Introduction
With the ongoing concerns related to environmental issues and the increasing population, the urge to innovate more sustainable protein food products, especially meat alternatives of meat with similar sensory profiling have gained attention from researchers and food industrialists (He et al., 2021; Mehrabi et al., 2020). Until now, meat alternatives have been attempted to be in line with animal meat products in terms of flavor, nutrition, and color (Alam et al., 2024; Joo et al., 2022; Kang et al., 2024). However, texture is considered the main sensory aspect that meat consumers have given more importance for searching a food to replace the meat from their diet (Kumari et al., 2024). As the structural foundation of meat depends on the basic structure of muscle fiber, developing a fiber-like structure is crucial to achieve a similar textural profile. Two techniques are mainly used to produce meat alternatives: top-down and bottom-up. Top-down techniques include the production through extrusion, shear cell, and freezing, while the bottom-up include wet-spinning, electro-spinning, and 3D printing (Dekkers et al., 2018; Kyriakopoulou et al., 2019). Among all the techniques extrusion (low moisture) and wet spinning are the only industrial technology. However, there have been some hindrances in the extrusion process due to high energy consumption, loss of nutrients because of high processing temperature, and low rehydration rate of the product without any specific structure similarity to the meat (McClements and Grossmann, 2021; Zahari et al., 2021). The other remaining technology faces problems like complications in operations and instability of the product, resulting in no industrial applications at the moment (Dekkers et al., 2018). At the same time, wet spinning is an industrialized production technique used to produce fiber-like structures for the textile industry. While considering the ingredients, soy protein isolate is the main ingredient used for the development of meat alternatives because of its high nutritional quality, and functional properties which have created a saturation in the use of raw material for alternative products. Inspired by Cui et al., (2022) for utilizing soy protein isolate, we hypothesized that other types of protein sources can also be utilised to produce fibers using the wet spinning technique.
Therefore, in this study, we are focusing on using other protein sources to counter the overdependence on soy protein for a sustainable food supply (Tang, 2019) and to expand the range of raw materials that are suitable for production. Here we utilized ingredients that are consumer-friendly to increase acceptance of these food items like pea protein isolate (PPI), wheat protein (WP), and myofibrillar paste (CMP: conventional meat paste) are protein sources, and sodium alginate (SA) is a thickener and emulsifier (Etter et al., 2024). As the diameter and the strength of the fibers are based on the type of raw material and processing parameters, optimization is crucial for the desired alternative product (Pawar and Edgar, 2012). The objective of this study is to utilize the different protein sources mentioned above using the wet spinning technique and perform a comparative analysis of the quality parameters in comparison to conventional meat (CM) to study the variation between them. Moreover, the results from this study can be beneficial for the production of meat alternatives without over-depending on one source of protein.
Materials and Methods
PPI and WP were purchased from an online platform and meat from a commercial slaughterhouse. SA with high viscosity was obtained from the Qingdao Gather Great Ocean Algae Industry (Qingdao, China). Calcium chloride was purchased from Qingdao Soda Ash Industrial Development (Qingdao, China). All the materials used for experiments were food grade. For CM and conventional meat fiber (CMF), the longissimus dorsi muscle was utilized from a barrow (Landrace×Yorkshire× Duroc, LYD). Muscle samples from barrow (6 months old, carcass weight 89 kg) were obtained from local farm at the Ansung, Korea.
Three samples with 8% WP, PPI, and myofibrillar paste in distilled water were prepared respectively and the fourth one with the combination of WP and PP in an equal concentration of (4%). On the other hand, a solution of 2% SA was prepared by dissolving it into the distilled water. All the solutions were kept overnight at 4°C to achieve complete hydration. Then, the solutions were mixed with SA in equal weight and degassed for 20 min using an ultrasonicator.
The wet spinning method was used to prepare imitated muscle fiber (IMF) from the spinning solution. The process flow of the IMF fabrication is shown in Fig. 1. The composite solution was extruded through a needle of 0.13 mm diameter into the 3% calcium chloride (w/w) coagulation bath at room temperature (20°C–25°C). The obtained IMF was suspended into the coagulation bath to attain the complete gelation of the solution. The macroscopic structural representation of fiber fabrication during the wet spinning process has been captured and illustrated from various angles (Fig. 2). After that, the IMF block was washed in the washing bath containing distilled water to remove the excess calcium chloride from the surface of the IMF block and finally, the IMF blocks were collected.
