Introduction
Goat has been one of the most valuable food resources since the beginning of human civilization (Webb et al., 2005). Korean Native Black Goat (KNBG; Capra hircus coreanae) is a single breed in Korea. About 80% of its fur is black. In South Korea, goat is one of the major livestock entity. Due to insufficient supply in the domestic meat market, the goat meat industry has not developed significantly compared to other livestock (Son, 1999). Native grass and agriculture byproducts have been used to raise KNBG. Korean consumers have traditionally considered KNBG as a folk medicine rather than a food because of their beneficial therapeutic effects. Although KNBG has extensive involvement in Korean culture, the exiting literature regarding KNBG meat is very limited compared to studies on other meat such as beef, pork, and chicken. We have recently reported research results on how to improve nutritional values (Hwang et al., 2017; Lee et al., 2018), fatty acid profile (Hwang et al., 2018), slaughter method (Bakhsh et al., 2018), post-mortem storage and slaughter age (Bakhsh et al., 2019), and different time and cooking temperatures (Ismail et al., 2019) for KNBG.
The recent surge in consumption of goat meat among Korean consumers, the full-time goat farming also increased in Korea (Jeong et al., 2006). Selection of specific muscle type and slaughter age in goat meat is now scorching subject of concern among consumers. It is well known that animal age has a strong impact on meat quality and predominantly marketing decisions. As old animals are heavier, their age affects the tenderness and color of the meat (Purchas, 2007). It is a general observation that meat of young or lightweight animals is favored by consumers because younger or light-weighted animals yield more tender meat than older or heavier animals (Vergara et al., 1999). In addition, it is also well known that muscle types play a major role in ultimate meat quality (Lawrie and Ledward, 2006). Muscle fiber type is responsible for the variation in meat quality within and between muscles (Kirchofer et al., 2002). Therefore, it is necessary to investigate the difference in meat quality between adult and young KNBGs according to muscle types. Thus, the purpose of this study was to investigate the effect of slaughter age and muscle type on meat quality and tenderness for four major muscles of KNBG.
Materials and Methods
Ten castrated male KNBGs were selected based on age of goat, including adult goat (AG) weighing 30.93±0.5 kg (n=5, 18-month-old) and young goat (YG) weighing 14.33±0.5 kg (n=5, 9-month-old) from the same farm. Transportation of experimental animals from the farm to the slaughterhouse was carried one day prior to slaughter. The experimental animals were rested for 18 h with access to water but with restriction of food. The slaughter of experimental animals took place in a commercial slaughterhouse.
Immediately after slaughter, about 20 g of sample was taken from the center of longissimus dorsi (LD), psoas major (PM), semimembranosus (SM), and gluteus medius (GM) muscles and then frozen in liquid nitrogen for histochemical analysis. Approximately after 24 h of chilling in a cold room, these four muscles were excised from the left and right sides of carcasses to investigate meat color (CIE L*a*b*), water-holding capacity (WHC) including cooking loss % (CL%) and release water % (RW%), Warner-Bratzler shear force (WBSF), sarcomere length (SL), myofibrillar fragmentation index (MFI), and collagen content.
Transverse serial sections for histochemical analyses were prepared from the entire block of frozen meat sample. All serial sections (10-μm in thickness) for dyeing were created using a cryostat microtome (HM525, Micromesh, Walldrof, Germany) at −27°C. Immediately after preparing these sections, incubation for histochemical demonstration was carried out according to the method of Brooke and Kaiser (1970) with slight modification as described by Hwang et al. (2010). The serial sections were incubated with myosin adenosine triphosphatase (mATPase) after alkaline (pH 10.70) and acid (pH 4.63) pre-incubation. An image analysis system (Image-Pro®plus 5.1, Media Cybernetics, Rockville, MD, USA) was used for analyses of these stained sections. About 600 fibers per sample were counted to estimate muscle fiber characteristics. According to the nomenclature of Brooke and Kaiser (1970), fibers were divided into fiber type I, IIA, and IIB. Total fiber number and area percentage were calculated. Fiber number percentage (FNP) refers to that each fiber type has a certain number of muscle fibers counted numerically, while fiber area percentage (FAP) is total cross-sectional area of each fiber type to the total fiber area.
