Introduction
Consumption of animal products in excess of need has been associated with cardiovascular diseases due to the high content of saturated fatty acids (SFAs) in animal products. In contrast with dietary monounsaturated fatty acids (MUFAs) such as oleic acid, SFAs are the main contributors to increasing low-density lipoprotein (LDL) levels in the blood (Jones et al., 2015). The substitution of SFA with oleic acid in a diet improves insulin sensitivity, decreasing the risk of diabetes and enhancing the protective effects on inflammatory responses and liver damage (Jurado-Ruiz et al., 2017; Palomer et al., 2018). Dietary polyunsaturated fatty acids (PUFA) also promote health benefits for humans. Drouin-Chartier et al. (2018) reported that the substitution of dietary SFAs with n-6 PUFAs decreased the production levels of LDL. Although food of animal origin is rich in n-6 PUFAs such as linoleic acid, daily intake of n-6 PUFAs should be balanced as closely as possible with the intake of n-3 PUFAs to prevent chronic inflammatory diseases (Hammad et al., 2016; Patterson et al., 2012). The recent, dramatic increase in the global attention on healthy food has become a subject of interest in both academia and the food industry. Therefore, the reformulation of meat products could be an alternative strategy for providing healthier meat products.
The replacement of animal fat in meat products with vegetable and/or fish oils has become an interesting subject from a human nutritional point of view. Vegetable oils provide dietary MUFA and PUFA for human needs. However, the replacement of solid fat with PUFA-rich oil is a technological challenge, as this oil does not provide plasticity to meat batter and thus impairs the emulsion stability and texture of these products (Baek et al., 2016). Canola oil is one of the oleic acid-rich vegetable oils that have both health benefits and potential applications in meat products. Canola oil prevents the development of diastolic dysfunction in a diet-induced rodent model of obesity and has good technological properties when included in an emulsion-type chicken sausage (Baek et al., 2016; Thandapilly et al., 2017). Another prospective substitute for solid animal fat is the edible oil extracted from the seeds of Perilla fructescens (Perilla), which is commonly used in Asia for culinary purposes. Perilla oil contains approximately 54–64% alpha linolenic acid (ALA), an n-3 fatty acid, and thus provides health benefits for humans (Asif et al., 2011). Supplementation with ALA from perilla oil decreases plasma total and LDL cholesterol concentrations in non-diabetic, borderline-to-moderate hypercholesterolaemic human subjects (Kim et al., 2016). To optimize the benefits of these oils while maintaining similar technological properties as using animal fat in meat products, an oil/water (o/w) emulsion could be formulated prior to application in the processing of meat products. An (o/w) emulsion made from vegetable oils and stabilizers (proteins and polysaccharides) is a promising technique for incorporating health-promoting ingredients together in the same foodstuff (Chang and McClements, 2016). This technique has also been applied previously in emulsion-type meat products (Asuming-Bediako et al., 2014; Pintado et al., 2018; Serdaroğlu et al., 2017). In this sense, a formulation of an (o/w) emulsion should be optimized considering many potential ingredients that can be used but may influence the technological properties of the product.
Response surface methodology (RSM) is a statistical approach widely used for optimizing various variables. In this regard, a complex factorial design such as a Plackett-Burman design (PBD) is used for screening the candidate variables and then a central composite design (CCD) is used for the optimization of the selected variables. The use of these designs can effectively minimize the error in measuring the effect of variables and their interactions (Yolmeh and Jafari, 2017). Therefore, the objective of this study was to optimize the formulation of a perilla-canola oil (o/w) emulsion using statistical approaches and to observe its potential application in a meat emulsion as an animal fat replacer.
