Introduction
Historically, many insects, especially those belonging to the orders Coleoptera, Hymenoptera, Orthoptera, and Lepidoptera have been used as food by humans and animals, notably in Africa, Asia, Australia, and the Americas (Pemberton 1999; Siemianowska et al., 2013).
There has been a growing concern on the use of insects as an aleternative food and an animal feed ingredient. Unsaturated fatty acids, crude proteins, minerals, vitamins, and carbohydrates from insects are more sustainable food sources than animal protein; therefore, they are considered as alternatives to animal-based protein or carbohydrate products (Alamu et al., 2013; Oonincx et al., 2012; Raubenheimer et al., 2013).
Recently, several attempts have been made to identify various insect resources as useful biological resources and utilize them for different purposes. According to the Food and Agriculture Organization (FAO), two billion people worldwide consume insects and the use of insects as food will provide economic benefits when insects are used as a substitute for animal protein (Noh et al., 2015). However, it is important to establish the safety of insects for human consumption. Accordingly, toxicity evaluations for factors such as dyspepsia, nutritional losses, allergic reactions, tumorigenic metabolites, and toxic effects, must be conducted (Awoniyi et al., 2004; Téguia et al., 2002).
Locusta migratoria [Orthoptera: Acrididae (Linnaeus, 1758)], migratory locust, was reported to feed on grass and often causes serious damage to agricultural crops (Tanaka et al., 2012). L. migratoria, Oxya chinensis sinuosa, and some species of the order Orthoptera have long been known to purportedly effective for the management of asthma, bronchitis, stroke, and Alzheimer’s disease (Kim et al., 2015; Lee et al., 2018), and research has been conducted on the anti-inflammatory and cellular protective effects of O. chinensis sinuosa (Park et al., 2006; Yoon et al., 2014). Studies have also been conducted on the nutritional components and antioxidant (Kim et al., 1987) and antibacterial effects (Kim et al., 2015) of L. migratoria. The effects of L. migratoria extract have been confirmed through several studies, and in vitro experiments have shown that the extract has anti-inflammatory, neuroblastogenic, and osteoblast differentiation effects (Baek et al., 2018; Lee et al., 2018). These investigations have contributed data for elucidating the beneficial effects of L. migratoria, similar to those of other Orthoptera orders.
Ethanol extract of L. migratoria has been investigated for its anti-inflammatory effects on BV-2 microglial cells (Lee et al., 2018), inhibitory effect on osteoblast differentiation (Baek et al., 2019), and osteoblastogenic activity (Baek et al., 2018). In addition, the nutritional value of L. migratoria as a novel food has been established (Clakson et al., 2018; Mohamed 2015a; Mohamed 2015b). With regard to the nutritional content of L. migratoria, 100 g of dry L. migratoria is comprised of 50.42±2% crude protein, 19.62±0.8% crude fat, 4.78±0.74% carbohydrates, 15.65±1.7% crude fiber, 6.24±0.5% ash, and 3.8±0.2% moisture (Mohamad, 2015a). The fatty acid content includes the saturated fatty acids: palmitic acid, and stearic acid, and the unsaturated fatty acids: oleic acid, linoleic acid, and omega-6 and omega-3 fatty acids (Mohamad, 2015b).
While toxicity evaluations similar to those conducted in this study have been performed for other edible insects such as Tenebrio molitor (Han et al., 2014), Allomyrina dichotoma (Noh et al., 2015), and Gryllus bimaculatus (Lee et al., 2016), few studies have evaluated the toxicity of L. migratoria. Therefore, some safety evaluations conducted in this study were performed to according to international test guidelines (OECD, 2008). In the aforementioned studies, no toxicity was observed with the consumption of T. molitor at a dose of <3,000 mg/kg/d (Han et al., 2014); A. dichotoma at 2,500 mg/kg/day (Noh et al., 2015); and G. bimaculatus at less than 1,000 mg/kg/day (Noh et al., 2015). T. molitor and A. dichotoma were confirmed to be associated with subchronic oral dose toxicity for 90 days in 5-week-old SD rats (Han et al., 2014; Noh et al., 2015), and G. bimaculatus was confirmed to be associated with subacute oral dose toxicity for 28 days with 5-week-old SD rats (Lee et al., 2016).
