Introduction
Diabetes mellitus is a serious chronic metabolic disorder, associated with life-threatening complications (Elbashir et al., 2018). It is characterized by a state of chronically elevated blood glucose levels (hyperglycemia) with altered nutrient metabolism, such as carbohydrates, fats, and proteins, in the body (American Diabetes Association, 2014). Moreover, the oxidative stress state associated with the generation of reactive oxygen species (ROS) increases in both type I and type II diabetes (Omari et al., 2019; Sabu and Kuttan, 2002). ROS generated during oxidative metabolism can cause various disorders.
α-Glucosidase enzyme inhibitors, as antidiabetic drugs, can lower blood glucose levels by reducing the intestinal absorption of carbohydrates (Buchholz and Melzig, 2016). Additionally, hyperlipidemia is a common feature in patients with diabetes mellitus and is one of the factors that is associated with considerably increases the risk of premature atherosclerosis (Schofield et al., 2016). It is known that pancreatic lipase regulates the digestion and absorption of dietary lipids (Mhatre et al., 2019). Therefore, inhibition of this enzyme may help prevent complications by regulating lipid levels in the blood of diabetic patients through reducing dietary fat absorption into the body.
Despite the growing interest in functional foods that modulate physiological activities by inhibiting these enzymes, the possibility of developing successful and targeted natural products to safely manage these diseases remains unexplored.
Yogurt, obtained by fermenting milk using lactic acid bacteria, contains proteins, vitamins such as vitamins A and B, and minerals such as calcium and manganese. Recently, yogurt has been extensively studied to further enhance its functions using bioactive substances from edible plants. Numerous studies have indicated that the addition of these substances increases the concentration of polyphenols and flavonoids, thus increasing the antioxidant and antimicrobial activities of yogurt (Halah and Nayra, 2011; Kang et al., 2018; Lim, 2018).
Carthamus tinctorius L., commonly known as safflower, belonging to the Asteraceae family (Delshad et al., 2018), is an annual herbaceous crop. It has been cultivated in India, Iran, and China for a long time (Asgarpanah and Kazemivash, 2013). Safflower is used as a traditional herbal medicine to promote blood circulation, regulate menstruation, and alleviate pain of joints (Delshad et al., 2018). Some studies have reported that safflower has more than 200 compounds including flavonoids, phenylethanoid glycosides, coumarins, fatty acids, steroids, and polysaccharides (Asgarpanah and Kazemivash, 2013). Furthermore, in in vitro and in vivo studies, hydroxysafflower yellow A, a major bioactive compound in safflower, has been found to have a strong oxidative radical-scavenging effect (Tian et al., 2008; Zhao et al., 2018).
The present study has focused on whether the addition of safflower extract containing various phytochemicals to yogurt is effective in the antioxidant activity and inhibition of α-glucosidase and pancreatic lipase. Therefore, this study was conducted to investigate the biochemical, microbiological, sensory characteristics, and physiological effects including α-glucosidase and pancreatic lipase activity inhibitory effects of yogurt supplemented with safflower petal (SP) extract in order to enhance the functionality of yogurt.
Materials and Methods
Dried SPs were purchased from a local market (Daejodong, Seoul, Korea). For ethanol extraction (SPE), 100 g of dried SPs was precipitated with 900 mL of 99.9% ethanol in a shaking incubator at room temperature for 15 h. For water extraction (SPW), 100 g of dried SPs was soaked in 900 mL of 100°C hot water for 1 h using a reflux condenser (Reflux-A, Reservoir; LK Lab Korea, Namyangju, Korea). These extracts were centrifuged at 1,889×g for 15 min; then, the supernatant was collected and passed through qualitative filter paper No. 1 (Toyo Roshi Kaisha, Tokyo, Japan) and 0.22 μm syringe filter (Sartorius, Goettingen, Germany). The aqueous extract was concentrated using a vacuum freeze-dryer at –80°C (UnifreezTM FD-8; Daihan Scientific, Wonju, Korea). The concentration of SPE and SPW was 50 mg/mL.
SPE and SPW were added to sterilized milk (Maeil Dairies, Seoul, Korea) at concentrations of 0%–1.0% and homogenized using Homogenizer T 25 (Ika, Staufen, Germany) at 25°C for 10 min, and then pasteurized at 85°C for 15 min. Thereafter, the samples were immediately cooled to 43°C and inoculated with a commercial yogurt starter culture (0.02%, v/v) of lactic acid bacteria (Lyofast YAB 450 AB; Sacco s.r.l., Codaragok, Italy) containing Streptococcus thermophilus, Lactobacillus delbrueckii spp. bulgaricus, Lactobacillus acidophilus, and Bifibobacterium animalis spp. lactis. Yogurt added with 0% safflower extract was used as control. These yogurt samples were fermented at 43°C for 6 h and stored at 4°C until further analysis.