Materials and Methods
Canola oil (CJ CheilJedang Co., Ltd., Korea), cold-pressed perilla oil (Ottogi Co., Ltd., Korea), food-grade beef tallow (Ottogi Co. Ltd., Korea) and frozen chicken breast (5-week-old Ross broiler) were obtained from a local market. Soy protein isolate (SPI, 100% defatted soy protein concentrate), fish gelatin (FG, 100% pure), sodium caseinate (SC, 90% casein), carrageenan (CR, BF-100), sodium tripolyphosphate (STPP), refined salt, sodium nitrite and sodium erythorbate were purchased from Esfood Co., Ltd. (Korea). Polyglycerol polyricinoleate (PGPR, MASEMUL™ PGPR 9090) manufactured by the esterification of condensed palm oil fatty acids with polyglycerol, was purchased from Musim Mas Ltd. (Indonesia). Inulin (2944B-V7) extracted from blue agave (Agave tequilana) was purchased from NOW Foods Ltd. (USA). Frozen chicken breast was thawed in a chilling room (2±2°C) overnight prior to the grinding process. The meat was cut into cubes after any subcutaneous fat and visible connective tissue was removed. The cubed meat was then ground through 6-mm plate grinder (M-12S, Hankook Fujee Industries, Korea). The ground meat was used for the meat emulsion.
The PBD was used to identify the most significant variables for manufacturing an (o/w) emulsion with a suitable appearance (color and creaming index). This screening method is efficient for identification of the important factors among a large number of factors affecting selected responses (Gündoğdu et al., 2016). The following first-order polynomial model was used for mathematical modeling:
where Y is the predicted response, β0 is the model intercept, βi is the linear coefficient and xi is the level of the independent variable.Seven independent variables and their addition levels (% w/w) were selected as follows: PGPR (0–3.2%), FG (0–1%), SPI (2–5%), SC (0–1%), CR (0–1%), inulin (0–3%) and STPP (0–1%). The emulsion pH, instrumental color, rheological properties and creaming index were all determined as the responses. In this study, a 12 run PBD was carried out for evaluation at two addition levels: –1 for the lower level and +1 for the higher level, with three replicates at the central point (0), totaling 15 runs. The responses were determined 12 h after (o/w) emulsion preparation. The independent variables with confidence intervals of more than 95% (p<0.05) were considered to have a significant effect on the response and were further optimized using response surface methodology.
After screening using the PBD, three significant variables (k=3) were selected and the optimization of the (o/w) emulsion was carried out using a CCD with 2k factorial points, 2k axial points and six replicates at the central point. SPI, CR and IN were selected considering their influences on the technological properties of the (o/w) emulsion and the nutrition value of the final product. They were denoted as X1, X2 and X3, respectively. Each variable was assessed at five different addition levels (–1.68, –1, 0, +1, +1.68). The design consisted of eight factorial points, six axial points and six replicates of the central point, totaling 20 runs.
The responses were analyzed using a second order polynomial equation, and the data were fitted by a multiple regression procedure. The statistical relationship between the response (Y) and independent variables is given by the following quadratic polynomial equation:
where Y is the response; X1, X2 and X3 are independent variables; β1, β2 and β3 are linear regression coefficients; β11, β22 and β33 are quadratic regression coefficients; β12, β13 and β23 are interactive regression coefficients; and β0 is a constant term.The (o/w) emulsion was prepared with 50% oil. For the oil phase, canola oil was mixed with perilla oil at a 70:30 (w/w) ratio. In the initial screening study using PBD, the oil phase was prepared in the absence or presence of PGPR. As PGPR was crucially important for inhibiting the emulsion break, the addition level was then fixed at 3.20% (w/w) of the total (o/w) emulsion for further optimization studies. For the aqueous phase, the other ingredients were mixed with 50°C distilled water and the addition level was based on the experimental design. Each oil and aqueous phase was homogenized separately using a hand mixer (EasyMax HT2511KR, Tefal, France) at maximum speed for 2 min, and then phases were mixed together using a food blender (HMF-1600PB, Hanil Electric, Korea) at maximum speed for 2 min. Both the oil and aqueous phases were heated to 50°C in a water bath prior to mixing and homogenization, according to Mun et al. (2010). After homogenization, the mixture was cooled down to room temperature and then stored in a chilling room (2±2°C) for 12 h until further analysis.