However, despite the various health benefits of edible insects, there has been limited research on their safety for human consumption. It has been reported that cross allergy L. migratoria reactions occur in fly-allergic patients and house dust mite-allergic patients (Pali-Schöll et al., 2019). Common foods such as milk, eggs, and peanuts are known to cause allergies, but these reactions reportedly did not apply to all people (Longo et al., 2013). Although there have been no papers describing oral toxicity studies for L. migratoria, genotoxicity was not observed in terms of the micronucleus, sister chromatid exchange, and structural chromosome aberration assays when samples were treated with human blood cells at a concentration of 0 to 1,000 mg/dL, based on the results of a genotoxicity test (Turkez et al., 2012). In this paper, since genotoxicity had not yet been confirmed at 3,000 mg/kg, it will be necessary to proceed with the longer 90-day repeated-dose study. The long-term safety for human application, including potential hypersensitivity and genotoxicity assay, has also not been evaluated. Therefore, it is suggested that food safety will be improved if tissues of L. migratoria that cause allergic reactions are removed prior to use as a food.
The present study aimed to evaluate the safety of L. migratoria for human food and animal feed via 28 days repeated-dose oral toxicity tests using Sprague-Dawley (SD) rats administered freeze-dried L. migratoria (fdLM) under the Good Laboratory Practice regulations (GLP) of the Organization for Economic Cooperation and Development (OECD) and the Korea Ministry of Food and Drug Safety (MFDS).
Materials and Methods
The preparation of fdLM was supported by the National Institute of Agricultural Sciences (Wanju, Korea). L. migratoria were allowed to grow under the following conditions in a plastic breeding box (27 cm×36 cm×8 cm) for 2 d at the Industrial Insect Division, National Institute of Agricultural Sciences: temperature of 26±2°C, 65% relative humidity, and 12/12-h light:dark cycle. The host plants of L. migratoria include corn leaves and wheat leaves. In this experiment, L. migratoria feeding on corn leaves were raised indoors, as an experimental sample. The L. migratoria adults were feed with 120 cm long (from leaf base) corn leaves. Before the preparation of the fdLM samples, the adult of L. migratoria were fasted for 1 d; fecal debris was separated and washed twice with flowing water and dried. The separated insects were autoclaved at 115°C for 10–15 min in a steam sterilizer (Tomy Kogyo, Tokyo, Japan), and then maintained at –70°C in an ultra-low temperature freezer (NIHON freezer, Tokyo, Japan) for more than 24 h to prevent the destruction and changes to the cell structure. The sterilized L. migratoria sample was rapidly frozen at ≤–35°C for 12 h or more to maintain the suitability of samples by preventing food deterioration due to temperature changes and to operate the freeze dryer properly. Subsequently, the samples were dried at 36.1°C for approximately 60–72 h in a vacuum of 70 torr or less and dried slowly (IlshinBioBase, Dongducheon, Korea). The L. migratoria powder was prepared by pulverizing the insects using a mesh mill (Garyeo Industry, Siheung, Korea).
SD rats have been widely used in general toxicity tests as a suitable experimental animal for toxicity testing. 40 specific pathogen-free Crl:CD SD rats of both sexes (20 males and 20 females) were randomly assigned to four groups. No more than 3 animals were housed per wire mesh cage (250 W×350 L×180 H mm) during the quarantine, acclimation, administration, and observation periods. The cages were replaced at the grouping stage. Environmental parameters were regulated to maintain the following conditions: temperature, 24.1±0.7°C; relative humidity, 48.1±4.4%; ventilation, 10–15 air changes/h; luminous intensity, 287 Lux; and a 12/12-h light:dark cycle. Sterilized, solid laboratory animal feed (Teklad Certified Irradiated Global 18% Protein Rodent Diet, Envigo, Indianapolis, IN, USA) was provided by DooYeol Biotech (Seoul, Korea). Tap water purified using a reverse osmosis filtering system was supplied to the animals. Contamination inspections were conducted in compliance with the standard operating procedure of the testing facility. The animals were fed ad libitum through a stainless steel feeder daily. Water was supplied ad libitum daily using polycarbonate bottles. Cages, cage racks, feeders, and water bottles were replaced at the grouping stage. This study was conducted in compliance with the OECD Guidelines for Testing of Chemicals, Section 4. Health Effects, No. 407, Repeated-Dose 28-Day Oral Toxicity Study in Rodents and approved by the Institutional Animal Care and Use Committee (IACUC) of the Korea Conformity Laboratories (KCL).
