Introduction
Due to its high nutritional value and unique flavor, goat meat has gained popularity as an alternative protein source worldwide, with a particular preference in Muslim-majority countries such as Iran, Pakistan, and Indonesia, as well as in regions where beef consumption is limited, including India, Myanmar, Thailand, and Vietnam (Dhanda et al., 2003; Liang and Paengkoum, 2019). Compared to other red meats, goat meat had a favorable protein-to-fat ratio due to its very low intramuscular fat content per gram of meat (≤2%), providing a healthier meat option (Gawat et al., 2023). A Thai-Native (TN)×Anglo-Nubian (AN) goat is one of the crossbreds in Thailand that has been extensively researched and farmed due to its great adaptability against harsh environments, higher multiple birth rate, body size, and yield (milk and carcass) compared to other crossbreds (Chartdaeng et al., 2007; Pralomkarn et al., 2012). Current research on TN×AN goats has primarily focused on improving growth and muscle quality through various feed supplements (Raksasiri et al., 2019; Taethaisong et al., 2023), the castration method (Phonmun et al., 2012), as well as breeds and rearing systems (Khaokhaikaew et al., 2010). However, there is limited information regarding the meat quality of this goat breed, whereas there is none about the cooked meat quality of TN×AN goat meat. In general, different meat muscles produce different muscle profiles, influencing both eating and nutritional qualities. Bicep femoris (BF) muscle is commonly preferred for its intense flavor and taste in red meat delicacies, despite being moderately tender (Carmack et al., 1995). BF contains more type I muscle fibers than longissimus dorsi, giving it a higher red color intensity (Ismail et al., 2019). The BF also exhibits a high free amino acid (FAA) content and water holding capacity, making it suitable for roast and grill cooking (Ali et al., 2021; Ismail et al., 2019). Notably, lysine (Lys) and alanine (Ala) were found to be predominant amino acids in BF, contributing to its salty and sweet taste, respectively. Furthermore, BF from goats had a higher taurine (Tau) content, making it an appealing option for health-conscious consumers (Ali et al., 2021).
Meats are subjected to thermal processes prior to their consumption, ensuring their safety, nutritional, and eating qualities. Textural changes, moisture loss, fat melting, structural shrinkage, browning development, and the formation of specific cooked aromas and oxidation products can vary with the method of meat cooking (Alfaifi et al., 2023; Putra et al., 2019). The multi-directional reactions of those mechanisms would produce a complex mixture of volatile and non-volatile compounds in various ways, resulting in distinct meat tastes and flavors. This includes the Maillard reaction, lipid oxidation, and the degradation of nitrogenous compounds (Bassam et al., 2022). Grilling, a conventional meat cookery, has been widely used due to its ease of use and desirable final product flavor. However, the consumption of grilled meat and the production of hazardous compounds may result in health problems, such as cancer (Bassam et al., 2022). As a result, modern and advanced meat cookery, such as sous vide and microwave, has been developed to minimize the formation of hazardous compounds while preserving desirable qualities. Ortuño et al. (2021) noted that SV cooking can retard the formation of malondialdehyde, 7α- and 7β-hydroxycholesterol, and the cholesterol oxidation ratio during cooking, indicating lipid oxidation prevention by this technique. It was also discovered that the volatile compounds of lamb patties cooked by SV (75°C, 35 min) did not differ significantly from those cooked by grilling (150°C, 72°C core temperature; Ortuño et al., 2021). On the other hand, microwave was a superior cooking method because of its rapid heat generation within the meat, which reduced meat quality degradation and energy consumption. It also produced relatively low total volatile nitrogen-nitrosamines and polycyclic aromatic hydrocarbons (Alfaifi et al., 2023). Technically, sous vide and microwave could reduce water loss, preserve volatile flavor, odor, and other sensory qualities, as well as improve cooking yield more than the conventional method (Ismail et al., 2019; Liu et al., 2021; Pongsetkul et al., 2022).
Flavor is an essential food characteristic formed by the combination of taste (relative to non-volatile compounds), odor (relative to volatile compounds), and chemesthetic sensations (Menis-Henrique, 2020). It is strongly linked to sensory profiles, which determine its acceptability. The meat flavor precursors essentially consist of small molecules of water-soluble metabolites such as FAAs, nucleotides, peptides, sugars, thiamine, and acids, playing a role in Maillard, Strecker, and other reactions (Aung et al., 2023). Cooked products generally contain diverse and distinct volatile and non-volatile profiles, influenced by many factors, including cooking methods, cooking conditions, etc. For instance, the electrically heated air method yielded more non-volatile compounds with a higher equivalent umami concentration and were associated with higher sensory acceptability than the other cooking methods used in cooked lamb (Liu et al., 2021). Pongsetkul et al. (2022) discovered that sous vide reduced up to 53% of trimethylamine (ammonia, a fishy, pungent-related odorous compound) in cooked fish meats, indicating a better delay in the development of undesirable compounds. This information revealed that different cooking methods were associated with flavor development in cooked products to varying degrees. Therefore, the purpose of this study was to comprehensively characterize and compare both non-volatile and volatile compounds in TN×AN goat meat as affected by different cooking methods (sous vide, grilling, and microwave) in relation to flavor development.