The pH value of the sample was recorded in triplicate using a pH meter calibrated with acid (pH 4.01), neutral (pH 7.00) and alkali (pH 9.21) technical buffer solutions (Seven Easy pH, Mettler-Toledo GmbH, Switzerland) at 22°C according to manufacturer’s instruction. For color measurement, emulsion was poured onto a petri dish. The instrumental surface color was recorded in five repetitions by measuring International Commission on Illumination’s system for lightness (CIE L*), redness (CIE a*) and yellowness (CIE b*) using a chromameter (CR-400, Konica Minolta Inc., Japan). The light source of illuminant C (2° observer) with 8 mm aperture and attached-closed cone was calibrated using a white plate (Y=93.6, X=0.3134, y=0.3194).
The storage (G’) and loss (G”) modulus of the (o/w) emulsion at room temperature (22±1°C) were determined in triplicate according to Delgado-Pando et al. (2010) with modifications. A rheometer (ARES, TA Instruments-Waters LLC, USA) was used with a stainless steel cone plate (50 mm diameter and 0.051 mm gap). Strain sweep tests were performed first to determine the linear viscoelastic region of each sample at 10 Hz. Frequency sweep tests were conducted later at 1 Pa between 0.1 and 10 Hz. The storage (G’) and loss (G”) modulus at 1 Hz were selected for data analysis.
Percentage of creaming index (CI%) was determined gravimetrically in triplicate according to Firebaugh and Daubert (2005) with modifications. Sample (10 mL) was filled into 15 mL-centrifuge tube and centrifuged at 1,000 g for 10 min at 4°C. The height of the oil released (Ho) and the total of height emulsion (He) were recorded. The CI% was reported as follows:
To observe the potential use of the (o/w) emulsion as animal fat replacer in optimization study, beef tallow was used as comparison in meat emulsion. The model system of meat emulsion was made with 20% (w/w) fat (o/w emulsion or beef tallow), 60% (w/w) ground chicken breast and 20% (w/w) ice. Refined salt (1.5%), sodium erythorbate (500 ppm), STPP (0.30%) and sodium nitrite (150 ppm) were added according to the weight of meat batter. Ground meat, ice and all the salts were homogenized using silent cutter for 2 min (CM-14, Mainca, Spain). The fat was then added and the batter was homogenized for another 2 min. Sample was vacuum-packed and stored in a chilling room (2±2°C) for 12 h until analysis.
The emulsion stability of meat emulsion was measured using centrifugation method according to Choi et al. (2007) with modifications. A sieve (19 mesh) was put into the middle of a specially formed glass tube and 10 g of batter was weighed into the glass tube. The glass tube was covered with aluminum foil, heated in a water bath at 75°C for 30 min and cooled down in a 2±2°C chilling room for another 30 min. Total fluid loss and oil release were determined gravimetrically and calculated as follows:
To determine cooking loss, meat emulsion was put on a petri dish (90×15 mm) to form a round shaped batter with a same thickness (1 cm), wrapped with aluminum foil and cooked in polyethylene zipper bags by water bath immersion at 80°C for 30 min. The cooked samples were then immediately removed from the bag and plate, cooled down in a chilling room (2±2°C) for 30 min and weighed. Cooking loss was expressed as the percentage of weight lost during heating.
The cooked meat emulsion was cut into 8 cubes (1 cm3) and then subjected to texture profile analysis using a TA-XT2i Plus instrument with a 50 kg load cell (Stable Micro Systems Ltd., UK) at room temperature. A cylindrical 35-mm-diameter probe was used in this study at a compression rate of 60%. The sample was placed under the probe that moved downwards at a constant speed of 5.0 mm/s (pre-test), 1.0 mm/s (test), and 5.0 mm/s (post-test) and the following parameters were obtained: Hardness (kg), cohesiveness, springiness (cm), gumminess (kg · cm) and chewiness (kg · cm).