Determination of meat color was conducted using a Konica Minolta Colorimeter (CR-300, Japan) equipped with a standard illuminant D65 by means of a 2°position of the standard observer with a pulse xenon lamp and 8 mm of reading surface area. L* (lightness), a* (redness), and b* (yellowness) color values were measured at three various locations. CIE a* and b* values were used to calculate saturation index [(a*2+b*2)1/2] and hue angle [(b*/a*) tan−1]. Corresponding muscle samples were exposed to bloom at 25°C for 30 min. The instrument was calibrated after each series of measurements. White ceramic plate were used to calibrate the instrument (Y=93.5, χ=0.3132, y=0.3198). An average of three readings was recorded from different locations of each sample group surface and used for statistical analysis.
WHC measurement of meat was carried out based on base on CL and RW methods. CL % was determined as described previously by Hwang et al. (2010). Release water (RW %) was based on the method of Joo (2018). A meat sample (3.0±0.05 g) was placed on a previously dried and weighed filter paper (Whatman No. 1 of 11 cm of diameter) with two thin plastic films. After weighing them with an electrical balance, the filter-paper and plastic film with meat sample between Plexiglas plates. A load of 2.5 kg and free mechanical force were applied for 5 min. The wet filter paper and plastic films were then quickly weighed after precisely removing the compressed meat. The percentage of RW was calculated as follows:
WBSF was conducted for cooked samples using established procedure of AMSA (1995). In a direction toward myofiber orientation, sample core (1.3 cm-diameter) was inserted into a cooked sample. These cores were sheared perpendicular to myofiber direction using an Instron tensile testing machine (Model 4443, Instron, Norwood, MA, USA) with a V-shaped shear blade. The final force was gained using 50 kg load cell tension produce at a crosshead speed of 250 mm/min. The recorded force during the test was termed as shear force. From each sample group, average of five readings was taken.
SL was evaluated with a method based on principles of Cross et al. (1981). Samples (3.0×3.0×2.0 cm) were run longitudinally and kept in vials comprising solution A (0.39 M boric acid, 0.1 M KCl, and 5 mM ethylene diaminetetraacetic acid in 2.5% glutaraldehyde) for 2 h. Samples were then shifted to new vials containing solution B (0.25 M KCl, 0.29 M boric acid, and 5 mM ethylene diaminetetraacetic acid in 2.5% glutaraldehyde) for 17-19 h. On a subsequent day, each fiber was split into small fragments and deposited into a microscope slide with a droplet of B solution. The slide was then positioned horizontally in the track of a perpendicularly directed laser ray to give a range of diffraction bands on a screen. Sarcomere length (μm) was calculated using the following formula:
Where Dwas the distance from the sample to diffraction pattern screen (D=98 mm) and Twas the separation (mm) between zero and the first maximum band.
MFI was measured according to the method of Hopkins et al. (2000) with minor changes as described by Nakyinsige et al. (2014). Briefly, 2.5 g of muscle was homogenized with 30 mL 20 mM ice-cold potassium phosphate buffer (pH 7.0) containing 100 mM KCl, 1 mM EDTA, and 1 mM MgCl2 for 60 s. Homogenates were then centrifuged at 1,000×g for 15 min at 2°C. Obtained supernatants were discarded while pellets were re-suspended in 25 mL phosphate buffer. Centrifugation at 1,000×g for 15 min at 2°C was then repeated. Similarly, after resulting supernatants were discarded, pellets were suspended in 15 mL of phosphate buffer and vortexed. To remove the remaining connective tissues, myofibril suspensions were filtered into 50 mL conical centrifuge tubes using 1.0 mm polyethylene strainers and then rinsed with an additional 15 mL of phosphate buffer. The myofibril suspension was diluted with potassium phosphate buffer to a final protein concentration of 0.5±0.05 mg/mL and the absorbance of the diluted myofibril suspension was measured at 540 nm with a UV-Vis spectroscopy system (Agilent 8453, Agilent, Santa Clara, CA, USA). Triplicate absorbance readings were averaged and multiplied by 150 to obtain MFI.
