the production and development of exceptionally diversified dairy products in Africa and Asia. Since ancient times, the marketing and processing of camel milk have remained insignificant due to the nonexistence of processing amenities in the camel nurturing areas; hence, unprocessed camel milk has continuously remained limited at family level by the nomads. Additionally, in contrast to bovine, very few food products derived from camel milk are available in the present market. The unique composition, inherent functionality, and presence of a slightly higher concentration of bioactive compounds are challenging for the dairy industry to convert camel milk into diversified functional dairy products with superior nutritional properties. Recently, camel milk is becoming the subject of commercial and research interest under the present scenario of global warming that is presenting challenges for the productive performance of cattle, especially in the dry regions, where the camel is a choice to provide milk and meat for the human population and adopt warm climatic conditions (Ereifej et al., 2011).
In contrast bovine milk, camel milk is composed of similar proportion of major constituents including protein, fat and lactose. However, the chemical makeup, distribution, and molecular structure of camel milk proteins and fat are quite different from the other mammalian species (Berhe et al., 2017). Moreover, proteomic evaluation of camel whey proteins showed a significant difference in structure and biological function compared to the cow, buffalo, yak, and goat whey proteins (Yang et al., 2013). Therefore, better understanding of techno-functional properties and chemical composition is required along with the optimization of new technologies to further develop the camel milk market to provide functional dairy products (Al haj and Al Kanhal, 2010; Faye, 2016; Konuspayeva et al., 2017). The present review summarizes the recent development regarding the processing and transformation of camel milk into functional dairy products and also highlights the future directions and processing constraints associated with the processing of camel milk. It is also envisaged to identify suitable processing conditions for developing innovative dairy products at the industrial level.
Chemical Composition of Camel Milk
The gross chemical composition of major nutrients (water, lactose, fat and protein) found in camel milk is more or less similar to cow milk, while significant variation has been observed in the micronutrient and molecular structure of major ingredients (Baig et al., 2022). Fig. 1 showed the chemical composition of camel milk. The average protein distribution is presented in Table 1. It was reported that the average water content of camel milk is approximately 87%–89% which was quite similar to cow milk. However, the water content of camel milk is influenced by several factors, including the availability of drinking water, environmental temperature, and agroecological conditions (Fuquay et al., 2011). According to the study of Konuspayeva et al. (2009), the protein content found in camel milk is 3.03±0.76%, which is similar to cow milk. The camel milk protein consists of 61.8%–88.5% casein, and the remaining is the whey proteins, which was higher than buffalo (0.68%), goat (0.53%), sheep (0.66%), and bovine (0.47%) milk (Ereifej et al., 2011; Rafiq et al., 2016). Camel milk derived casein are further classified into α-casein (α-CN), β-casein (β-CN), and κ-casein (κ-CN; Al haj and Al Kanhal, 2010; Nahar et al., 2016). The size of camel casein micelles is about 260–300 nm, which is greater than bovine milk (100–140 nm; Hailu et al., 2016a). Camel milk comprises 20%–35% whey proteins, including α-lactalbumin, serum albumin, lactoferrin, peptidoglycan recognition protein, and immunoglobulins (Hinz et al., 2012; Laleye et al., 2008). However, β-lactoglobulin is the predominant whey protein found in bovine milk that contributes to initiate the allergic reactions in human infants, which is naturally absent in camel milk (Omar et al., 2016). This unique feature promoted the use of camel milk as a promising alternative for infant formula milk (El-Agamy, 2009).
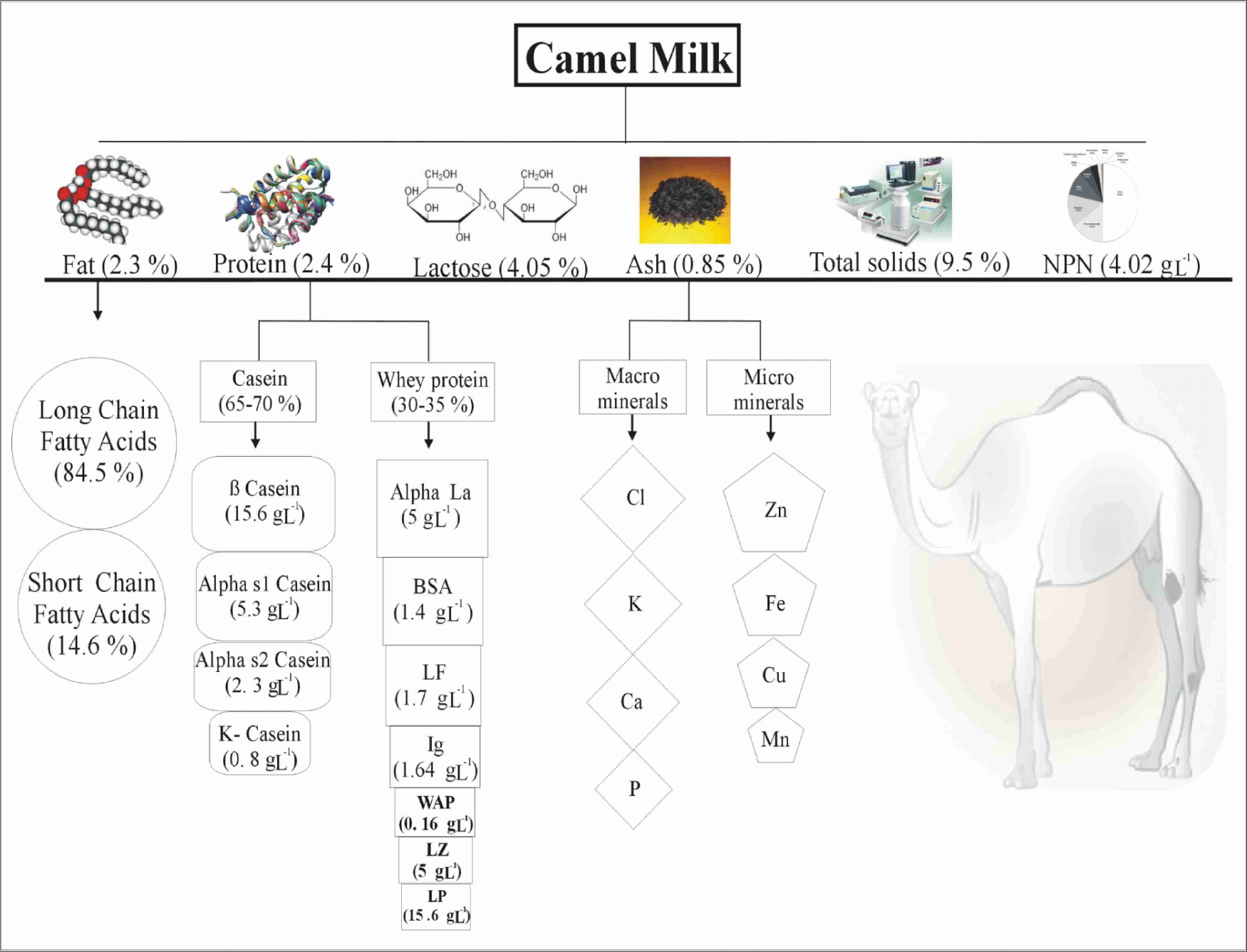