The optimized formula of the (o/w) emulsion was validated by application in a meat emulsion in triplicate. The technological properties (emulsion stability and cooking loss) of the meat emulsion and the texture profile (hardness) of the cooked meat emulsion was used for validation.
Minitab® 18.1 (USA) was used for designing the experiments and for data analysis. Analysis of variance (ANOVA) and multiple regression analyses were performed to verify the fit of the second order polynomial model. The response surface plots of predicted responses of the model were used to evaluate the interactions between selected variables.
Results and Discussion
Seven ingredients (PGPR, FG, SPI, SC, CR, IN and STTP) were used to formulate the (o/w) emulsion. According to the PBD, the responses on the pH of the (o/w) emulsion, color, storage and loss modulus and the creaming index are shown in Table 1, while the effect of each ingredient is shown in Table 2. Among the ingredients, SC did not show significant effects on any response. Therefore, this ingredient was removed from the optimization study.
The pH values of the (o/w) emulsion ranged from 7.31 to 8.92 and were positively influenced (p=0.02) by the addition level of STPP. STPP is an alkaline salt and commonly used to enhance the water holding capacity of meat and meat products by raising the pH (Rigdon et al., 2017; Xue et al., 2016). Although STPP is crucial for increasing the water holding capacity of meat products, the pH of an (o/w) emulsion made without STPP did not tend to be in acidic range (still more than 7.00), which is beneficial for maintaining a high pH in meat batter when an (o/w) emulsion is applied in the processing of meat products. STPP also did not show any significant effect on color, rheological parameters or the creaming index. Moreover, STPP can be added later during the processing of meat products with regulated concentration. Therefore, STPP could be removed from the formulation of (o/w) emulsion.
For appearance, a light and less yellow fat color is more suitable for mimicking animal fat that is commonly used in meat processing. In this study, the CIE L*, a* and b* values of beef tallow were 61.91, –1.58 and –7.20, respectively. On the other hand, the instrumental color values of the (o/w) emulsion ranged from 48.3 to 84.1 for lightness (L*), –2.82 to –1.00 for redness (a*) and 17.4 to 24.5 for yellowness (b*). Most of the ingredients except for PGPR showed negative effects on the L* value. Although perilla oil has a typical intense yellow color, the use of canola oil, which has a lighter color, and PGPR as emulsifier could stabilize the lipid droplet and mask the intense yellow appearance (Mun et al., 2010). Considering the significant (p<0.01) negative effect of FG on the L* value while showing no effect on other responses, this ingredient was then removed from the formulation. IN decreased both the L* and b* values while CR showed a contrasting effect on the b* value. Thus, the utilization of CR and IN was optimized, considering their potential role in a meat emulsion as a gelling agent (CR) and a health-promoting agent (IN).
In terms of rheological properties, none of the ingredients significantly affected either the storage (G’) or the loss (G”) modulus of the (o/w) emulsion. Despite this, soy protein isolate (SPI) tended to increase both the storage (p=0.09) and loss (p=0.08) modulus. In all samples, the observed loss modulus was higher than the storage modulus, demonstrating that the sample had a weak gel structure (Murillo-Martínez et al., 2011). Supplementary 1A shows that the (o/w) emulsions were more viscous than a gel-like form. The storage and loss modulus values ranged from 0.01 Pa to 389.5 Pa for G’ and 0.01 Pa to 867.5 Pa for G”. SPI is widely used as emulsifier in food industries due to its functionality to form a Pickering emulsion system and its cost efficiency (Tang, 2017). Therefore, the addition level of SPI also needed to be optimized.