Collagen content was measured with the method of AOAC (2002). Briefly, meat sample of 4 g was taken and 30 mL of H2SO4 was added to the sample for hydrolysis at 105°C for 16 h. Water was poured into a funnel to reach up to total volume of 500 mL after hydrolysis. A filtered solution of 5 mL was diluted with distill water up to 100 mL. To measure hydroxyproline content, 2 mL diluted solution was taken after adding 1 mL of oxidation solution (50 mM of chloramine-T hydrate, 156 mM citric acid, 375 mM NaOH, 661 mM sodium acetate trihydrate, 29% v/v 1-ptopanol, pH 6.0). Vortexing of samples was carried out. Samples were then kept at room temperature for 20 min by adding 1 mL of color reagent. These vortexed samples were covered with aluminum foil and kept in a water bath at 60°C for 15 min. Before measuring the absorbance level, samples were cooled down by holding it to running water for 3 min. The absorbance was measured at wavelength of 558 nm using a UV spectrometer. From standard curve created using 1.2, 2.4, 3.6, and 4.8 μg hydroxyproline per mL of H2O, hydroxyproline content was calculated. Results were computed with the following formula:
This experiment had a completely randomized design with a 4 (muscle: LD, PM, SM, GM)×2 (age: AG vs LB) factorial arrangement of treatments. All data of FNP, FAP, CIE L*a*b*, released water, CL, WBSF, SL, MFI, and collagen content were analyzed using general linear model procedure of Statistical Analysis System ver. 9.3 (SAS, 2002) and a model containing muscle, age, and interactions of these terms. Significance difference was defined at p<0.05. Pearson Correlation coefficient was conducted to describe the relationship between meat quality traits and muscle fiber characteristics using SAS PROC (SAS, 2002).
Results and Discussion
As shown in Fig. 1, muscle fibers in four major muscles from adult and young KNBG were divided into three types: type I, IIA, and IIB. Overall, muscle fibers of AG looked larger than those of YG, whereas the muscle fiber number of YG seemed to be more than that of AG. Muscle fiber characteristics (FNP and FAP) of the four muscles by age of KNBG are summarized in Table 1. A distinct variation in fiber type composition was observed among muscles in both AG and YG. PM had significantly (p<0.001) higher FNP and FAP of type I than other muscles. However, there was no significant difference between AG and YG for all four major muscles (p=0.966). No significant interaction between muscle type and slaughter age was observed in FNP and FAP of type I fiber. However, significantly higher FNP and FAP of type IIA were found for LD and SM muscles of YG compared to those of AG (p<0.001), whereas LD had the highest FNP and FAP of type IIA, followed by GM, PM, and SM muscles regardless of age. A significant interaction between muscle and age was observed in FNP and FAP of type IIA. FNP and FAP of type IIB were significantly higher in LD, SM, and GM muscles of AG compared to those of YG (p<0.001). No significant interaction between muscle and age was detected in FNP and FAP of type IIB. The interaction between muscle and age only affected FNP of type IIA (p=0.014) and FAP of type IIA (p<0.001).
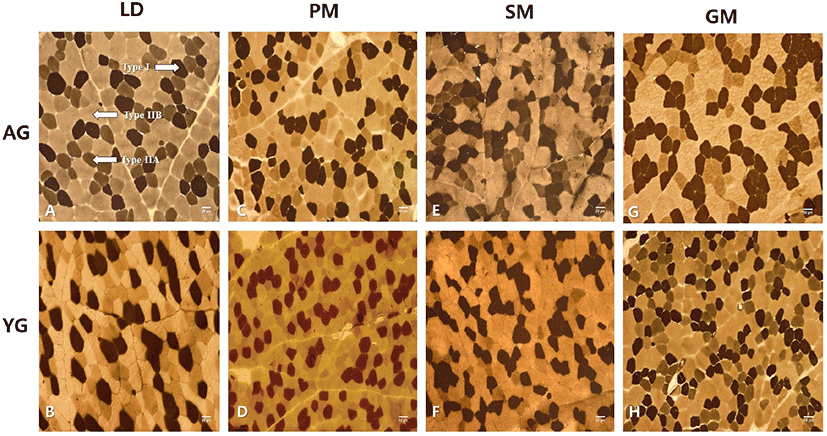
Numerous factors are known to play a key role in fiber type variation, including sex (Ozawa et al., 2000), age (Candek-Potokar et al., 1998), and breed (Ryu et al., 2008). Similarly, Swatland (1984) has described that muscle fibers undergo a frequent modification throughout life as an adaptation to shifting functional demand and that “fiber type” purely reveals the constitution of fiber at any particular time. Moreover, in an experiments using KNBG, Hwang et al. (2017) have reported that PM muscle has the highest FNP of type I but the lowest FNP of type IIB, while SM has the highest FNP of type IIB. These results appeared to be similar to results of the current study, with slight variations between the two studies possibly due to age difference. Furthermore, Bures and Barton (2012) have investigated bulls with four muscles (longissimus lumborum, semitendinosus, biceps femoris, and psoas major) and found significant differences in muscle fiber cross-sectional area, fiber type proportions, and the relative area of the muscle occupied by each fiber type between muscles. The smallest cross-sectional area of all fiber types was detected in the PM muscle, while the highest values were found in the ST muscle for type I and in the BF muscle for types IIA and IIB. The lowest fiber type proportions and relative areas of the muscle fiber types I, IIA, and IIB were observed in ST, LL, and BF muscles, respectively (Bures and Barton, 2012). These results are virtually consistent with the current study. Dissimilarities in muscle fiber number and muscle fiber area could be due to different species and different age of the experimental animal. Our earlier studies have also demonstrated that FNP and FAP of muscle fiber types vary due to variations in intramuscular fat content in several major Hanwoo muscles (Hwang et al., 2010; Joo et al., 2017). Similarly, other researchers have reported that muscle fiber characteristics and IMF content are influenced by various intrinsic and extrinsic factors, including species and breed (Joo et al., 2013; Sirin et al., 2017).