Materials and Methods
Twenty-five crossbreds of 50% TN×AN male goat with an average weight of 26.22±3.09 kg and an approximate age of 3–4 months, were purchased from 3 different farms (Nakhon Ratchasima, Thailand) and then slaughtered in a commercial slaughterhouse. After 24 h of cooling the carcass at 2°C, the right and left BF (hindquarter muscles) were collected. After removing the surface fat and connective tissue, the meats were cut into 1.5 cm thickness (each weighing approximately 120–150 g) and then randomly divided into 4 groups based on different cooking methods including raw/uncooked (‘Raw’), grilling (‘GR’), sous-vide boiling (‘SV’), and microwave heating (‘MW’).
The optimal cooking conditions were based on previous studies and no seasoning or marinating process was applied. A temperature data logger (Series II, ThermaData-K, Chandler, AZ, USA) integrated with a thermocouple probe, was placed into the samples to maintain the cooking temperature. For the GR, briefly, a plate grill (ELECTROLUX ETTG1-40BK, Electrolux, Bangkok, Thailand) was used for grilled samples. The plate was heated to 180°C followed by cooking the meats until reached the core temperature of 80°C–82°C for 5 min (Madruga et al., 2009). For the SV, the samples were individually vacuum-sealed (FVC-II, Furukawa MFG, Chiba, Japan) with a vacuum extent of 99.6%. The samples were then cooked in an SV-2447 vacuum cooker (Severin, Sauerland, Germany) at 70°C for 12 h, as per the method of Ismail et al. (2019). For the MW, the samples were placed in a MW oven (EMM20K18GW, Electrolux) at 1,000 W and a frequency of 2,465 MHz for 60 min following the methods of Liu et al. (2021). All procedures have been confirmed on their food safety following the standard of the USDA (2013).
The cooked samples were then stored at room temperature (28°C–30°C) for 30 min to 1 h before being subjected to the analysis of non-volatile components. For the determination of volatiles, the cooked samples were promptly cut into cubes (1.5×1.5×1.5 cm3), vacuumed packed, flash-frozen in liquid nitrogen, and subsequently stored at –80°C until analysis (within a week). All the conditioned samples (GR, SV, and MW) were then analyzed on their characteristics, in comparison to that of Raw.
FAA compositions were determined as per the method of Ali et al. (2021), with some modifications. Briefly, the sample (25 g) was mixed with a 6% (v/v) perchloric acid solution, followed by neutralization with 0.1 M NaOH and subsequent filtration. The filtrates were then analyzed using an amino acid analysis system (Prominence, Shimadzu, Kyoto, Japan), which was equipped with a column (Shim-pack Amino-Li, 100 mm×6.0 mm i.d.; column temperature, 39°C, Shimadzu) and pre-column (Shim-pack ISC-30/S0504 Li, 150 mm×4.0 mm i.d., Shimadzu). FFAs were identified using a fluorescence detector (RF-10AXL, Shimadzu), and the results were expressed in terms of mg/100 g sample.
Nucleotides including adenosine 5′-triphosphate (ATP), adenosine 5′-diphosphate (ADP), adenosine 5′-monophosphate (AMP), inosine 5′-monophosphate (IMP), and guanosine 5′-monophosphate (GMP), as well as some nucleosides (inosine, adenosine, and guanosine) and bases (hypoxanthine, xanthine), were quantified as per the method of Pongsetkul et al. (2017a). The results were expressed as mg nucleotide/100 g sample.