Adapted from Claeys et al. (2014) with permission of Elsevier.
Adapted from Baig et al. (2022) with permission of Elsevier.
The lactose content of camel milk ranges from 3.3% to 5.4% depending on the season, environmental temperature and availability of water and feeding resources (Ismaili et al., 2019). However, under similar agro-climatic conditions, the lactose content remains constant during the entire lactation period in camel milk (Gammoh et al., 2020). In extreme/harsh climatic conditions the taste of camel milk changes from sweet to sharp and salty due to the reduction of water and lactose content and increased concentration of minerals (Al-Juboori et al., 2013).
Like other nutrients found in camel milk, fat content ranging from 1.8% to 4.3% and influenced by several factors (environment, availability of fodders, stage of lactation, season and housing management; Jilo and Tegegne, 2016). Chemically, camel milk fat contains lower proportion of short chain fatty acids (SCFA) and higher percentage of long chain fatty acids (LCFA) as compared to the bovine milk. Additionally, camel milk fat contains higher content of cholesterol (34.5 mg/100 g) than that of bovine milk (25.63 mg/100 g; Nahar et al., 2016). The size of camel milk fat globules is about 2.99 μm, which was smaller than the buffalo and cow milk fat globules 8.7 μm, while similar to the goat milk 3.19 μm; these unique features contributed to the white color of camel milk (Al haj and Al Kanhal, 2010; El-Zeini, 2006). Camel milk is naturally whiter than cow’s milk. The white color comes from the presence of casein protein and fat globules in the milk. Camel milk can look a little bit whiter than cow’s milk because it has less carotene and smaller fat globules (SFGs) than cow’s milk.
The total solid (TS) content range between 7.7% to 12.1% with an average value was 11.97%, which is quite similar to the goat milk but lower than bovine and buffalo milk (Yoganandi et al., 2014). The variation of the TS content in camel milk is mainly attributed to the availability of water and fodder, climatic conditions, stage of lactation and season of the year (Brezovečki et al., 2015).
Despite the major milk constituents, camel milk is rich in micro-nutrients, including minerals and vitamins. The vitamin C content of camel milk is 3–5 times higher than that of bovine milk. Furthermore, camel milk contains a higher level of folic acid, niacin (B3), vitamin B12, and pantothenic acid, while the other vitamins (A, E, B, β-carotene, and riboflavin) found in lower concentration (Stahl et al., 2006). It was also reported that camel milk contains substantially higher levels of some micro minerals including copper (Cu), sodium (Na), potassium (K), iron (Fe) and magnesium (Mn), while the calcium (Ca), phosphorus (P) and magnesium (Mg) contents have closely resembled with the bovine milk (Mehaia, 1996; Sawaya et al., 1984).
Physical Properties of Camel Milk
Camel milk has unique physicochemical properties that distinguish it from the milk of other livestock species. The milk is opaque-white in color and has a typical milky odor, slightly salty, and little acidic, with a pH ranging from 6.2 to 6.5. Camel milk has a sharp sweet taste, which is attributed to the types of fodder available in the grazing area and the quality of drinking water (Abd El-Aziz et al., 2022; Sakandar et al., 2018). The homogenous distribution of small size fat globules throughout the milk contributes to its prominent white color. The acidity of camel milk can vary depending on various factors such as the animal’s diet, age, and health, as well as the handling and processing of the milk. Typically, the acidity of fresh camel milk falls within the range of 0.13% to 0.17% lactic acid. The pH of fresh camel milk also varies, usually ranging from 6.2 to 6.5, which is slightly more acidic than cow milk (Park et al., 2007; Yoganandi et al., 2014). The freezing point of camel milk is typically between –0.53°C to –0.57°C, which is slightly lower than the freezing point of cow’s milk due to its lower fat content and higher concentration of minerals. It was concluded that camel milk’s physical properties are quite dissimilar from other livestock species’ milk.