Effects of slaughter age and muscle type on color coordinates (CIE L*, a*, and b*) in goats are shown in Table 2. There were significant (p<0.001) differences in CIE L* and b* values between AG and YG and among four muscles. YG meat had higher L* and lower b* values than AG meat. The highest L* and b* values were observed in LD muscle (p<0.001). However, a* values were not significantly p>0.001) influenced by slaughter age and muscle type. The interaction between muscle and age only affected CIE b* (p=0.004).
A few studies have compared meat quality traits and muscle fiber characteristics in different muscles in KNBG. Previously, Hwang et al. (2017) have studies KNBG meat in four major muscles and found higher a* value in PM but lower a* value for SM, while LL has the highest L* value and SM has the lowest L* value. In the current study, higher L* values were recorded for LD and SM muscles among YG in relation to AG, showing higher FNP and FAP of type IIA. Similarly, L* values were positively correlated with FNP of type IIA and FAP of type IIA (r=0.47 and r=0.49, respectively; Table 3). These results suggest that the lightness of goat meat could be influenced by muscle type due to compositions of muscle fiber type in muscles. However, b* values were most affected by slaughter age. All four muscles of AG had significant higher b* values than YG (p>0.001). Regarding muscle type, LD had the highest b* values, followed by SM, PM, and GM muscles. Similar results were also reported for Chianina beef cattle slaughtered at two different ages (18-19 months and 20-21 months) in three different muscles (longissimus horacis, semitendinosus, and triceps brachii) with marginal differences in b* values based on slaughtering age. However, significant higher differences (p<0.001) were reported among muscles (Preziuso and Russo, 2004). Lee et al. (2018) have described that KNBG slaughtered at 36 months of age has similar results for b*, with LD and SM having the highest values, followed by PM and GM. In the present study, b* values were strongly positive correlated with FNP of type IIB and FAP of type IIB (r=0.50 and r=0.54, respectively; Table 3).
Table 2 shows WHC of meat expressed as RW and CL%. WHC was not influenced by slaughtering age, although SM had the highest RW and CL%, followed by GM, LD, and PM muscles. Consistent with the current study, De Palo et al. (2013) have investigated horse (6 months, 11 months, and 18 months) meat at different age and Ilavarasan et al. (2015) have determined goat (adult and young) meat slaughtered at different age, showing no significant difference in WHC. Similarly, RW% and CL% were strongly positively correlated with fiber number type IIB and fiber area type IIB (r=0.67 and r=0.55; r=0.61 and r=0.54, respectively; Table 3).
Changes in tenderness-related measurements including WBSF, SL, MFI, and collagen content are shown in Fig. 2. There were significant differences in WBSF, collagen between muscle types, and slaughter age (all p<0.001). PM had the lowest shear force values and presented more tender meat, whereas SM expressed the maximum toughness followed by GM and LD muscles of both AG and YG (Fig. 2A).