The taste active values (TAVs) and equivalent umami concentrations (EUCs) were calculated to enhance precision in evaluating the taste profiles of the samples, including Glu/Gln, Asp/Asn, 5′-AMP, 5′-IMP, and 5′-GMP (the essential taste precursor compounds). TAVs were calculated as the ratio between the concentration of the compound determined in the samples and its threshold value, generally measured in water or in a simple matrix (Shallenberger, 1993). TAV greater than 1 indicates the taste contribution to the product through an auxiliary effect on the taste. The EUCs were calculated using the following Eq. (1):
Where ai is the concentration of umami amino acids (UAAs; Glu and Asp; g/100 g). The bi was the relative umami coefficients of the UAAs (Glu: 1; Asp: 0.077). Besides, aj and bj represent the levels of taste nucleotides (5′-AMP, 5′-IMP, and 5′-GMP; g/100 g) and their relative umami coefficients (5′-AMP: 0.18; 5′-IMP: 1; 5′-GMP: 2.3), respectively. This value refers to the mixture of monosodium glutamate (MSG)-like amino acids and 5′-nucleotides. The umami intensity (Y) was expressed as g MSG/100 g sample.
The concentrations of sugars, including one disaccharide (maltose) and four monosaccharides (glucose, mannose, fructose, and ribose) were measured using high-performance capillary electrophoresis (HPCE, Agilent Technologies, Santa Clara, CA, USA) with UV detection. The results were expressed as μmol/g sample (Andersen et al., 2003).
The lipid oxidation products, including peroxide value (PV) and thiobarbituric acid reactive substances (TBARS) value, were determined following the method described by Pongsetkul et al. (2017a). To measure the PV, a standard curve of cumene hydroperoxide (0.5–2 ppm) was used. For the TBARS, a standard curve of malonaldehyde bis-(dimethyl acetal) (0–2 ppm) was used. The results were expressed as mg hydroperoxide/kg sample and mg malonaldehyde (MDA)/kg sample, respectively.
Maillard reaction products (MRPs) and browning intensity were measured according to Pongsetkul et al. (2017b), with some modifications. Briefly, the sample (2 g) was homogenized with distilled water (25 mL), then centrifuged to collect the supernatant, followed by a proper dilution. The diluted supernatant was then evaluated for the non-fluorescent and fluorescent intermediate products, as well as browning intensity, by measuring the absorbances at 280, 295, and 420 nm, respectively, using a spectrophotometer (UV-1601, Shimadzu). Moreover, fluorescent intensity was measured at an excitation wavelength of 347 nm and an emission wavelength of 415 nm using a spectrofluorometer (RF-1501, Shimadzu).
The volatile compounds in the samples were determined using solid-phase microextraction gas chromatography-mass spectrometry (SPME GC-MS), according to the method of Madruga et al. (2009) with minor modifications. The minced sample (2 g) was immediately transferred into a 20 mL-headspace vial, tightly capped with a PTFE septum, and heated at 60°C for 2 h to obtain equilibrium. Subsequently, the volatiles were absorbed onto an SPME fiber (50/30 lm DVB/ CarboxenTM/PDMS StableFlexTM, Supelco, Bellefonte, PA, USA) at 60°C for 1 h. Afterward, the SPME device was removed, and the samples were immediately inserted into the injection port of a GC-MS system. The GC-MS analysis was performed using an HP-5890 series II gas chromatography coupled with an HP-5972 mass-selective detector, equipped with a split less injector, and coupled with a quadrupole mass detector (Hewlett Packard, Atlanta, GA, USA). The volatile compounds were identified using ChemStation Library Search (Wiley 275.L) and quantified using an internal calibration curve of stock solutions in ultra-pure water saturated with salt. All identified volatile compounds were estimated and reported as a percentage of the total peak’s relative area.
All analyses were performed in triplicate and subjected to one-way analysis of variance (ANOVA) using the SPSS statistic program (Version 20.0, IBM, Armonk, NY, USA). Differences among all treatment groups were analyzed using Tukey’s test at a confidence level of 95%. In addition, the relationship between all volatile and non-volatile compounds, as well as the various cooked samples, was evaluated using principal component analysis (PCA) to determine the effect of cooking methods on their contribution to the flavor and taste profiles of goat meat.
Results and Discussion
The FAA composition of all cooked goat meats was evaluated based on their contribution to umami, sweet, or bitter taste (Table 1). The total FFAs in Raw (189.13 mg/100 g) were comparable to the previous report (220 mg/100 g; Madruga et al., 2009). Among all FAAs, Glu/Gln, Ala, Gly, Asp/Asn, and Arg were the most abundant FAAs found in all samples. After cooking, a significant decrease in the total FFAs of goat meat (28.4%–34.7%) was observed (p<0.05); however, there was no difference among the different cooking methods used (p>0.05). This may be attributed to protein degradation caused by heat cooking, which involves proteolysis and protein oxidation to a certain degree. Oxidative modifications of proteins can generate the formation of carbonyl compounds, protein crosslinking, cleavage of the protein backbone, and modification of amino acid side chains (Poljanec et al., 2021). In general, the remaining total FAA content after cooking plays a significant role in influencing the flavor and taste of cooked products (Dashdorj et al., 2015). FAAs are one of the substrates for the Maillard reaction or Stecker’s degradation, which leads to the formation of volatile compounds (Bassam et al., 2022).