The dosage of the test substance (freeze-dried powder of Locusta migratoria, fdLM) was determined based on the results of the preliminary toxicity test. The upper dose limit was set based on the maximum amount that can be technically administered. Thus, the maximum dose for both sexes was set to 3,000 mg/kg/day and two lower doses were added in a halving ratio from the 3,000 mg/kg/day at 1,500 and 750 mg/kg/day. Vehicle (distilled water for injection) or graded doses of fdLM (750, 1,500, and 3,000 mg/kg of body weight) were administered to 5-week old SD rats by oral gavage once daily for 28 d at a dose of 20 mg/kg of body weight after completion of a 14-day repeated Dose Range Finding (DRF) study. The test substance was administered by gavage at the dose levels of 750 (low-dose), 1,500 (middle-dose), and 3,000 (high-dose) mg/kg/day in the treatment groups and findings were compared with the vehicle control group. The toxicity of the test substance was compared between the groups. The rats were re-fed pellet food approximately 4 h after administration of the test substance. During the study, animals were observed for mortalities, daily clinical signs, and body weight changes. After termination of observation, gross findings at necropsy were examined. Toxicological assays were performed to evaluate safety of fdLM as an edible insect in a 14-day repeated-dose oral toxicity study in compliance with OECD guidelines under GLP regulations. For the 28-day oral toxicity study, 5-week old healthy male and female SD rats were assigned to 4 groups (5 rats/sex/group) as per GLP regulations and in accordance with the OECD Guideline 407 (2008). Vehicle (distilled water) or graded doses of fdLM (750, 1,500, and 3,000 mg/kg of body weight) were administered to rats by oral gavage once a day for 4 wk at a dose of 20 mL/kg of body weight. The rats were monitored daily for clinical signs including behavioral abnormalities, general appearance, and mortality until terminal sacrifice. The body weights and food/water consumption were recorded weekly, and at the termination of study, the rats were euthanized via isoflurane (2% to 5%) inhalation for blood sample collection.
Weights of individual animals were monitored once per week at acquisition, grouping, and prior to test substance administration during the study, and also immediately before necropsy. The animals were weighed in the morning. Food consumption was measured right before the first test substance administration and once per week during the study. To calculate food intake per day, the food ration for each cage was weighed the day before the body weight measurement and the residual quantity was measured on the day of the body weight measurement. Food consumption per animal was recalculated according to the average consumption (g/rat/day) for each individual animal. Water consumption was measured immediately before the first test substance administration and once per week during the study. The measurement and calculation of water intake per animal were performed as mentioned previously herein for food consumption measurements. Both eyes of all animals were examined macroscopically in the last week of treatment.
Urine samples were collected from 5 male and 5 female rats from each group in the last week of administration. The animals were individually placed in metabolic cages (Techniplast, Buguggiate, Italy), and approximately 1 mL samples of fresh urine were collected for urinalysis. The collected urine samples were analyzed using urine test strips (Multistix 10SG, Siemens, Germany) and urine auto-analyzer (Clinitek Advantus, Siemens, München, Germany). The following parameters were analyzed: glucose (GLU), bilirubin (BIL), ketone bodies (KET), specific gravity, occult blood (BLO), pH, protein (PRO), urobilinogen (URO), and nitrite (NIT).
After fasting overnight with water provided ad libitum, blood was collected from SD rats of both sexes. On the necropsy day, the animals were euthanized under inhalation of anesthesia with isoflurane. Blood samples were drawn from the abdominal aorta using a syringe and collected in tubes containing EDTA-K2 as anticoagulant for complete blood count and in serum-separating tubes (BD Microtainer, Franklin Lakes, NJ, USA). Whole blood samples collected in the EDTA-K2 tubes were analyzed using a hematology analyzer (ADVIA 2120, SIEMENS, München, Germany). The following hematological parameters were analyzed: white blood cell (WBC), neutrophil (NE), eosinophil (EO), basophil (BA), lymphocyte (LY), monocyte (MO), large unstained cell (LUC), percent of neutrophil (NEP), percent of eosinophil (EOP), percent of lymphocyte (LYP), percent of monocyte (MOP), percent of large unstained cell (LUP), red blood cell (RBC), hemoglobin (HB), red cell distribution width (RDW), hematocrit (HCT), mean corpuscular volume (MCV), mean corpuscular hemoglobin (MCH), mean corpuscular hemoglobin concentration (MCHC), reticulocyte (Reti), platelet (PLT), and mean platelet volume (MPV).