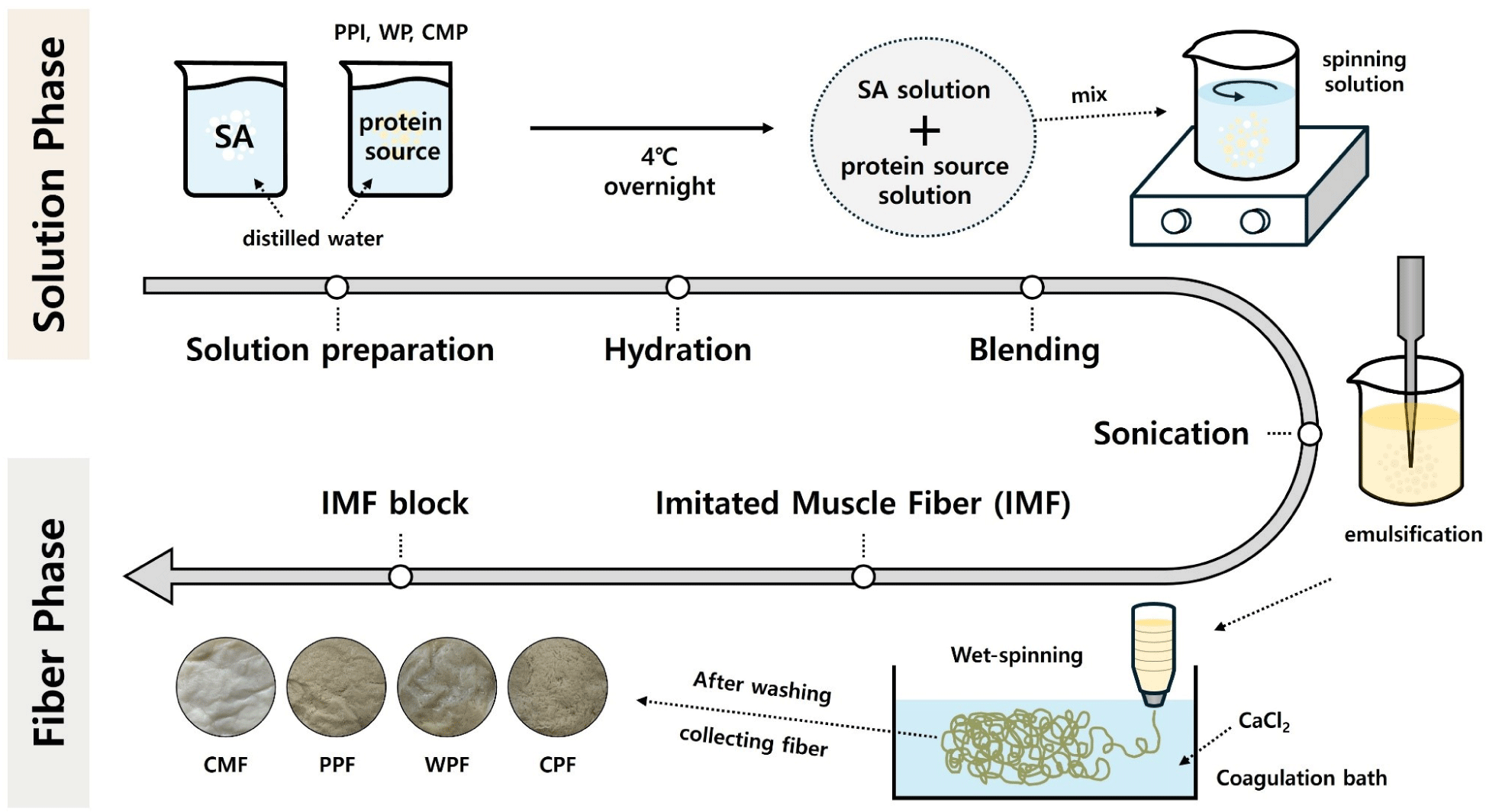
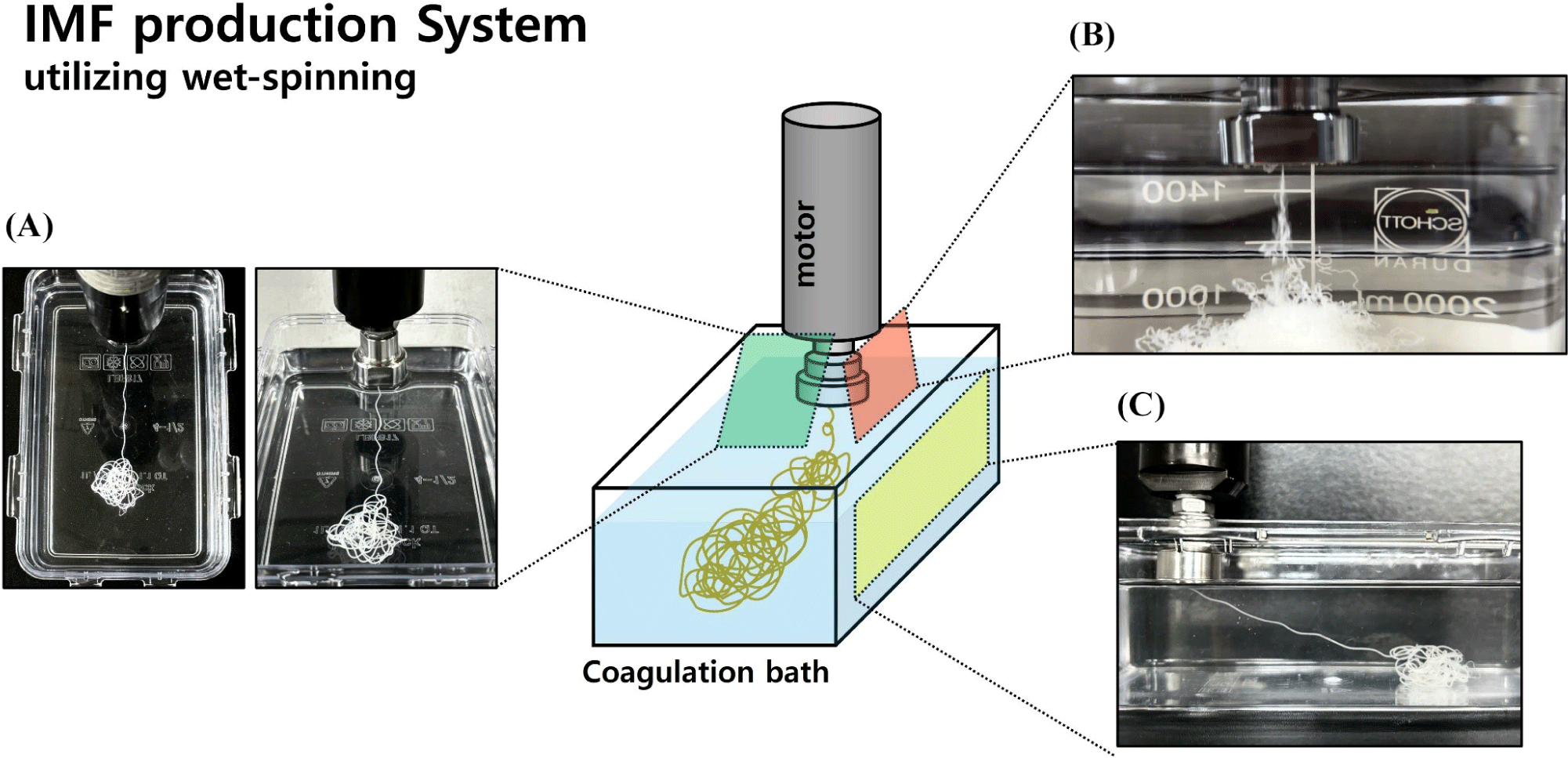
The moisture of the samples was analyzed using the Association of Official Agricultural Chemists (AOAC 650.46B) method. Samples (2 g) were weighed into the aluminium dish and allowed to dry for 16 h at 105°C in a dry oven. The moisture content was calculated as the percentage ratio of wet and dry weight. The experiment was conducted three times.