Techno-Functional Properties and Processing Constraints of Camel Milk
Techno-functional properties of milk, including solubility, water holding capacity, physical behavior, chemical structure, foaming capability, gelling formation and emulsifying properties, are known to play a vital role in the milk processing techniques and could be considered substantial contributors in the transformation of new food products from animal origin (Shokri et al., 2022). In general, the majority of milk obtained all around the world is exposed to various processing treatments by using modern technological procedures to increase the shelf life and developed functional dairy products with superior nutritional value and health-promoting potential. The possible use of camel milk to develop dairy products depends on physicochemical and techno-functional properties (Konuspayeva and Faye, 2021). The functional properties of food can be affected by the application of processing technologies, quality of food and finally, their acceptability and utilization of food formulation (Mahajan and Dua, 2002). The transformation of camel milk into processed dairy products is a challenging task and requires suitable technologies due to multiple factors such as unique chemical composition, presence of multicomponent colloidal system, size of protein micelles, size of fat globules and presence of antibacterial compounds (Arain et al., 2022; Bornaz et al., 2009). Recently, the techno-functional properties of camel milk have been of greatest interest to the scientific community because the technologies used for cow milk are not suitable for transforming camel milk into dairy products. Therefore, the advance technologies and development of new protocols for the camel dairy industry are urgently required to overcome the associated challenges regarding the transformation of camel milk into dairy products.
Impact of Processing Treatment on Camel Milk
The gross chemical composition of camel milk and cow milk may be similar, however the molecular properties of the milk components are different, particularly in the case of fat and proteins. For example, the fat globules in camel milk are smaller than those in cow milk, making it more difficult to process and transform into dairy products using the same methods typically used for cow milk (Hailu et al., 2016b). The key differences including weak or incomplete curd formation, incomplete fermentation, heat stability, coagulation properties or rennetability along with lower yield of final dairy products (Hailu et al., 2016a; Ramet, 2001).
Available literature has suggested that camel milk showed poor heat stability in response to thermal treatment compared to the milk of bovines (Hassan et al., 2007). The poor heat stability of camel milk is mainly attributed to the larger size of casein micelle, lower content of κ-casein (5% in camel milk while 13.6% found in bovine milk) and absence of β-lactoglobulin (Al haj and Al Kanhal, 2010). Camel milk contains a higher concentration of whey proteins than cow milk which are more heat sensitive than casein; these attributes make camel milk more susceptible to heat treatment, resulting denaturation, aggregation, and precipitation affecting the sensory, nutritional, and functional properties of milk and by-products. Furthermore, heat treatment including low temperature long time (LTLT) and high temperature short time (HTST) altered the color attributes such as CIE L*, CIE a*, and CIE b* in both bovine and camel milk (Omar et al., 2018). It has been reported that the reduction of CIE L* of camel milk in response to thermal processing (HTST) might be due to the breakdown of casein micelles into smaller particle size leading to enhance heat susceptibility of camel milk and ultimately developing the state of casein aggregation or precipitation (Hailu et al., 2016b; Needs et al., 2000). Moreover, another study reported that the composition and properties of camel milk was significantly affected by thermal treatment (sterilization, LTLT and HTST) and altered the average values of acidity and pH of milk (Elhasan et al., 2017). Furthermore, the reduction of camel milk pH in response to rising temperature might be associated with the detachment of ionizable groups such as inorganic phosphate (Pi) from milk protein (Alhaj et al., 2011). Camel milk pH declined linearly when the heating temperature is about <80°C (Ma and Barbano, 2003). Exposure of thermal treatment to camel milk causes the mineral imbalance mainly (Ca+Mg; citrate+P); these changes are reversible upon subsequent cooling; however, the reaction is irreversible if the heating temperature is >100°C (Ma and Barbano, 2003). Moreover, camel milk exposed to severe thermal treatment further declines the pH owing to the thermolytic breakdown of lactose to organic acid, mainly formic acid (Berg, 1993). Study of Berhe et al. (2018), demonstrated that declining trends of pH were also observed in camel milk by using commercial starter culture; however the acidification rate of camel milk were noted slower in comparison to the bovine milk. Similarly, another study reported that the rate of acidification or pH decline were also influenced by the type of starter culture used for fermentation (Bekele et al., 2019). The results indicated that the thermophilic culture induces faster acidification compared to the mesophilic culture. Conclusively, further in-depth studies are required to monitor the heat stability of camel milk during various developmental steps of camel dairy products.
Heat treatment of milk and dairy products is a most important tool to enhance the shelf life and improve the hygienic standards of food commodities for consumers. There are several thermal processing methods commonly practiced in the dairy industry, including pasteurization (both short time and longtime), ultra-high temperature, and sterilization (Benabdelkamel et al., 2017). The selection of heat treatment process mainly depends on the type of milk subjected to thermal processing and physicochemical behavior of milk in response to heat treatment (Alhaj et al., 2013). Earlier studies suggested camel milk showed poor heat stability than bovine milk at higher temperatures (130°C; Al haj and Al Kanhal, 2010; Arain et al., 2023; Farah, 1993). Camel milk has very unstable and low heat coagulation time (2–3 min) when heated at 120°C to 130°C (Farah, 1993; Sagar et al., 2016). This behavior of camel milk is mainly associated to the absent of β-lactoglobulin and lower concentration of heat stable κ-casein as compared to the milk of other mammals (Barłowska et al., 2011). According to the study of Kouniba et al. (2005) it has been suggested that pasteurization is the only preferred method for the thermal processing of camel milk at conventional and industrial level.