Moisture and total ash content was measured using the methods of Association of Official Analytical Chemists (2000). The concentration of proteins, crude fats, lactose, and total solids was measured using Mikloscan (Milkoscan Minor 78110; Foss, Hillerød, Denmark). The pH of yogurt samples was measured using a digital pH meter (Model 735P; IS Technology, Incheon, Korea). Titratable acidity (TA) was determined by adding 9 mL of dH2O to 1 g of yogurt sample and then titrating the yogurt mixture to pH 8.3 with 0.1 N NaOH. The amount of acid produced was calculated as follows:l
where, VNaOH is the volume of NaOH required to neutralize the acid.
Viscosity of yogurt supplemented with SP was measured using a viscometer (Model LVDV 1+; Brookfield Engineering Laboratories, Middleboro, MA, USA) with spindle No. 63 at 50 rpm for 5–8 min.
Viable cell count in yogurt was determined on a Streptococcus thermophilus agar (Sigma-Aldrich, St. Louis, MO, USA) plate after incubating at 37°C for 48 h. The results are expressed as CFU/mL.
2,2-Diphenyl-2-picrylhydrazyl (DPPH) inhibition was determined as described previously (Kang et al., 2018). Briefly, each yogurt extract (16 μL) was mixed with 320 μL of 0.15 mM DPPH solution (Sigma-Aldrich). The mixture was shaken and incubated at room temperature for 15 min. The absorbance of the solution was measured at 515 nm using an ELISA reader (Synergy 2; BioTek Instruments, Winooski, VT, USA). Inhibition of DPPH oxidation (%) was calculated as follows:
where, A is the absorbance at 515 nm.
2,2′-Azino-bis (3-ethylbenzothiazoline-6-sulfonic acid) (ABTS) radical-scavenging activity was determined using the method of Kang et al. (2018). The stock solution comprised 7.4 mM ABTS (Sigma-Aldrich) and 2.5 mM potassium persulfate solutions. The mixture was prepared by mixing the two stock solutions at a ratio of 1:1 (v/v) and allowed to react for 16 h in dark. The ABTS solution was diluted with tertiary dH2O to the appropriate absorbance (7.00±0.05), which was measured at 734 nm. The ABTS solution (180 μL) was mixed with 20 μL of yogurt extract, and the mixture was covered with aluminum foil and placed in the dark at room temperature for 30 min. The absorbance of the mixture was measured at 734 nm using an ELISA reader (Synergy 2; BioTek Instruments). Each sample was measured in triplicate, and percent inhibition was calculated using the following equation:
where, A is the absorbance at 734 nm.
Total phenolic content (TPC) was determined using the method of Wei et al. (2011) with modifications. Briefly, each yogurt extract (100 μL) was mixed with 100 μL of 1 N Folin–Ciocalteu reagent (Sigma-Aldrich); this mixture was thoroughly mixed and incubated at room temperature for 3 min. Na2CO3 (200 μL, 1 N) was added to the mixture, which was allowed to react at room temperature for 90 min. The developed color in the mixture was measured using an ELISA reader (Synergy 2; BioTek Instruments) at 725 nm. The TPC is expressed as gallic acid equivalent (Sigma-Aldrich). The regression line of the gallic acid standard was used to determine the TPC in yogurt extract as micrograms of gallic acid equivalent per milliliter (μg GAE/mL).
Total flavonoid content (TFC) was measured using the method of Abeysinghe et al. (2007) with modifications. Briefly, each yogurt extract (100 μL) was mixed with diethylene glycol (500 μL) and NaOH (50 μL, 1 N). The mixture was incubated at 37°C for 60 min. The developed color in the mixture measured using an ELISA reader (Synergy 2; BioTek Instruments) at 420 nm. The TFC is expressed as quercetin equivalent (Sigma-Aldrich). A standard curve was generated using the quercetin standard solution, and the results are expressed as quercetin equivalent per milliliter.