Considering that each categorized FAA appeared in cooked samples, GR and SV samples yielded the highest total UAAs, with 38.60 and 33.21 mg/100 g, respectively (p<0.05). While the highest sweet amino acids (SAAs) were noted in SV samples: 47.93 mg/100 g (p<0.05), coincidentally, the lowest bitter amino acids (BAAs) were observed in the SV sample (37.35 mg/100 g; p<0.05). These results suggested SV tended to preserve the sweetness of goat meat better than that of GR and MW due to less exposure to the oxidizing environment during cooking. Meanwhile, GR and MW might render a higher degree of protein structure modification at a higher temperature. Different proportions of UAAs, SAAs, and BAAs were presumed to determine the distinct flavor and taste of the cooked goat meat. BAAs could be considered an off-flavor in cooked goat meat that was related to protein oxidation, Cys and Met metabolism (Jia et al., 2021). Ma et al. (2020) stated that Met and Cys can be degraded during heating at a certain level and transformed to hydrogen sulfide, methyl mercaptan, and methylthialdehyde, thus increasing the aroma of meat. However, there was no difference in Met and Cys content among all cooked samples (p>0.05). Lys had a high reactivity in the Maillard reaction, allowing a faster formation of pleasant roasted flavor and appearance, compared to other substrates (i.e., Met and Cys; Zhang et al., 2022). The SV sample had a Lys content (8.09 mg/100 g) higher than the GR and MW samples (p<0.05), suggesting that the meat underwent a lower level of Maillard reaction when processed using the SV technique (p<0.05). The Maillard reaction typically occurs at a temperature of ≥140°C (Bassam et al., 2022), and for this reason, SV may not be sufficient to facilitate the reaction; thus, the Lys content in the SV sample was similar to that in Raw. The higher Lys residue in GR and MW may contribute to a desirable flavor and taste; however, it could also indicate a potential negative impact on the nutritional value, as Lys is an essential amino acid (EAA) for human health (Adeyeye, 2008). In terms of nutritional aspects, the total EAAs and non-EAAs (NEAAs) in the cooked goat meats (GR, SV, and MW) were significantly lower than those in the Raw (p<0.05). However, the World Health Organization recommended that total EAAs and total FAAs above 26% and 11% were adequate for ideal protein intake for children and adults, respectively (WHO, 1985). Therefore, the ratio of total EAAs to total FAAs in all samples, both raw and cooked meat, accounting for over 35%, suggested that goat meat provided favorable AA profiles and satisfactory nutritional values. The Leu/Ile ratio is very important for controlling the metabolism of Trp and niacin as a process in metabolic disease development, particularly pellagra (Adeyeye, 2008). Here, the Leu/Ile ratio was found to be lower (1.03) in the SV sample compared to others (1.15–1.24; p<0.05), indicating good protein quality in terms of nutrition. An excess or imbalance of Leu can also impact protein utilization, as measured by the predicted protein efficiency ratio (P-PER). Our results showed that the P-PER was lower after cooking compared to the Raw sample. The SV-cooked sample had the lowest value (p<0.05), which means that the protein was harder to digest when the meat was heated, especially when the SV technique was used. This could be caused by protein oxidation and degradation that occurred during cooking, leading to meat exudates (water-soluble compounds) governing a dilution effect on nutritional value (Madruga et al., 2009). Roldán et al. (2013) reported that heat cooking temperatures above 60°C induced muscle fibers to shrink longitudinally, leading to increasingly higher water loss and containing several essential nutrients. Nevertheless, all samples had a P-PER in the ranges of 2.82–4.57, indicating a favorable level. Hoffman and Falvo (2004) noted that a P-PER exceeding 2.7, considered the standard value of casein, is regarded as an excellent protein source. Therefore, different heat cooking rendered various FAA compositions, which were related to both the flavor development and nutritional value of goat meat to varying degrees.