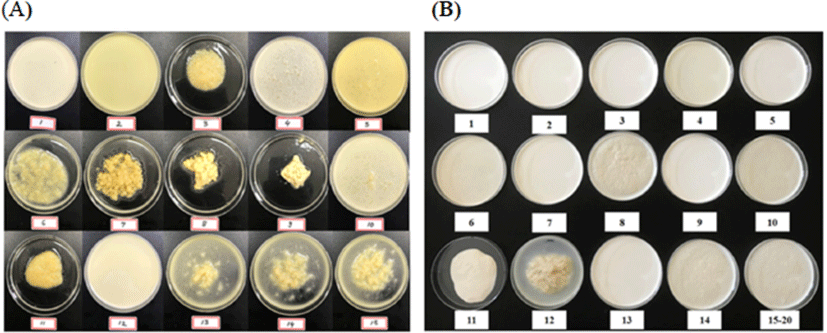
In the case of the creaming index, which demonstrates the stability of the (o/w) emulsion, PGPR played a very significant (p<0.01) role in inhibiting emulsion break, while other ingredients did not show significant effects on this. As creaming stability is the most important parameter determining a successful of (o/w) emulsion, PGPR was added at a fixed level (3.20% w/w of total emulsion) in the optimization study. The addition level of PGPR was also adjusted in a previous study (Serdaroğlu et al., 2017).
Three selected ingredients (SPI, CR, IN) were used as independent variables in a CCD to optimize the formulation of an (o/w) emulsion. The responses for pH, color, and the storage and loss modulus of the (o/w) emulsion are shown in Table 3, while the responses for the meat emulsion are shown in Table 4.
As PGPR was used at a fixed addition level, no emulsion break was recorded in the optimization study. The pH of the (o/w) emulsion ranged from 6.39 to 7.52, which was lower than that of the screening results, as some pH-raising ingredients such as STPP and SC were removed from the formulation. For the visual aspect of the (o/w) emulsion (Supplementary 1B), the appearance was lighter, less yellowish and more uniform than the screening results, as PGPR was added at a fixed level. The instrumental color values of the (o/w) emulsion ranged from 77.9 to 93.1 for lightness (L*), –3.12 to –1.44 for redness (*a) and 9.78 to 18.9 for yellowness (b*). The L* values increased, while the a* and b* values decreased in comparison with the results of the PBD. The storage and loss modulus of the (o/w) emulsion, which were lower than those of the screening results, ranged from 0.04 to 20.3 Pa for G’ and 0.41 to 113.9 Pa for G”. The decline in the storage and loss modulus demonstrates that the particle size of the (o/w) emulsion in the CCD was more uniform than that of the (o/w) emulsion in the PBD. PGPR is widely used in the chocolate industry as an emulsifier due to its ability to improve flow characteristics of molten chocolate by creating a uniform droplet size and thus weakening the gel aggregation (Afoakwa et al., 2007).
According to Table 4, a meat emulsion formulated with a perilla-canola oil (o/w) emulsion showed better emulsion stability, cooking yield and texture than those of the control meat emulsion. The cohesiveness, springiness, gumminess and chewiness were significantly (p<0.05) higher in a meat emulsion formulated with a perilla-canola oil (o/w) emulsion than in the control, and no differences were found on hardness. These data suggest that the replacement of animal fat with a perilla-canola oil (o/w) emulsion in emulsion-type meat products could be possible without impairing the technological and textural properties of the final product.