The human colorectal cell line (HT-29) used in this study was purchased from the American Type Culture Collection (Manassas, VA, USA). HT-29 cells were cultured in RPMI 1640 medium (Lonza, Walkersville, MD, USA) supplemented with 10% fetal bovine serum (Atlas Biologicals, Fort Collins, CO, USA), 100 U/mL penicillin (Gibco, Grand Island, NY, USA), and 100 μg/mL streptomycin (Gibco) at 37°C under 5% CO2. The cells were re-fed with the medium every 2 d until 80% confluence.
ROS were measured using the stain 2’,7’-dichlorofluorescein diacetate (DCFDA, Sigma-Aldrich). Briefly, the cells were seeded in six-well plates, incubated overnight, and then treated with diluted yogurt extract for 16 h. Thereafter, the cells were treated with 1 μg/mL lipopolysaccharide (Sigma-Aldrich)-containing medium for 6 h. The cells were then incubated with DCFDA at a final concentration of 10 μM for 30 min and washed twice with cold PBS. The plates were subsequently measured using the fluorescence microscope (Model IX71, Olympus Optical, Tokyo, Japan). Photographs were captured using the Olympus DP71 camera and DP controller software (Olympus Optical). Finally, ROS were quantified using Image J software (National Institute of Health, Bethesda, Rockville, MD, USA).
α-Glucosidase activity inhibitory effect was determined using a modified assay reported by Kwon et al. (2006). Briefly, 50 μL of yogurt extract was added to 100 μL of 0.1 M phosphate buffer (pH 6.9) containing α-glucosidase solution (1 U/mL, Sigma-Aldrich) in 96-well plates. The plates were incubated at 25°C for 10 min. After pre-incubation, 50 μL of 5 mM p-nitrophenyl-α-D-glucopyranoside solution in 0.1 M phosphate buffer (pH 6.9) was added to each well. The mixture was incubated at 25°C for 5 min. The absorbance of the mixture was measured at 405 nm before and after incubation using an ELISA reader (Synergy 2; BioTek Instruments). The absorbance of yogurt extract was compared with that of the control, which was 50 μL of buffer solution. The α-glucosidase activity inhibition is expressed as percent inhibition and was calculated using the following equation:
where, A is the absorbance at 405 nm.
Pancreatic lipase inhibition was determined using the modified method of Kim et al. (2007) using p-nitrophenyl butyrate (NPB). Briefly, the enzyme solution was prepared by adding 30 μL of lipase solution from porcine pancrease Type VI-S (Sigma-Aldrich). This solution was composed of 10 mM morpholinepropanesulfonic acid and 1 mM ethylenediaminetetraacetic acid (pH 6.8) in 850 μL of Tris buffer (100 mM Tris-HCl and 5 mM CaCl2, pH 7.0). Yogurt extract (100 μL) was mixed with an enzyme substrate solution (880 μL) containing 10 mM p-NPB and incubated for 15 min at 37°C. The absorbance of the sample was measured at 400 nm using an ELISA reader (Synergy 2; BioTek Instruments) and was compared with that of the control buffer solution, without the extract. The lipase activity inhibition is expressed as percent inhibition and was calculated using the following equation:
where, A is the absorbance at 400 nm.
All data are expressed as mean±SD of three experiments. Statistical analysis of the data was performed using the one-way analysis of variance (ANOVA; SPSS 25, SPSS, Chicago, IL, USA) followed by Duncan’s multiple range test and Student t-test for comparison of means. Statistical significance was set at p<0.05.
Results and Discussion
The nutritional composition of yogurt supplemented with SPE and SPW is shown in Table 1. The moisture content of SPE yogurt increased with an increase in the concentration of SPE added. The moisture content of SPE yogurt was 81.67%–82.13%, which was significantly lower than that of plain yogurt (p<0.05). In SPE yogurt, the concentration of proteins, fats, lactose, and total solids increased with an increase in the concentration of SPE. The lactose concentrations in 0.5% and 1.0% SPE yogurt were 13.02% and 13.11%, which were significantly higher than those in 0% and 0.1% SPE (p<0.05). The concentration of nutrients in SPE yogurt tended to be higher than that in SPW yogurt. In particular, the protein concentrations in SPE yogurt were 4.33%–4.40%, which were significantly higher than those of SPW yogurt (p<0.05). The supplementation of yogurt with red ginseng extract changed the composition of yogurt, that is, with an increase in red ginseng extract concentration, the moisture content and protein and lactose concentrations increased (Jung et al., 2016). These findings are similar to our data. According to Pires et al. (2018), the supplementation of various plant petal extracts to yogurt marginally increased the nutritional composition such as protein and lactose concentrations, except the moisture content. Overall, yogurt composition was affected by the addition of SP extract.