Blood serum was obtained by centrifuging the collected blood sample in a serum-separating tube at 800×g for 10 min. Blood biochemistry analysis was performed using a biochemistry analyzer (Hitachi7180, HITACHI, Tokyo, Japan). The following serum biochemistry parameters were also measured: aspartate aminotransferase (AST), alanine aminotransferase (ALT), alkaline phosphatase (ALP), bilirubin (BIL), blood urea nitrogen (BUN), albumin (ALB), uric acid (UA), creatinine (CRE), cholesterol (CHO), glucose (GLU), triglyceride (TG), protein (PRO), albumin/globulin ratio (A/G), creatine phosphokinase (CPK), lactate dehydrogenase (LDH), calcium ion (Ca+), inorganic phosphorus (IP), magnesium ion (Mg+), potassium ion (K+), sodium ion (Na+), and chloride ion (Cl–).
After the termination of test substance administration, scheduled necropsy was performed for all surviving animals and complete postmortem examinations were conducted on all organs. At necropsy, the ovaries, uterus, testes, spleen, liver, adrenal glands, kidneys, heart, lungs, brain, pituitary gland, and thymus were extracted and their weights were measured using an electronic balance. The testes were fixed in Böuin solution. The remaining extracted organs were fixed in 10% neutral phosphate-buffered formalin. The absolute weights of the organs were measured using an electronic balance, and the relative weights were calculated based on the fasting body weight before necropsy.
The liver and kidney were removed after observing gross lesions in all experimental animals administered the sample material on the final necropsy day. The organs were then embedded in paraffin. Sections (3–4 μm) were deparaffinized and the slides were dehydrated, with a wash for each step as follows: xylene, 100% ethanol, 95% ethanol, 70% ethanol, and distilled water. They were then stained with hematoxylin and eosin (HE) for histopathological examination. Next, the sections were washed under running distilled water for 10 min, dehydrated, mounted, and examined by light microscopy.
All data are presented as mean±SD. The vehicle control and fdLM dosing groups were analyzed by one-way analysis of variance (ANOVA) to determine statistical significance. Statistical differences between the groups were analyzed using parametric or non-parametric multiple comparison tests. Incidence rates were presented as percentages. Statistical analyses were performed in compliance with the standard operating procedure of the testing facility, and SPSS for Windows, version 12.0 (SPSS, Chicago, IL, USA) was used. When the statistical results showed significance, a post-hoc comparison test was performed. If equal variance was noted, Duncan’s test was used, and if not, Dunnett’s T3-test was applied. Statistical significance was analyzed using SPSS software and was only concluded to be significantly different if the p-value was less than 0.05.
Results and Discussion
Repeated-dose toxicity study of fdLM at doses of 750, 1,500, and 3,000 mg/kg/day administered by oral gavage for 14 days was carried out to assess toxicity, evaluate the approximate lethal dose (LD), and provide a rationale for dose selection levels for a subsequent 28-day repeated-dose oral toxicity study. As a result of the study, no test article-related changes in mortalities and unusual clinical signs were observed during the 14-day treatment period (body weights in Fig. 1; other data not shown). In body weight, decreases were observed in 1 of 5 females in the vehicle control group on Day 3 (Fig. 1). However, the change was a mild and temporary as shown in the vehicle control group (Fig. 1). The changes in the body weights level were within the normal range reported by Giknis and Cliford (2006). Therefore, the changes were considered to be an incidental changes not related to the test substance. In necropsy at the termination of observations, no abnormal gross findings were observed in all animals (data not shown).
