Introduction
Edible insects are attracting increasing interest in the food industry because of their potential to serve as an alternative protein source. Although insects have been eaten in many cultures for centuries, Western societies are only recently adopting such traditions. In many parts of the world, such as Africa, Asia, and Latin America, where insects are part of the diet, they are considered both a delicacy and a nutritional requirement (van Huis et al., 2013). According to the World Health Organization (Fishman et al., 2002), approximately 27% of children under 5 years of age are experiencing undernutrition stemming from the lack of adequate protein access. In these underdeveloped areas food security is prominent and entomophagy plays a key role as a solution.
As a food source, insects are not only comparable in nutritional value to conventional meat but also have many advantages in a variety of areas (van Huis et al., 2013). In terms of the environment, insect rearing produces far fewer greenhouse gases, requires clearing of less land, and requires less feed per unit of protein produced (12 times less than cattle), due to insects’ cold-blooded nature (van Huis et al., 2013). Along with their rapid growth, efficiency, and availability (over 1900 known edible species), insects show promise for use in sustainable agriculture (van Huis et al., 2013).
Although in Western societies insects are thought of as bothersome pests, research has shown that they display high availability of protein, lipid, vitamin, and mineral contents (Finke, 2002; Ghosh et al., 2017; Rumpold and Schlüter, 2013; Yi et al., 2013). All insects have a high abundance of amino acids (Ghosh et al., 2017; Melo et al., 2011; Yi et al., 2013; Zielińska et al., 2015), and in-depth analysis of individual insects provides further conclusions as well (Hall et al., 2017; Longvah et al., 2011; Zhao et al., 2016). Based on these studies, insects have been shown to contain a protein content ranging from 50-82% (dry weight). Compared to conventional protein sources such as beef, pork, chicken, and lamb, many insects, for example termites, grasshoppers, caterpillars, and houseflies, possess higher values (Rumpold and Schlüter, 2013).
Because insects possess physical and sensory characteristics that make them unattractive as food in Western culture, methods for extraction of protein for addition to other food have been tested, to increase their acceptance (Yi et al., 2013). Because insects show high lipid bioavailability that can interfere with protein extraction, lipid separation can be applied to enhance protein extraction. Although lipid separation of foods has been explored using a variety of materials for fat segregation, soybeans have been best studied (Karki et al., 2010; L'Hocine et al., 2006; Lou et al., 2010). Lipid extraction has been shown to not only isolate lipids specifically but also to improve certain functionalities and characteristics of proteins (L'Hocine et al., 2006; Lee et al., 2016). For edible insects, ethanol defatting has been applied to the larvae of yellow mealworm Tenebrio molitor (Zhao et al., 2016) for lipid determination, and hexane has been applied to a variety of insects (Longvah et al., 2011; Purschke et al., 2017; Yi et al., 2013) for comprehensive analysis. Although many alternatives to lipid extraction have been implemented (e.g. aqueous extraction, supercritical CO2), hexane defatting yields 96% or greater oil removal on average (Ricochon and Muniglia, 2010).
Ultrasound assisted extraction (UAE) or sonication is widely incorporated in food processing, as it provides an alternative extraction method to other heat or pressure treatments (microwave assisted, supercritical CO2, high-pressure processing). Ultrasound technology has seen an increase in utilization owing to its effectiveness and economic advantages (Preece et al., 2017). Ultrasound technology has been applied for protein extraction from a variety of foods including soy (Jambrak et al., 2009; Lee et al., 2016; Lou et al., 2010; Preece et al., 2017), whey protein concentrate (Chandrapala et al., 2011), and egg whites (Arzeni et al., 2012). Although Tzompa-Sosa et al. (2014) applied sonication technology to insects, no protein enhancement was noted; instead, it aided in lipid isolation when used as a pretreatment to the Folch and aqueous extraction procedures. To date no reports have been published on whether ultrasound technology is effective for extraction of protein from insects.
Edible insects have been studied as ingredients for formulation of meat and dairy products (Kim et al., 2016; Omotoso, 2006; Rumpold and Schlüter, 2013; Wang et al., 2017). Recently, Rumpold and Schlüter (2013) suggested application of edible insects in animal feed and human food products, with an emphasis on their use as a texturizing agent based on their high emulsion capacity. Kim et al. (2016) applied protein powders from edible insects in sausages, which increased cooking yield and hardness. Peptides extracted from silkworm pupae exhibited a positive effect on the quality of yogurt but require further research for application in dairy products (Wang et al., 2017).