As shown in Fig. 1A and 1D, the pH of SPE and SPW yogurt decreased significantly during fermentation (p<0.05). In contrast, the TA level in SPE and SPW yogurt was significantly increased during fermentation as shown in Fig. 1B and 1E (p<0.05). The pH and TA of all yogurt samples were similar during the initial and final stages of fermentation. These results are consistent with those of previous studies, that is, the pH decreased but the TA level increased due to acid production in red ginseng extract- and ginseng extract-supplemented yogurt (Jang et al., 2018; Jung et al., 2016).
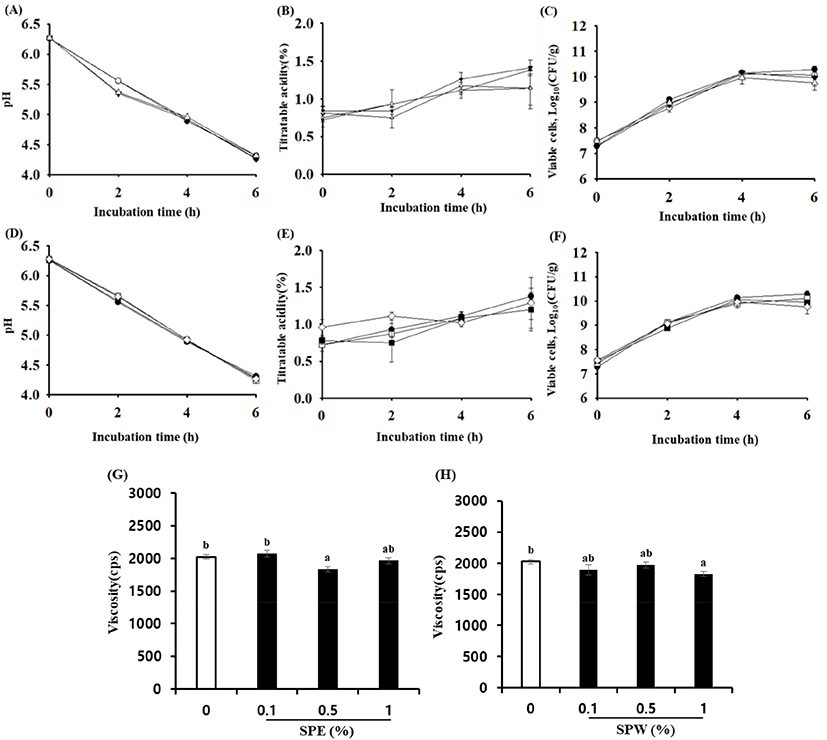
The viable cell counts in SPE and SPW yogurt during fermentation are shown in Fig. 1C and 1F. They ranged from 7.49 to 9.77 Log10 (CFU/g) and 7.43 to 9.99 Log10 (CFU/g), respectively. The number of viable cells in SPE was not significantly different from that in SPW yogurt. However, the number of viable cells in SPE and SPW yogurt was slightly lower than that in plain yogurt at the end of fermentation. It has been reported that in yogurt supplemented with cinnamon ethanol extract, the viable cell counts were marginally decreased at the end of fermentation (Choi et al., 2016). However, yogurt fermented with Korean traditional plant extracts showed an increase in lactic acid bacteria count during fermentation (Joung et al., 2016; Jung et al., 2016).
The viscosity of yogurt supplemented with SP extracts at different concentrations is shown in Fig. 1G and H. The viscosity of yogurt with SPE and SPW tended to be slightly lower than that of plain yogurt. The viscosity of 1.0% SPW and 0.5% SPE yogurt was significantly decreased compared with plain yogurt (p<0.05). It is known that viscosity is related to the aggregation of casein micelles (Joyce et al., 2017). Sung et al. (2015) reported that the viscosity of yogurt supplemented with 5% freeze-dried mulberry fruit juice was significantly lower than that of the control. Tseng and Zhao (2013) reported that the viscosity of yogurt supplemented with wine grape pomace was decreased; they suggested that this was probably because the addition of a high concentration of wine grape pomace into yogurt resulted in the breakdown of the coagulated milk. Furthermore, it has been reported that carthamin yellow from safflower significantly decreased whole blood viscosity, plasma viscosity, and erythrocyte aggregation index, in an animal study (Li et al., 2009).