The water-holding capacity of samples was measured in terms of cooking loss. The samples were weighed and cooked in a water bath at 75°C for 30 min, followed by measuring the weight of the samples after 10 min of chilling (Pathare and Roskilly, 2016). The cooking loss of the sample was expressed as a percentage using the following formula (Wi et al., 2020). Measurement of CL was performed three times.
CL: cooking loss.
W1: weight of the uncooked sample (g).
W2: weight of the cooked sample (g).
The color or chromaticity indicates quality and freshness, measured by a Chroma Meter (CR-300, Konica Minolta, Osaka, Japan). The color parameters CIE L*, CIE a*, and CIE b* were determined in quintuplicate for each sample. Results were expressed as mean±SD.
3 g of the sample was homogenized with 27 mL of distilled water and pH was measured using a digital pH meter (A211 pH Meter, Thermo Fisher Scientific, Waltham, MA, USA).
The Warner-Bratzler shear force (WBSF) of the samples was measured through the texture analyzer (AMETEK, Berwyn, PA, USA) with a V-shaped shear blade on its shear mode to determine the tenderness. The curve obtained reflects the tenderness of the sample. The analysis was performed at a speed of 100 mm/min with a force of 50 kg. The data were expressed as the mean and SD of the values measured five times.
The textural characteristics were determined using a double compression test, which involves compressing the sample under constant conditions and measuring the force-time profile. The measurements were carried out using a texture analyzer (AMETEK). Using a sharp knife the samples were cut into 1 cm×1 cm×1 cm cubes and placed in the instrument’s measurement cell. Compression and decompression were conducted twice at a fixed speed of 100 mm/min and a maximum load of 180 kg. The force versus time graph automatically recorded the hardness, springiness, gumminess, chewiness and cohesiveness of each sample by the instrument software. The data were expressed as the mean and SEM for the values measured five times.
The efficiency of a process is a measurement of how effectively the process converts raw materials into finished products over a period of time. It is typically expressed as the unit of output per unit of input per minute.