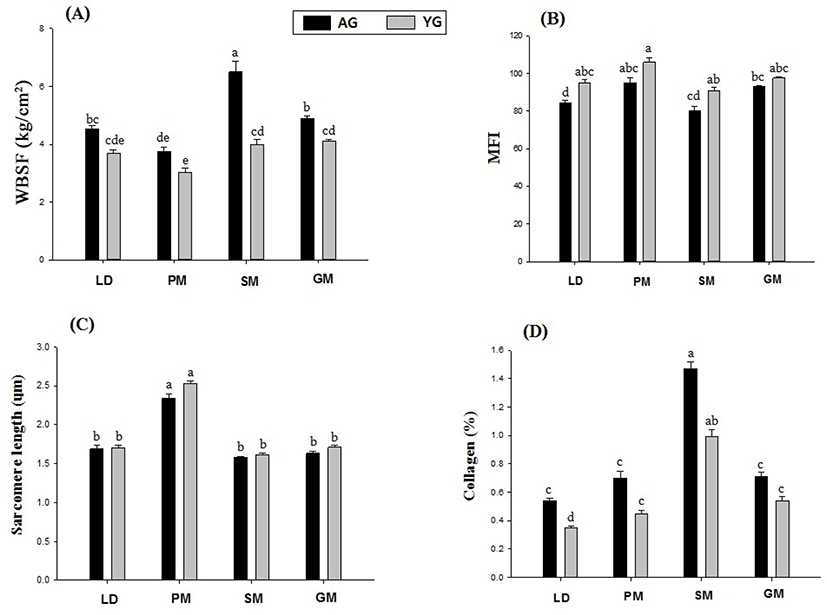
WBSF, which characterizes meat tenderness, depends on the structure of two main protein components of a muscle, i.e., proteins of intramuscular connective tissue and myofibrillar proteins. Their activity is determined by muscle type and animal’s age (Purslow, 2005). According to Bouton et al. (1978) and Forrest et al. (1975), after the growth and development of an animal after a certain age, a gradual toughening effect on the muscle will occur. They have also described that the decrease in tenderness seems to be largely due to increased number of crosslinks in collagen fibrils. Similarly, Bakhsh et al. (2019) and Wojtysiak (2013) have reported that older animals contain higher numbers of crosslinks of connective tissues than younger animals. This crosslink of connective tissue might have mainly contributed to the background of toughness of muscle food such as goat and pork meat. Dransfield and Jones (1981) have reported that LD muscle is more tender than SM or semitendinosus muscles. Results of the current study showed that PM muscle was tender than other muscles. Furthermore, WBSF values showed strongly positive correlations with FNP and FAP of type IIB (r=0.75 and r=0.77, respectively; Table 3). These relationships between WBSF and proportion type IIB fiber were in agreement with results observed for different breeds of cattle (Bures et al., 2015) and different muscles of KNBG (Hwang et al., 2017). Although these results are variable and sometimes contradictory, the current observations suggest that relationships exist between muscle fiber characteristics and meat quality of KNBG.
According to Kandeepan et al. (2009) and Olson and Stromer (1976), MFI and SL are good indicators of tenderization due to their close relationships with meat tenderness. MFI activity supports our WBSF values, showing that YG had higher MFI activity but lower WBSF values than AG, confirming that YG goat was tenderer than AG. Ilavarasan et al. (2015) and Kandeepan et al. (2009) have reported that aged goat and Cara-beef have higher MFI values than younger ones. They demonstrated that animal age has more influence on tenderness attribute than sex of the animal (Huff and Parrish, 1993). Results of the current study showed that PM of YG had significantly different SL value than that of AG (p<0.05). These results are consistent with those of Weaver et al. (2008). They reported that muscles with longer SLs had lower resistance of shear force (more tender). Moreover, SL had strongly positive correlations with FNP and FAP of type I (r=0.85 and r=0.85, respectively; Table 3).
Considering these results, it is important to discuss collagen content according to slaughter age and muscle type (Fig. 2D). The highest percentage of collagen content was observed in SM muscle among all muscles, regardless of muscle type LD muscle showing significantly different values between AG and YG.
Tenderness is the highest for meat from very young animals. It subsequently declines with increasing age and physical activity, along with an increase in the amount of collagen and its degree of complexity (Astruc, 2014). Similarly, Wojtysiak (2013) have observed pigs slaughtered at different ages (90 d, 150 d, and 210 d) and found significantly higher percentage of collagen and higher WBSF values in the old age group than those in the young age group, consistent with current results. Table 3 shows strong positive correlations of collagen content with FNP and FAP of type IIB (r=0.45 and r=0.36, respectively). This confirmed that lower WBSF and higher collagen content in different muscles of AG and YG could lead to more tender meat in different muscles.
Conclusion
Muscle fiber compositions of different muscle types of goat meat depend on the age. As a result, meat quality traits such as color, MFI, collagen content, and tenderness also vary depending on age. Moreover, AG had higher b* value, lower MFI %, and high WBSF values than YG. It also produced tougher meat than YG. Regardless of age, muscle type has a great effect on meat quality traits. Especially, SM muscle was the toughest muscle among all muscles, showing higher WBSF and collagen content but lower SL and MFI values among all muscles. While PM muscle was tenderer at young slaughter with lower WBSF with higher MFI and lengthier sarcomeres between AG and YG.