As shown in Table 2, the DPPH radical-scavenging activity of SPW yogurt was 5.81%–10.66%, which was considerably higher than that of SPE yogurt, which was 1.03%–3.24%. Moreover, the ABTS radical-scavenging activity of SPW yogurt was 40.00%–49.67%, but the activity was not detected in SPE yogurt. These results are consistent with those of Kang et al. (2018), who reported that the antioxidant activity of yogurt supplemented with water extract of fermented pepper was higher than that of methanol extract. Here, the antioxidant activities of yogurt seemed to increase with the concentration of SPE and SPW. In particular, the antioxidant activity of 1.0% SPW yogurt was the highest, and it was significantly higher than that of plain yogurt (p<0.05). These results can be attributed to the TPC and TFC in the SP. Similar to the antioxidant effects, the TPC and TFC in yogurt increased with the increase in the concentration of SPW and SPE. The TPC and TFC in yogurt supplemented with SPW 1.0% were 393.13 and 320.90 μg/mL, respectively, which were the highest among the yogurt samples (p<0.05). The TPC in yogurt supplemented with SPE and SPW was 93.55–115.12 and 282.52–393.19 μg/mL, respectively, and the TFC was 52.53–77.76 and 180.97–320.90 μg/mL, respectively. It is known that edible safflower yellow pigments, which belong to the C-glucosylquinochalcone family of flavonoids, are water soluble (Li et al., 2018). This suggests that hot water extracted more bioactive substances such as polyphenols and flavonoids from SP, in this study. Kruawan and Kangsadalampai (2006) reported that the water extract of Carthamus tinctorius L. presented high antioxidant activity and TPC. In summary, the results demonstrated that SP extracted with hot water had greater antioxidant capacity, as evidenced by an increase in the TPC and TFC.
The effect of SPE yogurt on intracellular ROS levels was determined in order to elucidate its antioxidant activity, through which SPE can protect cells against LPS-induced damage. LPS, a major component of bacterial cell walls, induces the production of inflammatory mediators such as ROS (Lee et al., 2014). ROS play an important role in oxidative stress associated with the development of chronic diseases such as cancer and type II diabetes (Lee et al., 2014). Therefore, this study was focused on LPS-induced ROS production in vitro. As shown in Fig. 2, SPE and SPW yogurt significantly reduced ROS production in LPS-induced HT-29 cells (p<0.001). Ma et al. (2016), based on their study in LPS-induced hepatocellular (HepG2) cells, reported that SP yellow B possesses a high ROS-scavenging activity. As mentioned above, edible safflower yellow pigments are water soluble, and therefore, the ROS-scavenging ability of SPW yogurt with a high TPA and TFC was higher than that of SPE yogurt. Thus, these results demonstrated the antioxidant effect of SP extract-supplemented yogurt in vitro.
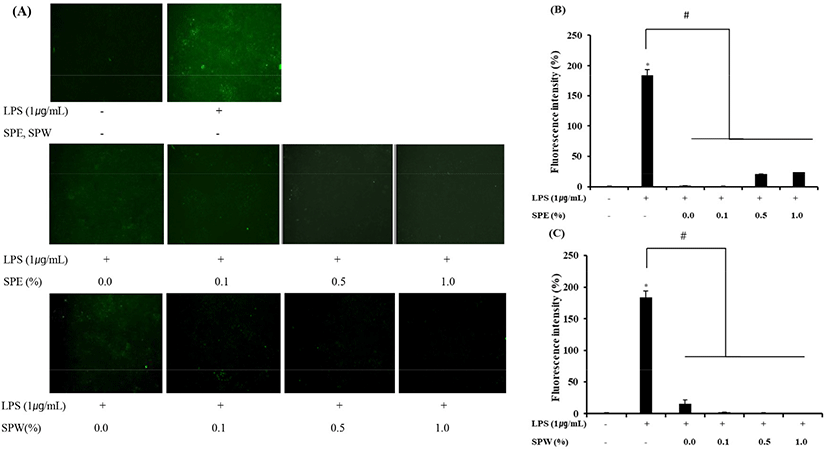
It is well known that α-glucosidase and pancreatic lipase are involved in the digestion and absorption of carbohydrates and lipids, respectively (Buchholz and Melzig, 2016; Elbashir et al., 2018). In this study, the activities of these enzymes were measured to determine whether SP could regulate enzymes related to diabetic complications such as hyperglycemia and hyperlipidemia. As shown Fig. 3B, SPW yogurt significantly inhibited the α-glucosidase activity in a dose-dependent manner (p<0.05). Furthermore, the inhibitory effect of SPW yogurt on pancreatic lipase activity was the highest at a concentration of 0.5% (Fig. 3D). However, these enzyme inhibitory effects of SPE yogurt were significantly lower than those of plain yogurt, except the α-glucosidase activity inhibitory effect of 0.1% SPE yogurt (Fig. 3A and C; p<0.05). The α-glucosidase activity inhibitory effect of 0.1% SPE yogurt was significantly increased compared with yogurt samples with other concentrations of SPE (p<0.05). Several studies have suggested that various plants inhibit the activities of α-glucosidase and pancreatic lipase (Elbashir et al., 2018; Gholamhoseinian et al., 2008; Yoshioka et al., 2019). Elbashir et al. (2018) suggested that the strong α-glucosidase activity inhibitory effect of these plants is related to their antioxidant capacities due to the presence of bioactive compounds including polyphenols, flavonoids, and tannins. In this study, SPW yogurt had more TPC and TFC, and showed higher free radical-scavenging activities than SPE yogurt. Therefore, SP extract-supplemented yogurt might alleviate hyperglycemia and hyperlipidemia by downregulating the digestion and absorption of carbohydrates and lipids.