The statistical analysis was conducted using SAS 9.4 (SAS Institute, Cary, NC, USA) and GraphPad Prism (10.1.2; GraphPad, San Diego, CA, USA). All data were represented as mean and SD, and texture profile analysis (TPA) was shown as mean and SEM. The resulted data were analysed by one-way analysis of variance (ANOVA; Brown-Forsythe and Welch) with the Dunnett T3 test. Analysis of TPA data was performed by one-way ANOVA with Tuckey`s multiple comparison test. Statistical significance was determined as p-value (p<0.05).
Results and Discussion
The morphological appearance of the IMFs was analyzed by virtually inspecting the fiber block. It has been observed that all the fibers were thin in appearance with significant color differences and were unaligned (Fig. 3). The difference in color profile is described by the color analysis (Table 1).
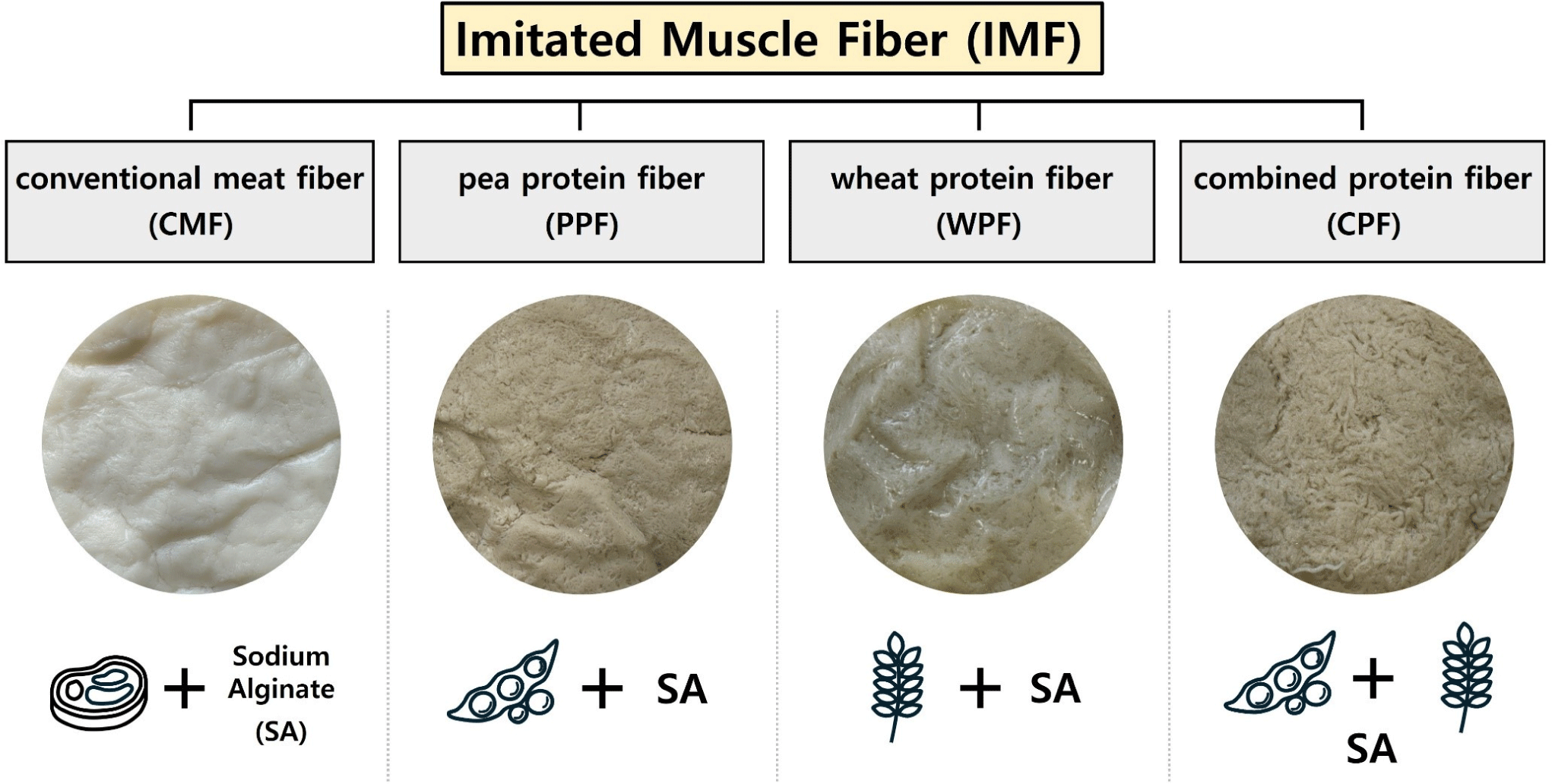
The color profile analysis includes the CIE L*, CIE a* and CIE b* of the product (Table 1). The CIE L* value indicates significant variations in CIE L* across the samples and was significantly highest in CMF (p<0.05). It suggests that the paste modification of CMF increased the CIE L* due to the incorporation of more air. Also, potential reduction in particle size could also have enhanced light scattering, resulting in higher reflectance compared to the control (CM, 53.81). Additionally, pea protein fiber (PPF) and combination protein fiber (CPF) were also lighter than CM, with values of 71.78 and 72.82, respectively due to the absence of dark pigment, contributing to its high CIE L*. CIE L* values of wheat protein fiber (WPF) had a higher CIE L* than CM, but lower than the other IMFs as it had a creamy color originally. CIE a* value for CM has the significantly highest, indicating a reddish tint while the other samples have low or negative values (p<0.05). Theses color difference was attributed to green due to their original characteristics (Sakata and Honokel, 2001). CIE b* value of PPF (14.60), CPF (14.26) and WPF (7.61) exhibited the highest CIE b*. PPI, contains yellow pigments naturally such as carotenoids, contributed to its high yellowish value substantially. This resulted higher yellowish than CM (2.99) indicating a shift towards yellow compared to CM (Asen et al., 2023). While CMP (1.41) shows a lower yellow value, suggesting a reduction in yellow hue due to the paste modification.