Generally, deactivating enzyme alkaline phosphate (ALP) is the main parameter to determine the successful pasteurization of bovine milk in the dairy industry (Rankin et al., 2010). However, camel milk has its own pasteurization conditions, owing to the camel milk ALP is heat-resistant and shows activity at higher temperature (90°C; Elagamy, 2000). Consequently, the study of Didier et al. (2001) reported that the most effective indicators for pasteurization in camel milk are glutamyltranspeptidase or leucine arylamidase heat labile enzymes and showed heat sensitivity at 75°C for 30 s and 75°C for 28 s respectively during thermal treatment. Another study also suggested that the most appropriate pasteurization indicator for camel milk could be the gamma-glutamyl transferase (GGT) when heating the milk at 72°C for 20 min (Wernery et al., 2007). Conversely, the study of Lorenzen et al. (2011) contradicted the above findings concerning the pasteurization indicators of camel milk, it was concluded that the GGT remains active in pasteurized camel milk; however the lactoperoxidase (LPO) could be the most suitable indicator of pasteurization. Similarly, another study also verified that the camel milk LPO was the heat sensitive enzyme compared to the LPO present in bovine milk and suggested that this enzyme could be a suitable indicator for camel milk pasteurization (Tayefi-Nasrabadi et al., 2011). Based on the above findings of various studies, it has been concluded that the pasteurization indicator is quite different than the bovine milk. Therefore, the appropriate indicator for camel milk pasteurization such as lactate dehydrogenase and GGT or other heat-labile enzymes must be explored in future research.
The severity of heat treatment played a significant role during cheese manufacturing from camel milk. The rennet clotting time or coagulation behavior of camel milk are influenced by several factors, including intensity of heat treatment, type of starter culture used and manipulation of cheese processing methodologies (Al haj and Al Kanhal, 2010). Camel milk exposed to mild thermal treatments such as LTLT or HTST pasteurization has shown no adverse effect on clotting time and coagulation properties, the reason behind that the lower temperature restores the mineral equilibrium after cooling (Genene et al., 2019). It was reported that cheese production from camel milk by using the same traditional techniques applied for bovine milk is difficult and sometimes impossible due to multiple factors, including prolonged coagulation time, weak curd formation, and ultimately lower cheese yield, these challenges need to address for future development of camel milk industry (Berhe et al., 2017; Brezovečki et al., 2015). On the other hand, Barłowska et al. (2011) reported that the camel cheese processing difficulties are mainly associated to the larger size of casein micelle, deficiency of κ-casein and casein to whey protein ratio. It is well documented that the milk of other animals like sheep, goat, cow and buffalo contains a balance of casein to whey protein ratio i.e. 80:20, while in case of camel milk this ratio was 72:28; this variation contributed to the cheese processing difficulties (Rafiq et al., 2016). Furthermore, the challenges concerning camel cheese processing were discussed in the cheese manufacturing section.
Recently, the transformation of camel milk into a fermented dairy product like yogurt attracted great attention among researchers and the dairy industry due to the public demand for functional food. Consequently, camel milk yogurt has been introduced commercially in several African and Middle Eastern countries. The schematic overview of camel milk yogurt processing constraints is presented in Fig. 2. Camel milk has a unique chemical composition compared to other mammals’ milk (Galeboe et al., 2018). Interestingly, camel milk showed a different behavior than bovine milk during lactic acid fermentation (Arain et al., 2023). According to the published literature, yogurt making from camel milk is challenging as it presents several technological problems. The study of Berhe et al. (2017) suggested that the processing challenges associated with yogurt manufacturing from camel milk were mainly attributed to poor coagulation ability, resulting in the thin consistency and weak structure of the final product. The texture is the most important organoleptic quality attribute related to the appearance, mouthfeel and overall consumer acceptability of yogurt. The study of El Zubeir et al. (2012) reported that the complete fermentation of camel milk could not be achieved by using the 2.5% of bacterial starter culture with incubation at 37°C for 16–18 h. Camel milk yogurt poses a texture problem with incomplete curd formation and firmness, the curd is fragile and heterogeneous, containing disseminated flakes (Berhe et al., 2017). Indeed, the consistency and viscosity of the camel yogurt was unchanged during the gelling process when compared to the milk of bovine and other dairy species. These challenges are attributed to the unique composition of camel milk and presence of higher concentration of protective proteins and antibacterial constituents that prolongs the lag phase while reducing the decline phase of starter culture during the fermentation process (Attia et al., 2001; Jumah et al., 2001). Additionally, the foaming properties of camel milk are also associated with this technological constraint as foam formation predominantly contributes to the weak and unstable structure of the gel (Lajnaf et al., 2017). Several study attempts have been made to enhance the physicochemical and organoleptic quality of camel yogurt by improving the firmness and reducing the syneresis during production and storage (Abd Elhamid and Elbayoumi, 2017; Khalifa and Ibrahim, 2015). For this purpose, various strains of lactic acid bacteria and food additives have been used to overcome these challenges (Arain et al., 2023; Gorelik et al., 2017; Ifeanyi et al., 2013) On the other hand, Al-Zoreky and Al-Otaibi (2015) reported that the addition of stabilizer (carboxymethyl cellulose, alginate or gum acacia and pectin) into camel milk at the level of 0.6% could not improve the water holding capacity, consistency and coagulum structure of camel yogurt when compared to the bovine yogurt. Overall, camel milk’s unique composition and functional properties are the main obstacle to restricting or prolonging camel milk’s fermentation process (Fig. 2). Altogether, these challenges restricted camel milk yogurt’s industrial production and commercial availability. Therefore, a further research is required to optimize the manufacturing condition to overcome these challenges related to yogurt manufacturing from camel milk.