ATP breakdown occurs gradually in the postmortem state of the animal indicating meat freshness. It starts with the depletion of muscle glycogen, leading to the quick degradation of ATP. The ATP is hydrolyzed into ADP by ATPase, followed by sequential breakdown into AMP, IMP, and inosinic acid, then accumulates via AMP deamination. These breakdown products can either cause meat to putrefy or enhance its taste, leading to sensory acceptance. For instance, inosinic acid enhances the umami taste of Glu, whereas hypoxanthine contributes to the bitterness of meat (Utama et al., 2018). Notably, ATP in all samples was not detected, indicating that ATP had degraded completely during aging (for 24 h) after postmortem (Table 2). The rapid degradation of ATP to form its metabolites during the aging stage of meat, including pork, beef, and chicken, was noted in previous studies (Aliani et al., 2013; Ferguson and Gerrard, 2014), which coincided with our results. The disappearance of ADP and lower AMP levels in cooked products (GR, SV, and MW; p<0.05) compared to Raw sample suggested that heating accelerated this breakdown process. However, it could be observed that different cooking methods generated varying levels of ATP breakdown into nucleotides. Among all nucleotides, IMP and inosine were found to be dominant in all samples. IMP and GMP are the flavor-potentiating nucleotides that can enhance the umami taste of meat and display a synergism with L-glutamate. This leads to an increase in palatability as the intensity of the meat taste develops. Moreover, those nucleotides contributed to the brothy and meaty flavor (Dashdorj et al., 2015). GMP was present at a low level and significantly reduced by heat cooking, decreasing from 5.12 mg/100 g in the Raw to 0.54–1.01 mg/100 g in cooked samples (p<0.05). Nevertheless, the low amount of GMP present in the cooked samples remained potential to enhance the flavor effectively since it was reported to be 2.3-folds more active than IMP (Dashdorj et al., 2015). Among all samples, MW achieved the lowest IMP (34.34 mg/100 g) and GMP (0.54 mg/100 g), indicating that microwave cooking may have the most potential to accelerate purine degradation. This coincided with the highest xanthine (10.66 mg/100 g) compared to others (p<0.05). Fukuuchi et al. (2018) also suggested that IMP and GMP were more resistant to decomposition by boiling than microwave heating. Interestingly, GR exhibited the highest hypoxanthine with low GMP compared to SV and MW (p<0.05), suggesting that it might possess the most pronounced bitter taste among all the samples. This might imply the highest purine degradation rate due to the high cooking temperature of the GR used (180°C). At the same time, GR could induce a moderate degradation of AMP due to its moderate adenosine content. Overall, different cooking methods resulted in distinct nucleotide profiles. In GR, hypoxanthine was the dominant nucleotide, while SV displayed primary purine degradation with IMP, inosine, and adenosine as major constituents and low guanosine. In contrast, MW possessed both IMP and AMP degradation, with inosine, xanthine, and adenosine as the main degradation products. This is correlated to various taste and flavor characteristics of the products as affected by cooking methods. Compared among cooked products, GR and MW had the highest and lowest total nucleotides, accounting for 154.19 and 112.69 mg/100 g, respectively. Carmack et al. (1995) noted that the total nucleotides, which are normally reduced during cooking, indicate the occurrence of complex biochemical reactions related to the flavor and taste development of the final products. The lowest total nucleotides in MW might infer to the less desirable taste and flavor governed by ATP breakdown products. MW might induce undesirable textural and flavor modifications such as charring, drying, excessive evaporation, hardening, and the development of burnt or raw flavor and aroma notes (Ibrahim et al., 2012). Although GR had the highest bitterness, this sample had the highest total nucleotides. This might suggest that grilling could remain preferable due to the pleasant flavor formed during GR that involved other biochemical reactions.