The pH and moisture content of IMFs demonstrated a bit higher than the CM (Table 2). The CMF had the highest pH (6.44) and moisture content (82.66) while the CM was the lowest. However, pH and moisture content in the other fibers ranges from 5.88 to 6.22 and 80.16 to 81.52 respectively. The change in the pH of C comparison to CM can be due to the neutralization of organic acids, interaction with protein, and change in the ionic strength, as demonstrated by Huff-Lonergan and Lonergan (2005). The difference in the pH between the plant protein fibers could be due to the difference in initial pH of PPI, WP. The use of calcium chloride during the processing of the fiber block creates the porous structure responsible for the high moisture accumulation in the fiber (Cui et al., 2022; Cui et al., 2023). In terms of CL of the IMF, the CM showed the significantly highest CL with 23.51% while the CPF has the lowest at 14.37%, giving a better fiber in terms of maintaining the juiciness of the fiber after cooking (p<0.05). A different rate of protein denaturation could be explained for the differences observed in the cooking loss across the fibers. While comparing the CL of the PPF, WPF, and CPF, it was found that the combination of PPI and WP helps to reduce the cooking loss significantly from the IMF from the individual sources. This combination can be suitable for manufacturing fibers with better mouthfeel and juiciness.
The textural properties including hardness, springiness, gumminess, chewiness, and cohesiveness of the IMFs were presented in Table 3. CM textural properties were included as a comparison to the IMFs. In springiness among the fresh IMFs, there is no significant difference (p>0.05). However, all the other parameters had significant differences in comparison to CM (p<0.05). The CMF showed significantly higher hardness, chewiness, cohesiveness, and gumminess than CM, which could be due to the fibrous nature of protein and cross-linking with SA during the process (Nagamine et al., 2023; Pietrasik and Jarmoluk, 2003). The chewiness, cohesiveness, and gumminess were also significantly higher in PPF due to the nature of legumin and vicilin that form a much denser and more compact structure and also the high fiber content and high-water holding capacity (Asgar et al., 2010; Day, 2013). However, significantly lower cohesiveness in WPF and CPF to CM could be caused by presence of WP (glutenin and gliadin), low fiber content, and low water retention capacity form a less cohesive structure with an elastic gel-like structure (Sha and Xiong, 2020; Shimoni and Galili, 1996).
The same trends have been observed in the springiness of fibers after cooking (p>0.05). Cooked CMF had significantly lower cohesiveness than CM (p<0.05). While the PPF has been found to have a significantly higher value of hardness, cohesiveness, chewiness, and gumminess than CM (p<0.05). The presence of gluten in the WPF had lowered these values. The contradictory effect of PPI and WP had created the difference in the WPF and CPF which were lower in every aspect except gumminess than to CM. The hardness of the fresh PPF was similar to fresh CM but it was reduced after cooking. CMF had the significantly highest hardness while the WPF and CPF had lower hardness before and after cooking (p<0.05). The variation in the hardness of IMFs could be due to the difference in the deformation of proteins and their interaction with SA during processing. Hence, further research is needed. Due to the presence of gluten form and soft gel with a more open and airy structure, reduction was caused hardness in WPF and CPF. The strong interaction of PPI with SA might have disrupted during cooking.
It has been observed that all the fibers in the fresh IMF have significant differences in terms of shear to the CM (Fig. 4). These differences in WBSF could be attributed to several key factors including the difference in the protein structural composition and behavior with SA as the myofibers organized in a hierarchical order. In contrast, during fiber formation, SA and calcium chloride lead to the formation of a rigid gel matrix. However, the presence of high moisture could increase the tenderness, but it might have contradicted by the formation of a rigid gel (Foegeding et al., 2011). On the other hand, the WBSF of cooked WPF, and CPF haven’t shown a significant difference in comparison to cooked CM (p>0.05). However, WBSF has increased significantly in cooked CMF (p<0.05). It could be attributed to denaturation of WP (unfolding) and gelatinization of starch present in wheat to form a gel-like soft structure leading to the reduction of shear force (Aguilera, 2022; Biliaderis, 2009; Foegeding et al., 2011; Singh et al., 2010). The use of SA and calcium chloride may also have affected the mechanical properties of the products. It is considered that determination of the actual cause behind these variations should be found out in future study.