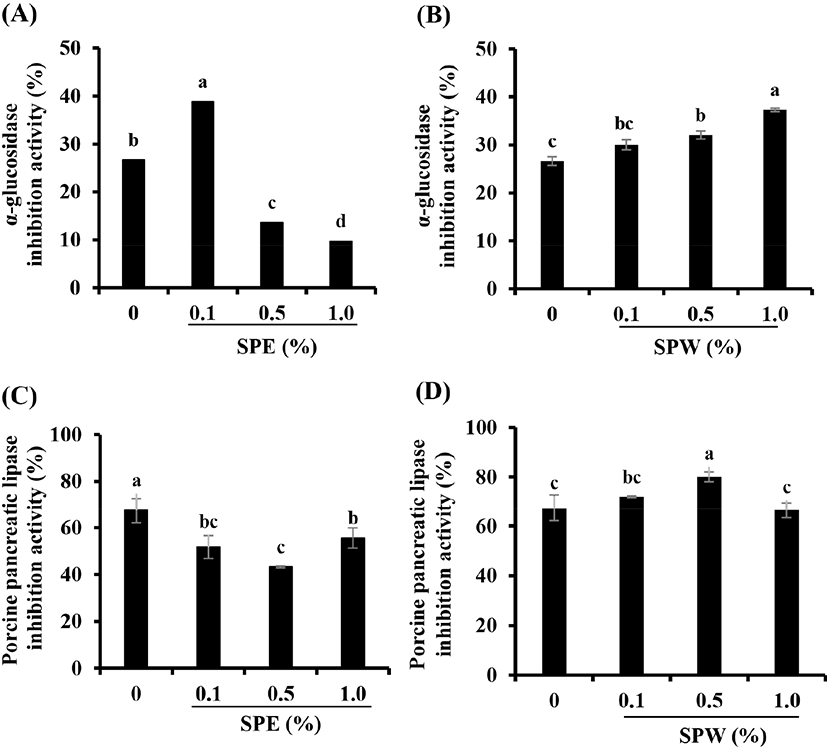
Taken together, the number of viable LAB in safflower yogurt was similar to that the plain yogurt, which was more than Korea Food Standard (1×107 CFU/mL) and the pH was 4.26–4.31, which were similar to that of the plain yogurt (pH 4.3). These results indicated that SP extract-supplemented yogurt had no negative effects on yogurt characteristics compared with plain yogurt. The addition of SP extract resulted in an increase in the TPC and TFC, which increased the antioxidant activity of SP extract-supplemented yogurt. The inhibitory effect on enzymes that are involved in carbohydrate and lipid digestion and absorption resulted in a positive effect on the regulation of hyperglycemia and hyperlipidemia. In addition, although the changes in the storage characteristics of SP extract-supplemented yogurt were not evaluated in this study, they were considered stable because there were no significant negative changes in the quality of yogurt stored at 4°C for 21 d. In conclusion, SP extract has potential application in the production of high value-added products, as it can enhance health benefits of various foods including yogurt. Further animal studies are needed to confirm the action mechanisms and in vivo effects to validate the potential benefits of yogurt supplemented with SP extract in managing weight and blood glucose.