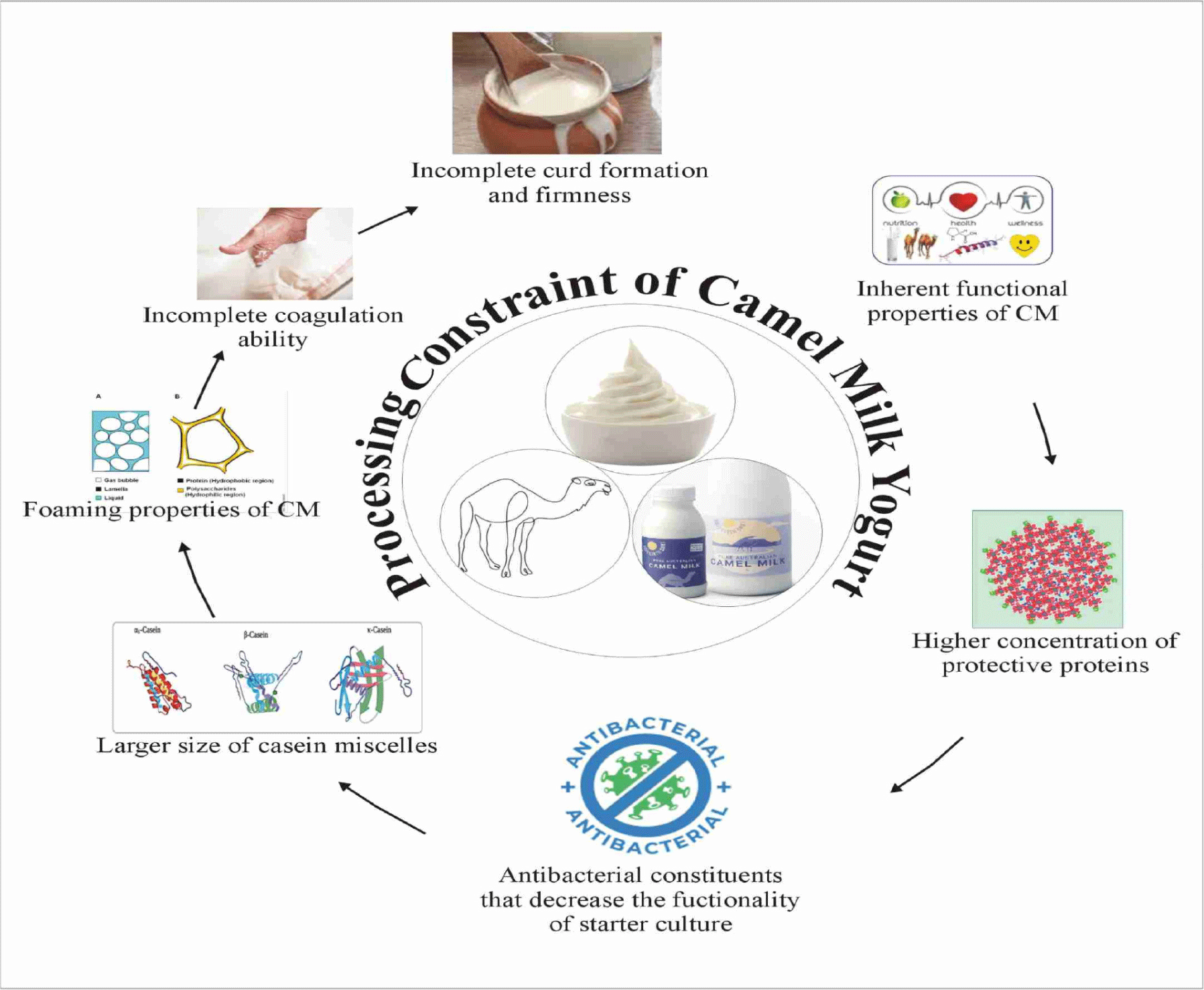
Challenges Associated to Cheese Manufacturing from Camel Milk
The basic principle in cheese making is to separate the milk protein from whey through coagulation. With the advancement of science and technology, the coagulation process is achieved by adding starter culture bacteria, which produces lactic acid, and rennet (substance isolated from calf stomach), containing the flocculation enzyme. The rennet enzyme plays a vital role in cheese making by accelerating the chemical reaction and ensure the separation of curd from the whey. Cheese making from camel milk is technically more difficult and even impossible through the traditional methods that are already used in the dairy industry for other livestock species (cow, buffalo, sheep, and goat) owing to the prolonged coagulation time, weak coagulum and low yield (Al haj and Al Kanhal, 2010; Berhe et al., 2017). The challenges associated with the manufacturing of camel milk cheese are presented in Fig. 3. The limited types of cheese produced from camel milk by acidic separation along with thermal treatments (Dokata, 2014). Published literature has shown that cheese manufacturing from camel milk is possible through several endeavors; however, most studies reported contradictory outcomes regarding cheese yield and organoleptic quality (Mahgoub and Sulieman, 2022). The scientific constraint or issue related to cheese manufacturing from camel milk includes prolong rennet coagulation time; Camel milk requires 3–5 fold more time to achieve successful coagulation in comparison to bovine milk (Farah et al., 1990). The reason behind the prolonged coagulation time is the larger size of casein micelles, concentration and behavior of camel milk protein, and the processing conditions used for cheese manufacturing.