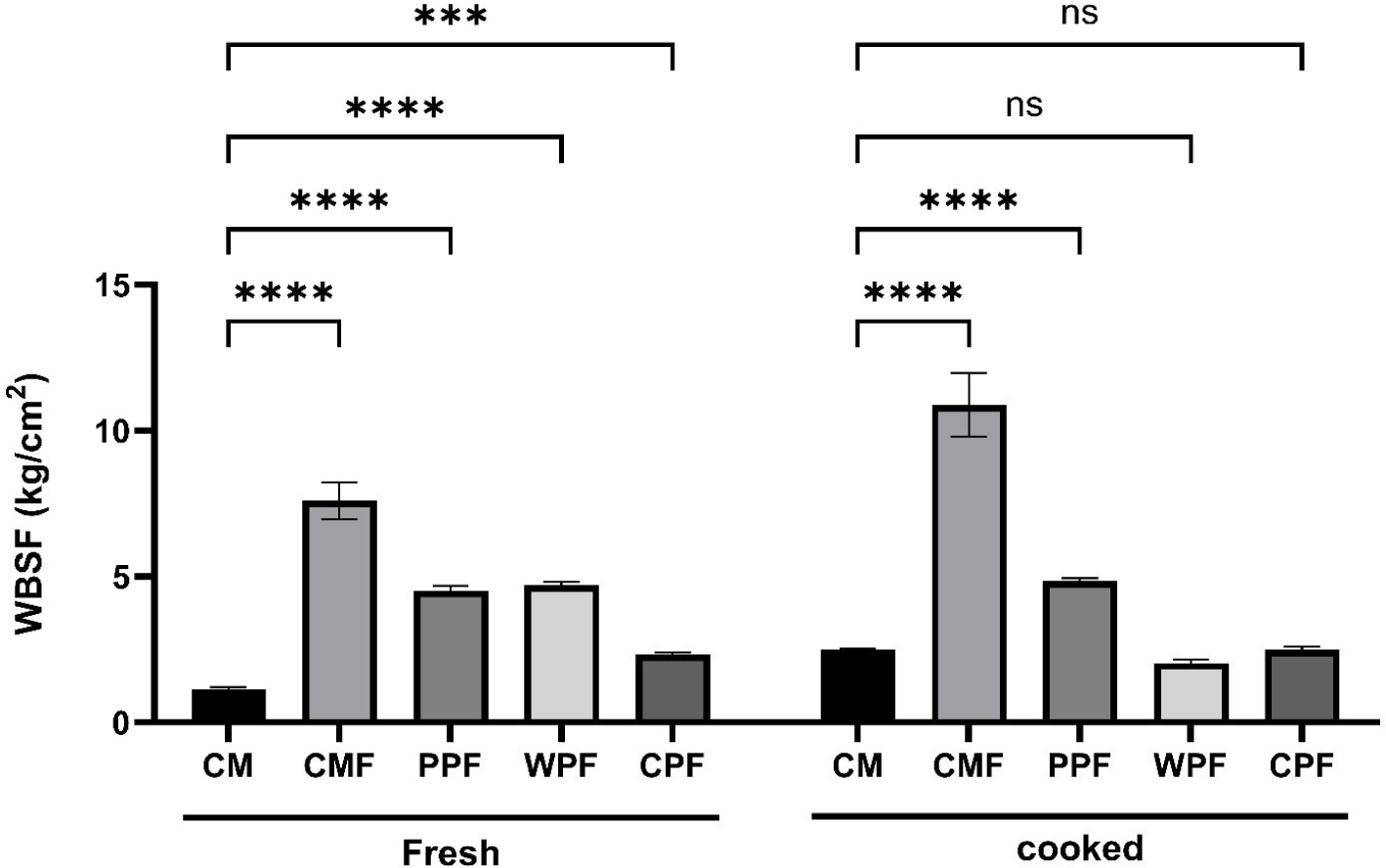
The wet spinning machine processed 700 mL of spinning solution over a time duration of 50 min and yielded different amounts of final product from all the samples mentioned in Fig. 5. With the help of Eq. (2), the process efficiency per minute is calculated. It was found that the processing efficiency with the PPI solution was about 3.63 g per minute, indicating the conversion rate of input to output. However, the conversion rate of CPF, WPF, and CMP was 3.4, 3.2, and 2.8 g per minute respectively. These difference in the process efficiency depend on several critical factors related to the characteristics of the solution and the operational parameters of the machine. Here, during this process, it was noted that due to the differences in the characteristics of WP and PPI like the formation of lumps in WP solution. As WP tends to form viscoelastic networks and increase the resistance to flow, which also affects the consistency of the solution (Wang, 2014). PPI had lower solubility which is a challenge to form a homogenous mixture and required continuous mixing. Additionally, the CMP solution caused clogging of the spinneret leading to lower operational efficiency due to high levels of suspended fiber and the fibrous nature of protein. All these deviations highlight areas for process optimization, such as viscosity reduction and temperature management, to enhance the machine's operational efficiency (Chen et al., 2021). Further research should be done to improve the process operation efficiency of the wet spinning for industrialization.