The most umami taste-contributing amino acids (Asp/Asn and Glu/Gln) and 5′-nucleotides (AMP, IMP, and GMP) were used to evaluate the TAVs and EUC of samples (Fig. 1). The higher TAV indicates a potent synergistic effect on umami development, with a value greater than 1 implying that the component contributes to the umami taste (Aung et al., 2023). From the results, IMP and Glu/Gln achieved the highest TAVs in all samples, rendering their major contribution to the umami taste in goat meat products. Impacts of IMP have been reported to include enhancing distinct flavor attributes in meat, such as brothy and meaty, as well as improving taste in combination with MSG and GMP (Dashdorj et al., 2015). Raw had the highest TAV on IMP (4.08), followed by GR (2.65), SV (2.00), and MW (1.37), which was in line with the amount of UAAs (Table 2). A similar trend was observed for Glu/Gln, but it had a lesser contribution to the umami taste than IMP in all samples tested, as indicated by lower TAVs, despite being the most abundant FAAs (Table 1). Higher TAVs in Raw sample, compared to cooked samples suggested that the cooked samples underwent extensive degradation with molecular modifications induced by heating through different mechanisms, resulting in the degradation or disappearance of these desirable flavor and taste compounds. Correspondingly, the greatest EUC was obtained by Raw (6.00 g MSG/100 g) compared to others (Fig. 1B). The decrease in EUC values in the cooked samples might be due to the loss of water-soluble compounds during heat cooking, as previously discussed. Among the cooked samples, GR possessed a higher EUC (2.49 g MSG/100 g), suggesting the grilling temperature was more capable to generate umami tastes than those of SV and MW (lower temperature). Previous reports have given a clear view that umami taste is more pronounced at high cooking temperatures with the attainment of a desirable level of doneness in meat (Hwang et al., 2020; Wang et al., 2023). Although Raw had the highest EUC, uncooked meat was reported to have a blood-like taste (Rotola-Pukkila et al., 2015), and most populations generally prefer consuming cooked goat meat, considering its palatability and safety aspects (Alfaifi et al., 2023; Dhanda et al., 2003; Putra et al., 2019). Besides, raw meat does not demonstrate a great deal of flavor. Hwang et al. (2020) declared the ambiguity of umami taste as compared to sweet and salty due to its formation through serial chemical reactions between compounds. Although umami is the typical taste of MSG, it is not very palatable, whereas Yamaguchi et al. (1971) suggested that the desirable umami taste is influenced by the synergistic mechanism between IMP and Glu in the food system. Furthermore, not only nucleotides and FAAs, but the flavor and taste of cooked products are also influenced by other compounds, including the breakdown of sugars and lipid oxidation, which are related to the release of aroma from volatile compounds (Aliani et al., 2013; Pongsetkul et al., 2022; Wang et al., 2023).
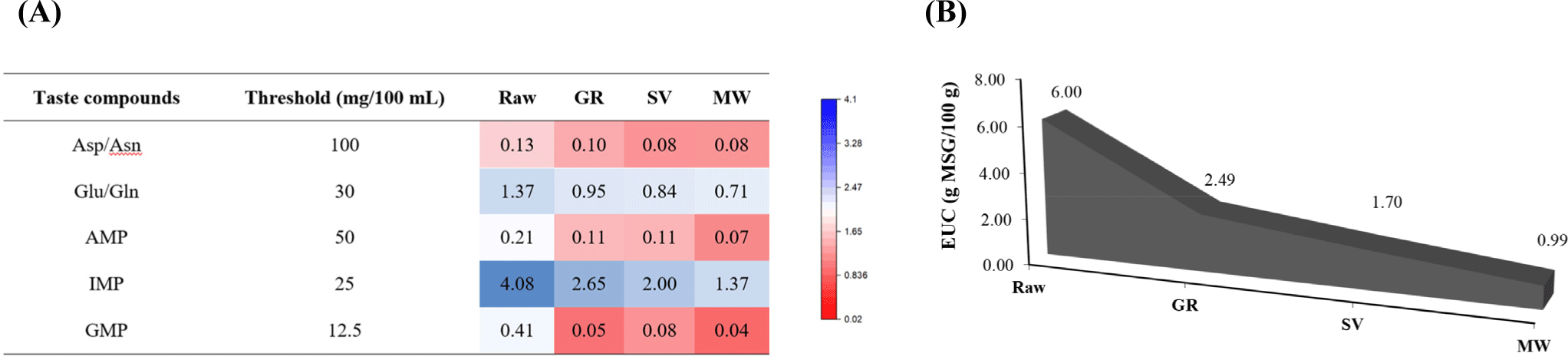
Reducing sugars in meat tissues are derived from carbohydrate breakdown, primarily in the form of polysaccharide-glycogen, glycoproteins, and mucopolysaccharides (Ivanović et al., 2016). These compounds typically contribute to the flavor development of meat during cooking. For example, glucose and ribose can react with α-amino acids and interact with lipids to generate the aroma compounds via the Maillard reaction and lipid oxidation, respectively (Roldán et al., 2013; Van Ba et al., 2012). The concentrations of sugars (including maltose, glucose, mannose, fructose, and ribose) in goat meat samples are presented in Table 2. Glucose and fructose were the most abundant sugars found in all samples. The amount of sugar associated with the flavor and taste of the goat meats has been reported in some previous studies. A significant loss of fructose, glucose, IMP, and Cys detected in cooked samples was reasonable for the formation of aroma in goat meat (Madruga et al., 2009). Mannose-6-phosphate, glucose, and myo-inositol could suppress unpleasant flavors in lamb and sheep meat (Grabež et al., 2019). Based on our results, the total reducing sugar contents in the Raw sample were significantly diminished from 310.50 μmol/g to 166.70–98.45 μmol/g after cooking (p<0.05). This indicated the advancement of the Maillard reaction in meat, where reducing sugars were utilized during heat treatment, leading to the formation of brown pigments and aromatic compounds, particularly on the meat’s surface when grilling or using microwave heating (Yoo et al., 2020). SV displayed the highest total sugar content among all cooked meats (p<0.05). This was attributed to the absence of extremely high surface temperatures (~60°C) and the resulting lack of surface dehydration, which led to lower levels of Maillard reaction development (Ruiz-Carrascal et al., 2019). Nevertheless, extensive heating at low temperatures could facilitate the interaction between sugars (xylose, glucose, and maltose) and thiamine degradation compounds, thus generating aromas and flavors in SV products, such as furan (Bleicher et al., 2022), which found at certain levels in our SV sample. Besides, the lowest sugar content in GR (p<0.05) suggested that some sugars may have undergone caramelization, resulting in an intensely burnt appearance and flavor (Bassam et al., 2022). In addition, higher moisture loss during rendering result in a faster rate of Maillard reaction, resulting in increased roasted odor notes, which are typical characteristics of grilled meat (Van Ba et al., 2012; Yoo et al., 2020). MW showed a moderate loss of sugar content, indicating the sugars have undergone interaction with other taste-active compounds present in goat meat via hydrogen bonds and dipolar rotation in an electric field (Ibrahim et al., 2012). These describe the distinct flavor and taste of cooked meat produced by various cooking methods.