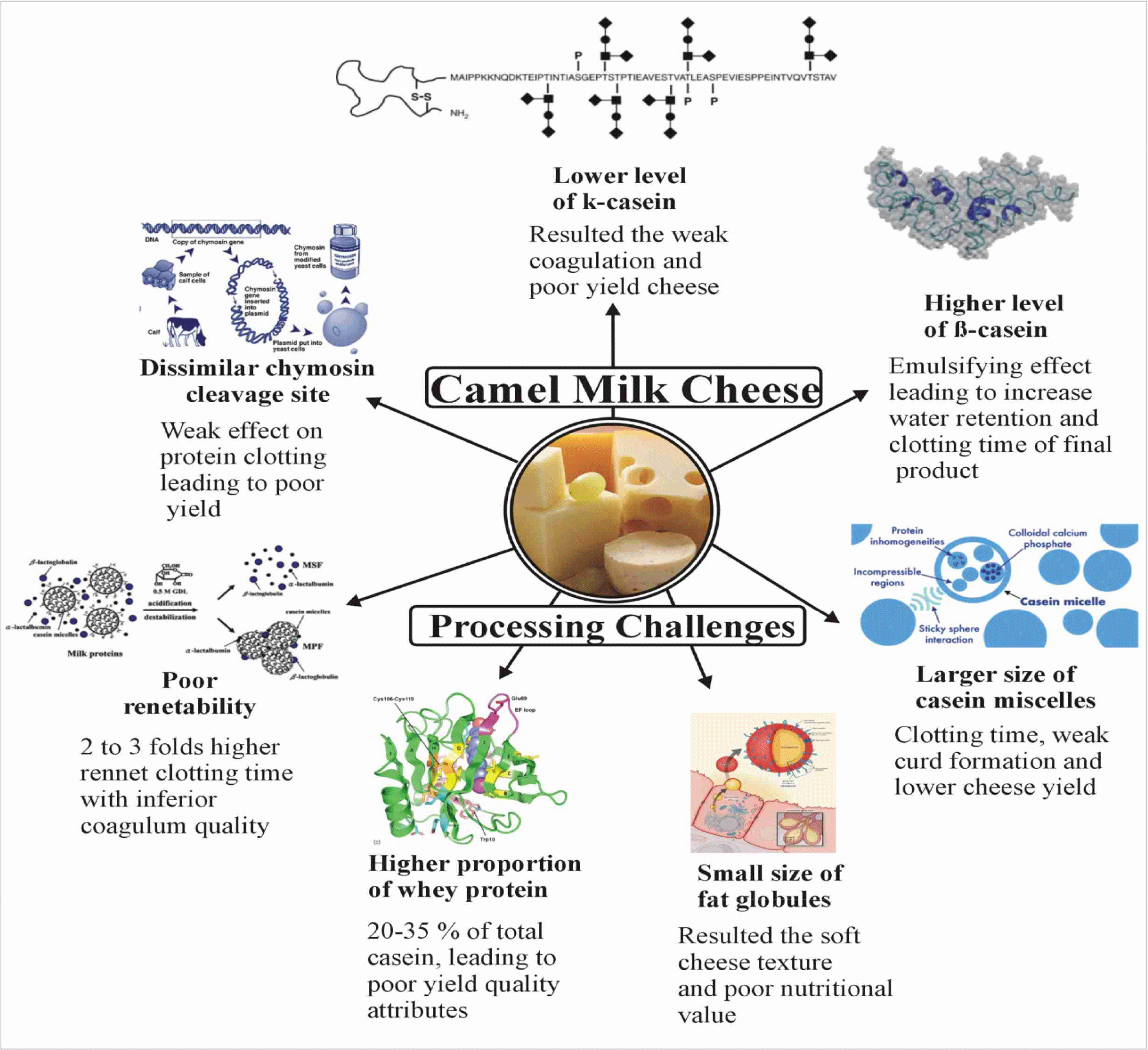
Formation of weak curd: The weak curd of camel milk mainly associated to the lower TS content (11.9%) as compared with other species of livestock including cow, buffalo, and sheep milk, particularly for casein content (El Zubeir and Jabreel, 2008; Mehaia, 2002). Furthermore, the smaller size of camel milk fat globules (2.99 mm), along with poor elasticity and greater fragility of cheese gel texture, also contributed to the weak curd formation during cheese making (Ho et al., 2022).
Rennet action: The weak enzymatic activity of rennet (cheese enzyme) cause incomplete coagulation with thrombus formation, resulted weak and inconsistent curd formation, which negatively influences the yield and organoleptic quality of the final product.
The poor yield of cheese: the amount of soft cheese obtained from camel milk is 50% less than that of bovine milk. It has been reported that a kilogram of cow’s milk produces about (250 g) of cheese, whereas a kilogram of camel milk produces about (120 g) of cheese yield; the poor yield might be attributed due to the lower amount of TSs and chemical behavior of camel milk under cheese processing (Mehaia, 2002).