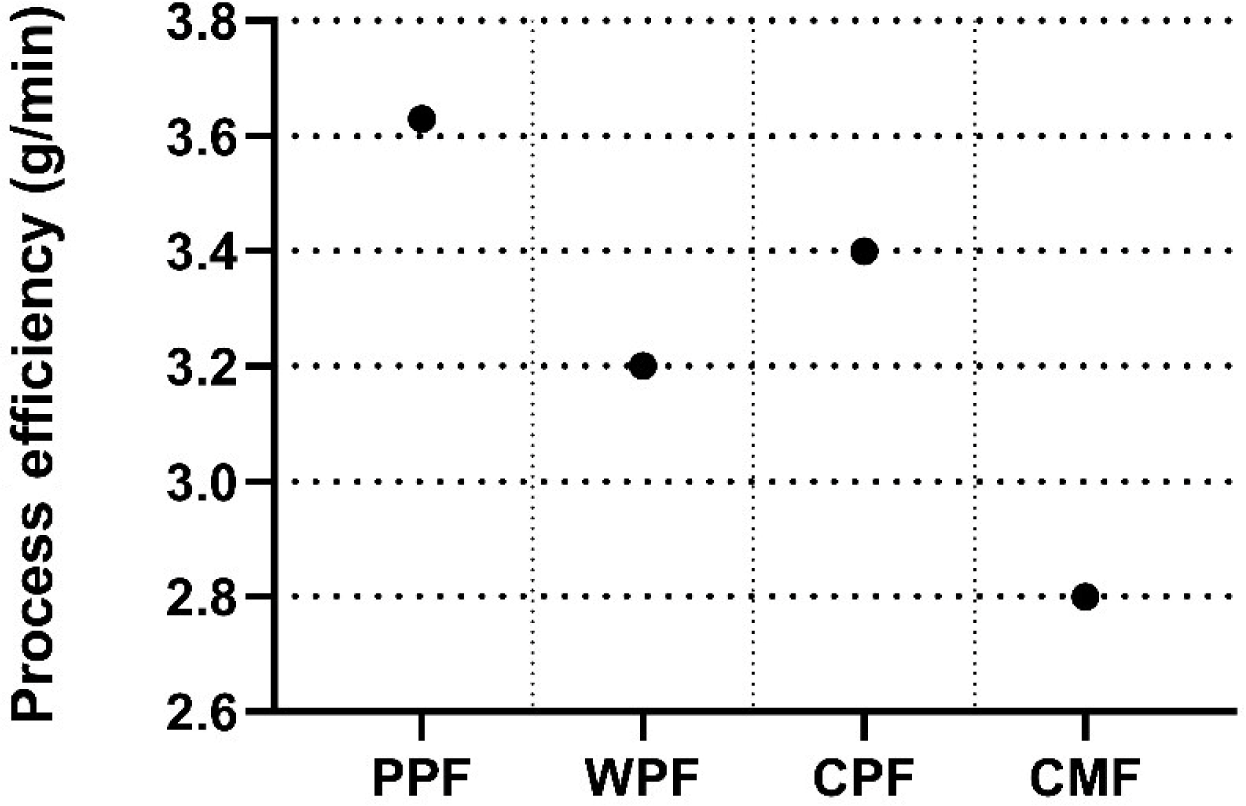
Conclusion
In this study, PPI, WP, and CM paste were utilized with SA to fabricate fiber to mimic the animal muscle fiber by wet spinning process. It was seen that the processing speed of the fiber was strongly dependent on the type of protein sources. The physicochemical and textural profile analysis displayed the difference between the meat quality parameters. While the use of WP has shown promising quality in achieving the desired product, but not up to the mark of CM. Therefore, further analysis to find out how the protein deformation occurs during cooking in the presence of SA is needed. It is concluded that the use of a single protein source cannot create fiber similar to meat fiber. However, optimization of the different protein sources is essential to achieve similar profiling.