The present study aimed to establish the optimum conditions for protein separation from three edible insects using ultrasound, for application in the food industry.
Materials and Methods
n-Hexane was of chemical grade and purchased from Honeywell Burdick & Jackson (Thomas Scientific, USA). The bovine serum albumin powder was purchased from Equitech-Bio, Inc. (USA).
Yellow mealworm larvae (Tenebrio molitor), field cricket adults (Gryllus bimaculatus), and silkworm pupae (Bombyx mori) were obtained from Insect Vision (Korea). The T. molitor and G. bimaculatus samples were previously microwave dried and the B. mori samples were frozen. The B. mori samples were freeze-dried following receipt in our laboratory, and all insect samples were immediately stored at −40°C until use. Prior to defatting, insect samples were ground into coarse meal using a traditional pestle and mortar.
Lipids were extracted from the insect samples using n-hexane as a solvent, at a solvent to sample ratio of 1:20. Samples were stirred for 12 h and the hexane was removed by filtering, then replaced every 12 h for a total time of 48 h. Samples were emptied onto aluminum foil and left to dry overnight under a fume hood. Total lipid content was determined using the Soxhlet extraction method(AOAC, 2000).
A Sonics® Vibra-Cell™ VCX 750 ultrasonic unit (Sonics & Materials Inc., USA) was used, with a maximum power output of 2.5 kW. It was operated at 20 kHz with a 75% AMP and pulsed every 3 s. Insects ground to a coarse meal (12.5 g) were mixed with 200 mL of distilled water containing 9.46 mM ascorbic acid. The suspension was then sonicated for 20 min and aliquots were collected at 1, 2, 5, 10, 15, and 20 min. The procedure was performed on ice and the sample was allowed to rest between intervals of equal time. Samples were sieved through a stainless-steel filter (pore size of 1 mm) and filtrates were collected and freeze-dried for further experiments. To determine protein amount and calculate protein yield, the samples were analyzed by the Dumas method using an NDA 701 Dumas Nitrogen Analyzer (Velp Scientifica, Italy). Protein yield (%) and total protein content of each insect sample were calculated based on protein quantity measured and reported as %N using the standard conversion factor of 6.25. The amino acid composition of the defatted insect samples was analyzed using an Agilent 1100 HPLC with an Eclipse AAA column (4.5 × 150 mm, 5 µm) from Agilent Technologies (USA).
Results
The lipid removal process was performed for all three insect samples, using conventional hexane defatting for 24-48 h (Fig. 1). Subsequent analysis of fat content, using the Soxhlet method, revealed crude residual fat values of less than 2.0% for all samples. The lowest average fat values, achieved after 48 h of defatting for mealworm, cricket, and silkworm samples, were 0.69%, 1.07% and 0.34%, respectively. No significant differences were observed after 36 h of defatting. Based on the values displayed in Fig. 1, the optimal time for defatting was 36 h.
Total protein content based on (%N) was highest in the cricket samples, which contained 74% protein after defatting. Silkworm pupae contained approximately 65%, while the mealworm showed the lowest protein content at just under 63% total protein.
Application of ultrasound sonication to the three insect samples was performed for various time periods, to a maximum of 20 min. The experiment was performed in triplicate and the averages are displayed in Fig. 2. Protein yield percentage was calculated based on the total protein amount of each insect. Although the yield showed a general increasing trend with time for all samples, a significant difference was observed between the silkworm pupae and the other two insects. After 20 min of sonication, the maximum protein yield of the silkworm pupae sample was 94%, 76% greater than its yield without sonication (18%, 0 min). After 5 min of treatment, the protein yield increased exponentially to 89%. Sonication for 20 min increased protein yield, but not significantly. Thus, for B. mori 5 min appears to be the most efficient period of sonication.