The total casein mostly influences the firmness of cheese to kappa-casein ratio; the higher concentration of kappa casein led to produce firmer cheese. The variation found in the protein fraction in camel milk, especially the lower amount of kappa-casein (2%–4%), which is much lower compared to cow milk (13%–15%) and buffalo milk (13%–20%; Ho et al., 2022). Moreover, the dissimilar chymosin target sites found in camel kappa-casein compared to the bovine counterpart contributed to incomplete coagulation. Additionally, the large micelle size and level of total casein is another important feature of camel milk that contributed to the long clotting time, weak curd formation, and lower cheese yield (Kappeler et al., 2006). Moreover, commonly used chymosin in the commercial dairy industry could not achieve proper clotting of casein micelles, leading to weak curd formation from camel milk. Hence, optimal clotting and obtaining a firm coagulum is the key processing challenge faced by the camel dairy industry regarding cheese formation on a commercial scale (Konuspayeva, 2020). Earlier studies have demonstrated that camel milk casein contains larger micelles (380 nm), nearly double as compared to bovine (150 nm), goat (260 nm), and sheep (180 nm; Barłowska et al., 2011). Consequently, camel milk showed 2 to 3 folds higher rennet coagulation time with inferior coagulum quality than the bovine milk during cheese making (Farah and Bachmann, 1987).
Additionally, some other factors significantly affecting the suitability of camel milk for cheese making included the stage of lactation, hot environmental conditions, dry season, and availability of fodder and water (Khan et al., 2004; Konuspayeva et al., 2014). Despite these challenges, researchers have made several attempts to develop different types of camel milk cheeses. However, a further research is still needed to address the technical obstacles and improve the overall quality of camel milk cheese. For instance, more studies are needed to better understand camel milk’s chemical and physical properties and how they affect the cheese-making process.
Camel Milk Butter and Its Production Challenges
The fat content in camel milk ranges from 1.2% to 6.4%, which is not similar to bovine milk (Konuspayeva et al., 2009). Milk fat content can vary depending on the animal species, and this is due to differences in their unique chemical composition and state of dispersion. The dispersion state of milk fat plays an important role in the creaming rate, technological and rheological parameters followed by organoleptic quality of the final product, such as viscosity, separation rate, conductance, color, emulsion stability, and fitness for butter and cheese processing. Camel milk butter is one of the important dairy products obtained from camel milk, having significant nutritional value for humans and infants. In arid areas of the world where camel are reared as a dairy animal, this commodity plays an important role to fulfill the nutritional requirements and uplift the health status of the people; due to these reasons, there is increasing demand for such type of functional food all over the world. Traditionally, camel milk butter was produced by using the same methodology (churning process) as applied for butter making from cow or buffalo milk in several regions of the world, including Sinai Peninsula, north Kenya, and Algerian Sahara (Dokata, 2014; Farah et al., 1989; Mourad and Nour-Eddine, 2006). Ghee (a type of clarified butter) obtained from camel milk is a popular dairy product in Indo-Pakistan, also known as filtered butter. In contrast, the yield of final product is lower than the cow and buffalo milk (Parmar, 2013). The butter processing from camel milk is complicated by using the traditional methodology for other animal milk due to its creaming capabilities and unique properties of milk fat (Asres and Yusuf, 2015). Fig. 4 summarizes the inherent compositional factors that collectively contributed to the butter processing difficulties from camel milk.
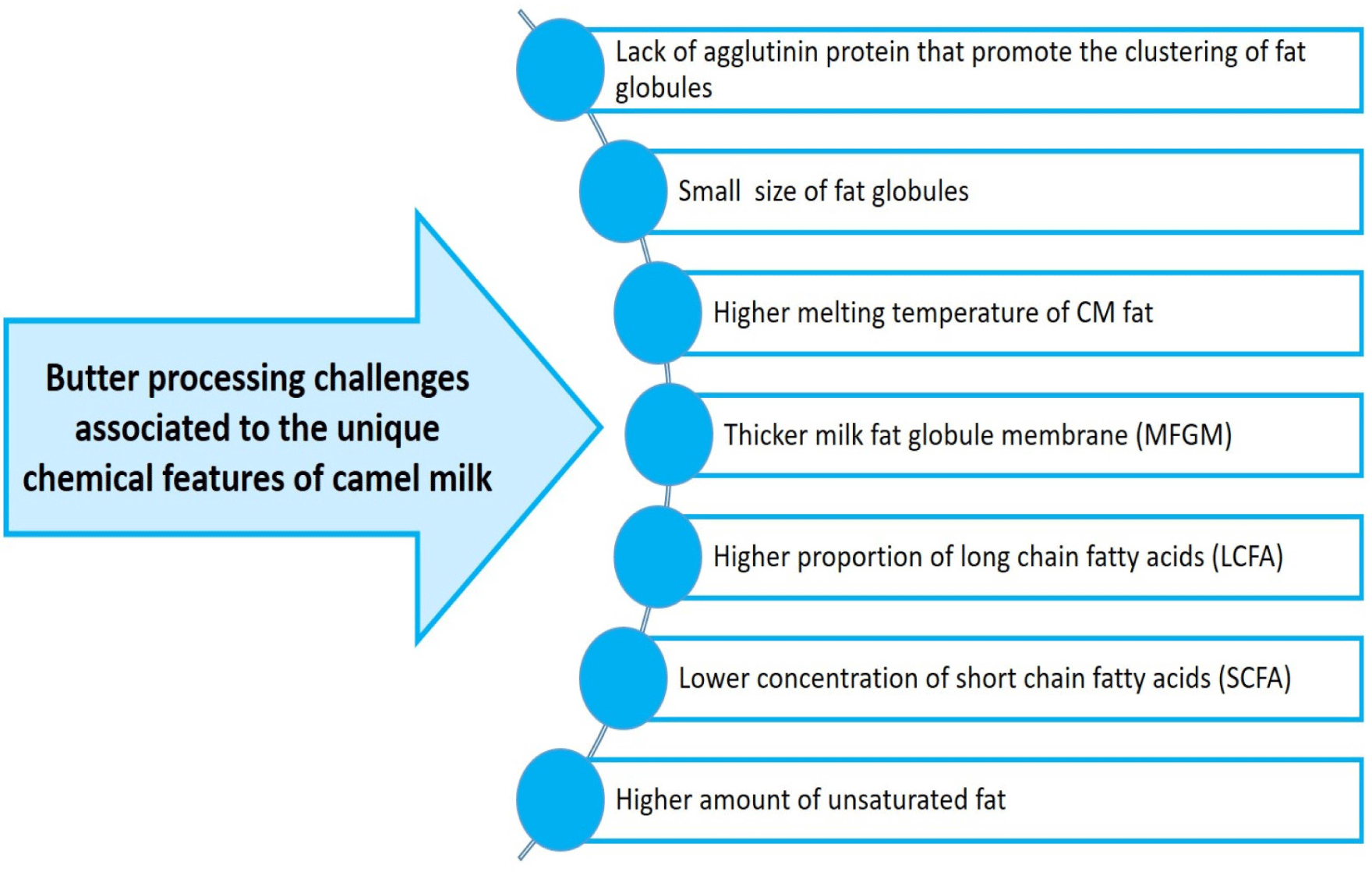