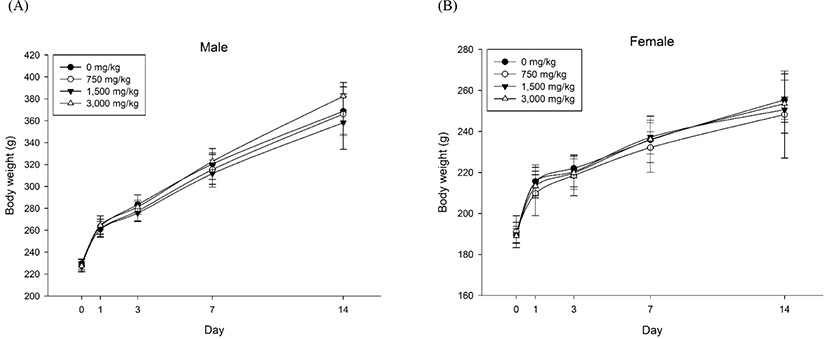
In conclusion, among fdLM concentrations tested, 3,000 mg/kg/day was selected as the high dose for the 28-day repeated-dose oral fdLM toxicity study. As a result, fdLM did not induce toxicity in rats and the approximate lethal dose was greater than 3,000 mg/kg (data not shown). In addition, no toxicological changes were observed in the 14-day repeated-dose oral toxicity studies. Preliminary examination after single oral administration of test substance to each male and female rat at doses of 1,500 and 3,000 mg/kg showed no remarkable general symptoms and weight changes (Fig. 1).
Body weight gains and feed and water intake are considered normal changes within the biological variation range. It was also judged that there was no influence of the test substance because no specificity was confirmed in the observation of clinical symptoms, including fundus examination. Intergroup differences in body weight (Fig. 2) and food consumption during the experimental period were not significant. There were no significant differences in body weight and food consumption among the groups (data not shown). Furthermore, with regard to water consumption, intergroup differences during the experimental period were not significant. The ophthalmologic examination revealed no abnormalities in any of the animals tested (data not shown).
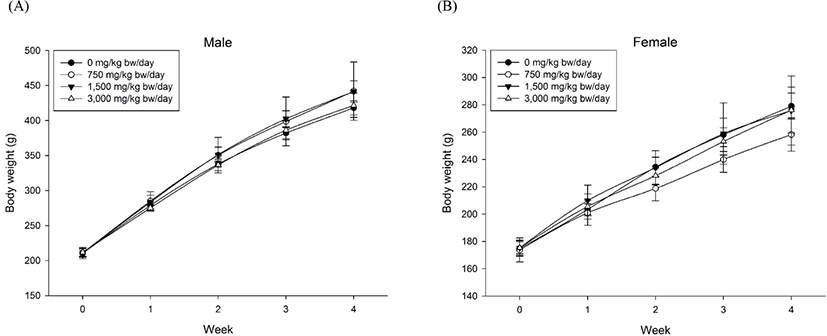
Intergroup differences in the urinalysis results were not significant (Table 1). Regarding hematological tests, the percentages of BA and MOP decreased in the males at 1,500 mg/kg/day and females at 750 mg/kg/day dosing groups (Table 2). However, the changes were mild and within the historical biological ranges; furthermore, they did not show a distinct dose-response correlation.
fdLM, freeze-dried powder of Locusta migratoria; WBC, white blood cell; NE, neutrophil; EO, eosinophil; BA, basophil; LY, lymphocyte; MO, monocyte; LUC, large unstained cell; NEP, percent of neutrophil; EOP, percent of eosinophil; LYP, percent of lymphocyte; MOP, percent of monocyte; LUP, percent of large unstained cell; RBC, red blood cell; HB, hemoglobin; RDW, red cell distribution width; HCT, hematocrit; MCV, mean corpuscular volume; MCH, mean corpuscular hemoglobin; MCHC, mean corpuscular hemoglobin concentration; Reti, reticulocyte; PLT, platelet; MPV, mean platelet volume.
BA is present in very small amounts in rats (Giknis and Clifford, 2006). BA represents <1% of the peripheral blood leukocytes in the peripheral blood and is often recruited to the site of allergic inflammation (Dvorak and Mihm, 1972; Iliopoulos et al., 1992; Irani et al., 1998; Macfarlane et al., 2000; Nouri-Aria et al., 2001). Increase in BA levels is seen in the immune response against parasites and are involved in the allergic disorders or in immunity to pathogens (Min et al., 2004; Mitre et al., 2004; Roth et al., 1980; Voehringer et al., 2004).