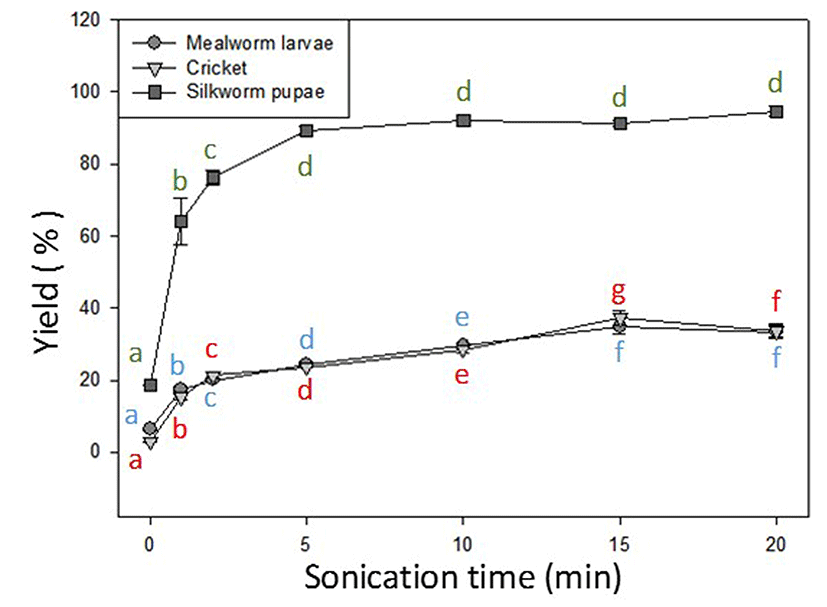
Mealworm and cricket samples showed a lower protein yield. Of the three insects, mealworm had the lowest protein yield at 35%; cricket had a yield of 37% (15 min). Both insect samples showed an increase in protein yield after 15 min of sonication, of 28% for mealworm and 34% for cricket. The highest values were obtained at 15 min for both the mealworm and cricket samples, and the slight decrease at the 20-min mark indicates that 15 min of sonication was sufficient for both samples.
The amino acid composition of each edible insect was determined (Fig. 3). The essential amino acids are the first eight shown on the x-axis and the following represent the non-essential amino acids. The three insects displayed similar profiles, with highest levels of glutamine and lowest levels of methionine. Levels of both proline and tyrosine, however, were notably high for the mealworm sample.
Discussion
Conventional hexane defatting was carried out for 24-48 h on mealworm, cricket, and silkworm samples. Our results showed average residual crude fat values of 0.75% for mealworm, 1.17% for cricket, and 0.47% for silkworm pupae. Our proximate composition analysis data for crude fat prior to defatting (33.46% for mealworm; 19.77% for cricket; 30.87% for silkworm pupae: data not shown) was comparable to the findings of others (Finke, 2002; Ghosh et al., 2017; Hall et al., 2017; Yi et al., 2013). In particular, our results corroborated those of Ghosh et al. (2017), who assayed 5 species of insects currently eaten in South Korea, including two of the three contained in our current study (T. molitor larvae, G. bimaculatus adults). A direct comparison of the yellow mealworm (Tenebrio molitor) sample was performed by Purschke et al. (2017) for extraction of oils with conventional hexane vs. supercritical CO2 (SCO2). Total defatting percentages (%) were 96.56% for n-hexane and 92.57% for SCO2 under conditions of 325 bars, 55°C, and 75 min. Although the SCO2 method produced a more rapid result, the energy output also needs to be considered, and the two methods yielded products with similar physio-chemical properties and fat composition (Purschke et al., 2017). We also applied SCO2 for defatting of mealworms, but only 30% of lipids were removed at 50°C (data not shown), which was not sufficient pretreatment for protein separation. Hexane defatting of mealworm showed equally high defatting percentages, but with a higher hexane to sample ratio. These findings coupled with our results showcase hexane’s efficiency to reduce the lipid content of insect samples.
Few studies have been published using insect samples, and soybean protein may serve as an alternate point of comparison. Comparable proximate analyses of soy protein including essential amino acid profiles (Yi et al., 2013) provide a point of reference for assessment. A study was conducted on the defatting of soybeans to compare different defatting solvents such as ethanol and methanol to hexane, due to reports of its effect on functionality and availability, as well as tighter emission restrictions and safety concerns (L'Hocine et al., 2006). Residual fat percentages in 100 g samples with a 1:3 solvent ratio were 1.4% for hexane, 3.8% for ethanol, and 14.5% for methanol.