PV and TBARS measure the hydroperoxides and aldehydes as indicators of primary and secondary lipid oxidation, respectively. Hydroperoxides are unstable and decompose rapidly, resulting in a reduction of their content as the oxidation rate increases. In contrast, aldehydes are stable and display rancid aromas in a low amount in meat (Domínguez et al., 2019). Fig. 2 demonstrates that Raw had the lowest PV and TBARS among all samples, accounting for 0.32 mg hydroperoxide/kg sample and 0.25 mg MDA/kg sample, respectively, indicating its freshness with less lipid oxidation occurring (p<0.05). Similarly, it was reported that the fresh or raw goat meat contained low PV (0.18 mg hydroperoxide/kg sample) and TBARS (0.17–0.25 mg MDA/kg sample; Aung et al., 2023; Jia et al., 2021). A dramatic increase in PV and TBARS was noted in the samples after cooking (p<0.05). Among all cooked samples, SV had the highest PV (p<0.05) with a low TBARS, indicating a higher level of hydroperoxide formation compared to the decomposition into secondary products when meat was processed with the SV technique. Notably, a low PV might represent both early and advanced oxidation (Domínguez et al., 2019). There was no difference in PV between GR and MW (p>0.05), however, GR had the highest TBARS (1.46 mg MDA/kg sample) among all samples (p<0.05). These results implied that high temperature cooking as used in GR rendered a faster decomposition of hydroperoxide, resulting in a higher aldehyde content and rancidity level. In contrast, SV and MW could retard the rancidity during goat meat cooking due to a lower temperature than GR used. Moreover, a lower available oxygen level during SV cooking might have partially limited secondary lipid oxidation during cooking and prevented its further development during heated display (Ortuño et al., 2021). The secondary products of lipid oxidation (i.e., alcohol, aldehydes, ketones, hydrocarbons, acids, esters, etc.) impart flavor and taste development in meat, which are desirable at a particular threshold (Liu et al., 2021; Madruga et al., 2009). Liu et al. (2024) stated that sulfur compounds, in association with lipid-derived aldehydes and sulfur-containing amino acids, are responsible for creating a roasted flavor in GR. The interaction between predominant aldehydes (pentanal, hexanal, and heptanal) and Strecker degradation of amino acids, could generate the sweet aroma and grassy notes in cooked lamb meat (Liu et al., 2021).