Although BAs were not detected in all males, BA levels increased slightly in male rats treated with 1,500 mg/kg/day of fdLM. Therefore, although statistics were processed, these data suggested that the changes were not treatment-related. However, the level of BA varies enormously between infected rats and an increase in BAs is not always statistically significant at <5% based in other research (Ogilvie et al., 1980).
As a result of the BA increase in a small number of the male rats administered 1,500 mg/kg/day in this study, the nutritional value and safety aspects of fdLM need to be further studied. This is because food allergy is defined as a side effect that occurs from the immune response (Kojima et al., 2007).
The serum biochemistry analysis revealed significantly lower IP levels in females in the 1,500 and 3,000 mg/kg/day dosing groups than in the vehicle control group (p<0.01; Table 3). However, the changes in the IP levels (medium dose group, 6.6 mg/dL and high dose group, 6.7 mg/dL) did not show a clear dose-response relationship. They were also within the normal range of accumulated historical data of the test institution (5.8–8.9 mg/dL; 95% confidence interval) and the report of Giknis and Cliford (2006), and, therefore, were not considered not to be treatment-related. In this study, the change in the normal range level and the absence of the dose-response correlation means that altered IP levels were considered a symptom was considered to have occurred spontaneously and without toxicological significance. However, we are planning a longer-term test (13-week repeated-dose study) and it is necessary to carefully observe these results regarding these parameters and findings.
fdLM, freeze-dried powder of Locusta migratoria; AST, aspartate aminotransferase; ALT, alanine aminotransferase; ALP, alkaline phosphatase; BIL, bilirubin; ALB, albumin; BUN, blood urea nitrogen; CRE, creatinine; UA, uric acid; GLU, glucose; CHO, cholesterol; TG, triglyceride; PRO, protein; ALB, albumin; A/G, albumin/globulin ratio; LDH, lactate dehydrogenase; CPK, creatine phosphokinase; Ca, calcium ion; IP, inorganic phosphorus; Mg, magnesium ion; Na, sodium ion; K, potassium ion; Cl, chloride ion.
In many studies, it is known that the depletion of IP causes hyperuricemia and renal tubular dysfunction (Haut et al., 1980). The depletion of inorganic phosphorus is a critical cause of both ATP (adenosine triphosphate) and TAN (total adenine nucleotide), with higher fructose (Morris et al., 1978). In normal rats, an increase in phosphate intake is known to cause renal disease, and inadequate phosphate intake is known to induce renal injury (Haut et al., 1980). Also, IP is present in trace amounts in rats, and its value is related to Ca, ALP, and hormones (Mukaiyama et al., 2015; Ostrowska et al., 2003). However, based on the results of this study, there were no changes in Ca and ALP. Although IP is judged to be mildly influenced by the test substance due to slight decrease observed, it does not seem to have any toxicological significance. Previous studies have shown that an increase in blood IP may be a risk factor for chronic heart disease including cardiovascular outcomes, artery calcification, congestive heart failure and hyperphosphatemia (Campos-Obando et al., 2018; Olanbiwonnu and Holden, 2018). No other significant differences were observed between the vehicle control and dosing groups. In addition, no treatment-related microscopic or macroscopic findings were detected in the treated animals.
Absolute organ weights are shown in Table 4. Organ weight measurements indicated lower prostate weights (absolute and relative) in the males in the 3,000 mg/kg/day dosing group. The decreases in both the absolute and relative weights were significant compared to those in the vehicle control group (p<0.05; Table 4). There were no other significant differences between the vehicle control and dosing groups. Many factors influence prostate weight, including inter-animal viability, stress, and physiological factors unrelated to treatment; further, prostate weight changes are often unrelated to histopathological findings (Michael et al., 2007).
The changes in the prostate weight were within the historical biological range and did not indicate a clear dose-response correlation. Therefore, the changes were not considered to be treatment-related. Furthermore, the changes were within the normal range reported by Giknis and Cliford (2006). Necropsy revealed no abnormal gross findings in any animal.