The protein content detected on a dry matter basis after defatting of each insect ranged from 62% to 74%, with the cricket samples displaying the highest values (results not shown). Research on proximate nutritional content showed similar values for all insects (Ghosh et al., 2017; Longvah et al., 2011; Purschke et al., 2017; Rumpold and Schlüter, 2013; Yi et al., 2013; Zhao et al., 2016; Zielińska et al., 2015). On a dry weight basis, the protein content of Tenebrio molitor larvae ranged from 51% to 58% for non-defatted samples, while Yi et al. (2016) obtained a protein content value of 76.5% for n-hexane defatted mealworm samples after 6 h of treatment. Protein content of Bombyx mori pupae ranged from 48% to 58%, for nondefatted samples based on dry weight. A non-defatted protein percentage of 58.32% calculated by Ghosh et al. (2017) for Gryllus bimaculatus is comparable to our result. To date, values of protein content specifically for defatted B. mori pupae and G. bimaculatus have yet to be reported.
Ultrasound is widely used for extraction of substances such as herbal oils, protein, and bioactive components in plant material (flavones, polyphenolics) (Vilkhu et al., 2008). For insects, sonication methods have not been reported, although electric blending (Hall et al., 2017; Yi et al., 2013) and extraction with NaOH (Zhao et al., 2016) or HCL (Longvah et al., 2011) have been reported. Thus, in our experiment the extraction of protein through sonication was performed on three edible insects up to a duration of 20 min. Peak protein yield was 94% for silkworm pupae, 37% for cricket, and 35% for mealworm. Sonication for 1 min, to a maximum of 15 or 20 min, produced an increase in protein yield for the aforementioned insects of 76%, 34%, and 28% respectively. Due to the lack of research on ultrasound-assisted extraction of protein from insects, the results cannot be thoroughly discussed in detail. The differences in protein yield percentage between silkworm pupae and the other two insects may be attributed to size differences (surface area of crude samples) and the greater prominence of chitin in the exoskeletons of both mealworms and crickets. Our experimental procedure might have caused some sample loss, leading to underestimation of protein percentage, especially for samples with a higher chitin content. After sonication, the suspensions contained suspended particles of the insect being tested, and the sieved residues were discarded to reduce the insoluble fraction. The silkworm pupae sample was the most soluble of the three, which might explain the results obtained. A mechanical method to more finely homogenize the samples prior to sonication and testing of the insoluble fractions caught in the sieve should be investigated to improve accuracy. Using soy protein as an alternative reference point, ultrasound technology applied to defatted soy protein flakes showed not only a significant reduction in particle size, from 1000 µm to 90 µm, but also a 10.5% increase in overall protein release (Karki et al., 2010). After 2 min of sonication, the protein yield increased by 14% and 18% in mealworm and cricket samples, comparable to the results published by Karki et al. (2010).
The amino acid profiles of the selected edible insect samples are summarized in Fig. 3. No significant changes in amino acid profiles were observed after sonication. In comparison to previous reports (Ghosh et al., 2017; Longvah et al., 2011; Rumpold and Schlüter, 2013; Yi et al., 2016; Zielińska et al., 2015), the selected insects showed a high-quality amino acid profile that meets typical amino acid requirements for human nutrition. The amino acid profiles of insects are usually quite similar, but both proline and tyrosine values were much higher in mealworms. Since tyrosine is biologically related to phenylalanine, their contents are commonly reported together (Rumpold and Schlüter, 2013; Yi et al., 2016) but others have reported a much lower tyrosine content in T. molitor larvae (Zielińska et al., 2015). Initial levels of proline in T. molitor, prior to sonication treatment (7.21%), were similar to levels reported by Yi et al. (2013), at 6.6%, but higher than the 4.34% reported by Zielińska et al. (2015). An extraction method that did not use a defatting step displayed lower protein values than the method reported here (Ghosh et al., 2017) but the trends were comparable. Thus, our results generally corroborate previously published results.
Conclusion
Hexane defatting of whole insect samples yielded 1.36% or less residual fat content, and a protein content of 62-74%. Protein extraction using sonication increased protein yield percentages for all insects. Although the increase in protein yield was lower for mealworm and cricket samples than for silkworm pupae (94%), this may have resulted from characteristics of the insect specimens themselves or their life stage. Hexane defatting significantly decreased total fat percentage and ultrasound-assisted extraction increased protein yield percentage. These findings will further the exploration of edible insect protein as an ingredient in meat and dairy products.