The main processing constraint associated with the camel milk butter preparation is the absence of agglutinin protein, higher proportion of LCFA, lower concentration of SCFA (6 to 8 times lower than sheep, goat, cow, and buffalo), higher amount of unsaturated fat, smaller size fat globules and true fat bounded by a thick milk fat globular membrane, all these factors contributed to reduce the creaming capability of camel milk during butter preparation (Abbas et al., 2013; Attia et al., 2000; Haddad et al., 2011). In addition, the fat distribution in camel milk is micelle-like globules, and its tightly bound with inherent proteins make it difficult to separate by traditional churning method. Therefore, the churning temperature and agitation method during butter processing must be optimized for efficient butter production from camel milk (Muthukumaran et al., 2022). In the case of butter manufacturing, it is necessary to use milk containing a dominant share of larger fat globules (LFGs) with minimum share of milk fat globule membrane, contributing to the lower cholesterol content in the final product. In ideal condition, butter produced from cow milk/cream have softer consistency (due to higher amount of unsaturated fatty acids), higher spreadability and predominant yellow color. Moreover, due to the weak or thin globular membrane of LFGs, it is easily destabilized during the churning process, which contributes to increase the yield of butter. Subsequently, butter obtained from camel milk contains a predominant share of SFGs with higher content of membrane fragments, leading to inferior organoleptic quality and easily undergo oxidative rancidity process, leading to the higher concentration of water and protein (Barłowska et al., 2011). Additionally, camel milk has two main dissimilarities in milk fat microstructure when compared to cow milk; firstly, the camel milk fat has a greater number of SFGs, which contributed to cause technological problem during butter manufacturing (Attia et al., 2000; Karray et al., 2005a; Karray et al., 2005b); secondly, the camel milk fat encapsulated by a thicker globular membrane containing an abundant amount of phospholipids that contributed to enhance the emulsion stability of camel milk (Attia et al., 2000). On the other hand, camel milk contains minute quantity of butyric acid (0.5%), which was abundant in cow milk (5%), that might be contributed to raise the melting point of camel milk fat (Konuspayeva et al., 2008). The melting point of camel milk-fat is somewhat higher (41°C–43°C) than the bovine fat, that makes it difficult to churn at lower temperature (Berhe et al., 2013; Farah et al., 1989). These compositional factors significantly contributed to the lower butter yield and inferior organoleptic quality (Berhe et al., 2013; Farah et al., 1989). Consequently, due to these unique compositional features (microstructure and fatty acid profile), it is difficult to obtain butter from camel milk using the same methodology as in other bovine milk.
Conclusions and Future Directions
Camel milk has gained worldwide attention among researchers and the dairy industry owing to its exceptionally superior nutritional and health promoting effects. The production and consumption of camel milk and dairy products have increased globally. The processing of camel milk and dairy products is a modernized and unique feature compared to the milk of bovines and other species. However, the processing methodology used to transform raw camel milk into fermented dairy products, pasteurized milk, and powder or numerous other products is facing several challenges, including the systemic application of already certified technologies for bovine milk is not suitable for camel milk and the insufficient research outcome regarding the physical behavior of camel milk in response to the processing treatments. The unique structural, inherent functionality and chemical biodiversity of camel milk is the key processing constraint associated to the processing difficulties of camel milk. Moreover, it is an urgent need of advance research concerning the processing constraint associated with camel milk and developing the most suitable methodology for the camel dairy industry. Therefore, the current review attempts to provide insights into the techno-functional properties of camel milk and associated processing constraints. Furthermore, this review also provides basic knowledge regarding camel milk’s chemical composition and physicochemical behavior in respect to the various processing treatments.