As the storage modulus (G’) is an important parameter determining the viscoelasticity of an emulsion, while cooking loss and hardness are two crucial technological and textural properties for a meat product, these three parameters were selected for study in a multiple regression analysis. The response surface plot for storage modulus, cooking loss and hardness is presented in Figs. 1A, B, and Fig. C, respectively. The following second order polynomial equations for storage modulus (G’), cooking loss and hardness, as affected by the addition levels of SPI (x1), CR (x2) and IN (x3), are the result of multiple regression analysis:
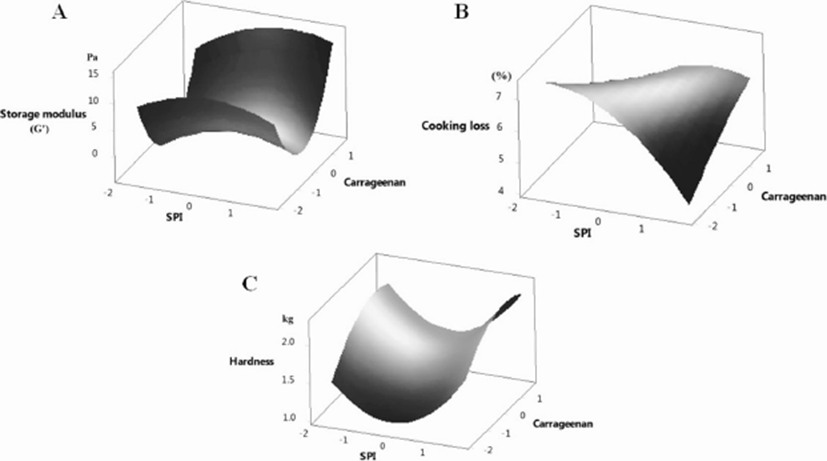
As CR showed a positive quadratic effect (p<0.01) on the storage modulus (Table 5 and Supplementary 2), both minimum and maximum addition levels of CR could improve the viscoelasticity of the emulsion. However, the interaction effect between SPI and CR resulted in an elevated cooking loss (p<0.01). SPI itself showed an ability to lower the cooking loss (linear effect: p<0.03) and the addition of IN along with SPI also negatively affected cooking loss (CR.IN interaction effect: p<0.01). The absence of CR in run 11 resulted in a more stable (mayonnaise-like) emulsion with the second highest storage and loss modulus of runs in the CCD. It has been suggested that the presence of CR reduces the solubility of protein in an emulsion system, thus forming aggregate gels. Molina Ortiz et al. (2004) reported that the formation of insoluble aggregates in a soy protein-carrageenan system is due to CR reducing protein solubility through electrostatic and hydrophobic interactions. On the other hand, IN has been suggested to enhance the gel formation of SPI, build a dense cross-linking structure with soy protein and thus reduce the pore size of an SPI gel (Tseng et al., 2008). As IN reduces the pore size of a protein gel, the oil droplets could be maintained inside an emulsion system.
The equations of the regression models were validated using selected optimal conditions (w/w of emulsion): SPI (4.48%), CR (0%) and IN (14%). The selected conditions were obtained by maximizing the storage modulus, minimizing the cooking loss and adjusting the hardness to that of a control meat emulsion using response surface predictions. Under these optimized conditions, the observed experimental values (in triplicate) of the storage modulus (17.45±0.68 Pa), cooking loss (5.63±0.23%) and hardness (1.81±0.12 kg) were close to the predicted model response (19.71 Pa for storage modulus; 5.21% for cooking loss; 1.76 kg for hardness) with low relative deviation. Although the results in technological properties show the potential use of perilla-canola oil (o/w) emulsion to replace solid animal fat, the overall quality of the emulsion-type meat product formulated with perilla-canola oil (o/w) emulsion should be further investigated. Freire et al. (2016) reported that frankfurter elaborated with an (o/w) emulsion made from perilla oil increased lipid oxidation and reduced overall acceptability in comparison with that made from pork back fat. As the (o/w) emulsion used in this study was made from a mixture of perilla and canola oil (30:70 w/w), the effect would be different from previous study.
Conclusion
Perilla-canola oil (30:70 w/w) stabilized with PGPR, SPI and IN are effective ingredients that can be utilized in the formation of an (o/w) emulsion as an animal fat (beef tallow) replacer in emulsion-type meat products. The use of 50% oil in the (o/w) emulsion could reduce the fat content of the final products. Moreover, the use of IN in the formulation of an (o/w) emulsion could fulfill the need for dietary fiber. Further studies are required to evaluate the consumer acceptance and shelf life of meat products formulated with a perilla-canola oil (o/w) emulsion.