In histopathological examination, liver and kidney tissues from all administered animals were assessed by HE staining. All SD rats treated with fdLM showed normal liver architecture based on major histological structures such as hepatocytes, portal triad, and central vein. (Fig. 3). Kidneys also showed normal architecture in tubules and glomeruli, which are functionally important structures (Fig. 4). All SD rats treated with fdLM showed no lesions such as inflammation, necrosis, iron deposition, and bilirubin deposition (Figs. 3 and 4). No lesions that could cause any kind of structural and functional abnormalities were observed including inflammation, necrosis, fibrosis, and iron and bilirubin deposition (Figs. 3 and 4). There were no differences in histopathological examinations when compared to those in the control group. Moreover, from a histological point of view, the liver and kidney maintained their normal structure. This indicates that the organs were functioning well and suggests that the test substance had no effect on these organs, and normal structures were maintained in each cell. Therefore, pathological histological examinations showed no significant changes in the liver and kidney in the control and fdLM-administered groups, or any specific dose-dependent histological abnormalities due to administration of the test substance. These above findings were considered consistent with long-term weight changes and blood biochemical changes.
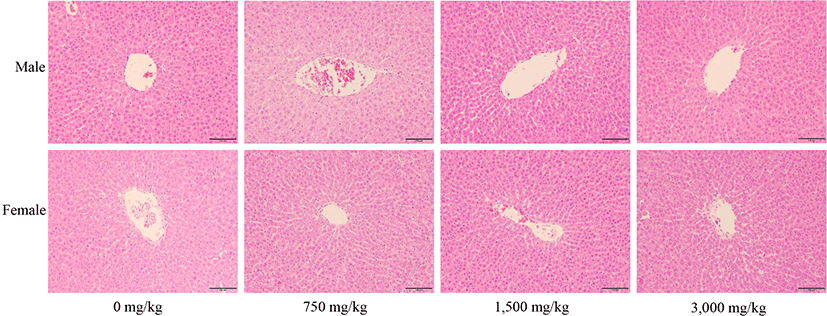
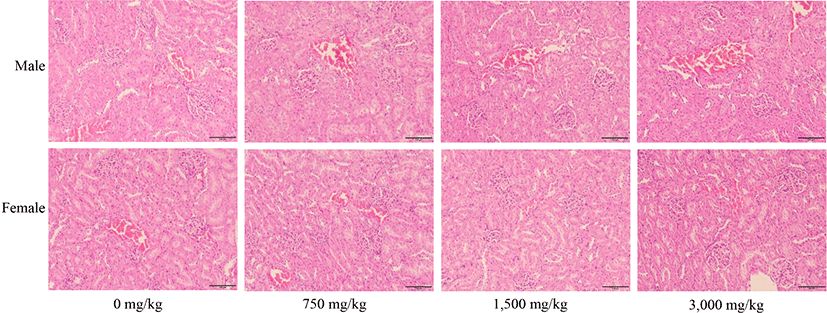
Conclusion
This research was performed to evaluate repeated-dose oral toxicity and no-observed-adverse-effect-level (NOAEL) for the test substance, fdLM, when administered for 28 d to SD rats and to determine the appropriate dose for a 90-day repeated-dose oral toxicity study in the same model. The test substance was administered by gavage at doses of 750 (low-dose), 1,500 (intermediate-dose), and 3,000 (high dose) mg/kg/day in the treatment groups, which were compared with the vehicle control group. There were no unusual clinical signs and mortalities in any of the treated groups during the experimental period. Although some significant differences in clinical chemistry and organ weights were reported in the treatment groups, the changes were considered incidental because they were inconsistent between the sexes or indicated no dose-response relationships. Additionally, the microscopic findings were either common findings in rats or unrelated to both the control and treatment groups (Mclnnes, 2012). The NOAEL was set to be higher than 3,000 mg/kg/day for both sexes of SD rats under the experimental conditions of this study. No toxicity has been observed in trials to date. It is thought that potential toxicity of the test substance can be observed through additional repeated doses in a 90-day oral dose toxicity test. Therefore, it was considered acceptable to set the high-dose level as 3,000 mg/kg/day for our upcoming 90-day repeated-dose oral toxicity study in the same model to investigate the safety of fdLM for human and animal food.