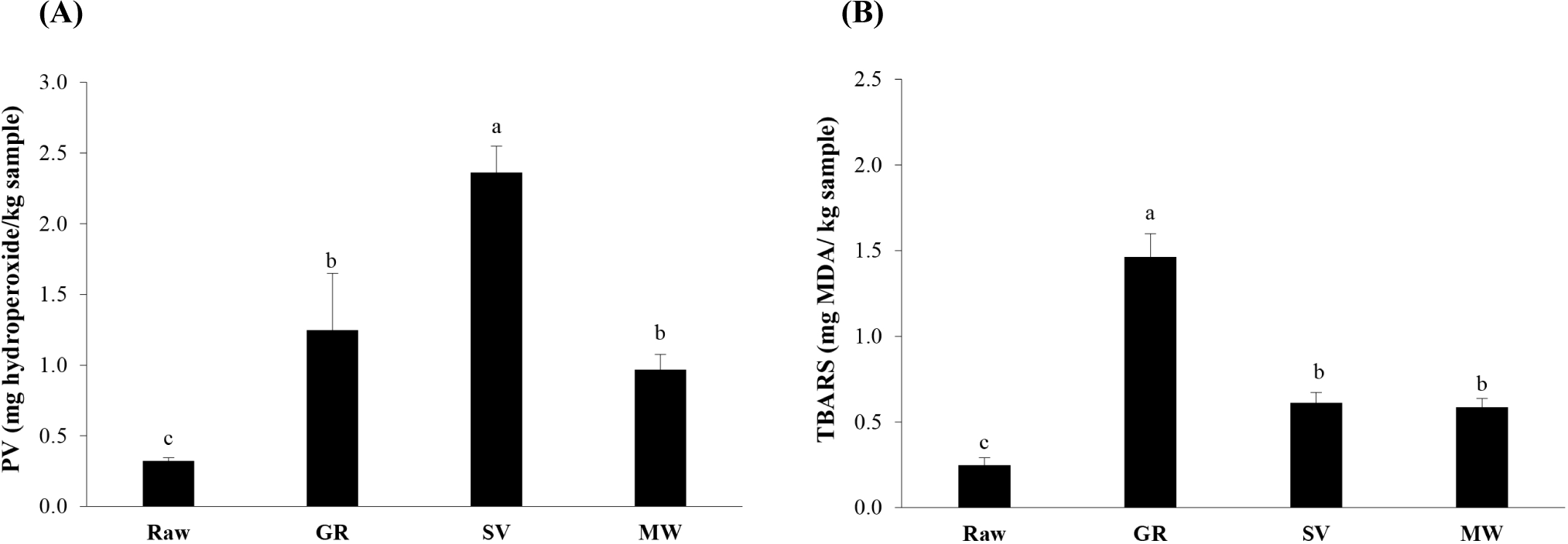
Fig. 3 shows the varying MRPs’ intensities, including intermediate products as determined by non-fluorescent (A280, A295) and fluorescent intensities, as well as final products as determined by the browning intensity (A420) of goat meat samples, as influenced by different cooking methods. All cooked products exhibited higher intensities of all MRPs compared to the Raw, suggesting an obvious impact of heating on accelerating the Maillard reaction. The lowest intensities observed at A280 and A295 in the Raw (Figs. 3A and B) may be explained by the highest total sugar (Table 2) and FAAs (Table 1) content, indicating a low occurrence of the Maillard reaction in fresh meat, particularly at the initial stage. Interestingly, the highest non-fluorescent MRPs were observed in MW, followed by GR, SV, and Raw, respectively (p<0.05). A similar trend was also found for the fluorescent intensity (Fig. 3C). This suggested that MW-cooking can facilitate the formation of Maillard reaction intermediate compounds to a greater extent than other cooking methods. This could be associated with the generation of high temperature, pressure, and superheating within the matrix during MW-cooking, which accelerates simple or single-step chemical reactions like esterification, hydrolysis, and cyclization reactions (Ibrahim et al., 2012). Generally, these intermediate products are responsible for eventually turning into the final brown pigments. For example, the reaction between glucose-Ala and glucose-Gly generated the fluorescent MRPs and was considered an indicator of the level of the advanced glycosylation end-modified proteins (Matiacevich et al., 2005). The hydroxymethylfurfural, furosine, N-ε-(carboxymethyl) lysine, etc. were reported to contribute as the precursors to the formation of melanoidins and brown nitrogenous polymers (Ibrahim et al., 2012). The browning intensity of MW was observed at a certain level (0.49; Fig. 3D). However, when comparing samples, GR exhibited the highest browning intensity (0.73; p<0.05), while SV and Raw showed comparable lower intensities. The rapid Maillard reaction that occurred in GR was in accordance with the lowest remaining amounts of predominant Maillard substrates [Lys and reducing sugars (ribose and glucose)] found in this sample (Tables 1 and 2). GR-cooking was reported to perceive an intense brown appearance (melanoidin) with a distinct aroma due to the generation of volatile compounds such as pleasant floral, nutty, caramel, attractive meat, and spicy (Liu et al., 2022). Additionally, the lower browning intensity in SV may be described by its lower cooking temperature, as the Maillard reaction is known to be accelerated by higher temperatures (Liu et al., 2022). Although SV-cooked products seem to lack strong browning on the surface and roasted flavor notes, this cooking technique can help to prevent surface dehydration, myoglobin oxidation, and protein denaturation due to the moist environment with limited oxygen availability, as reported by Ortuño et al. (2021).
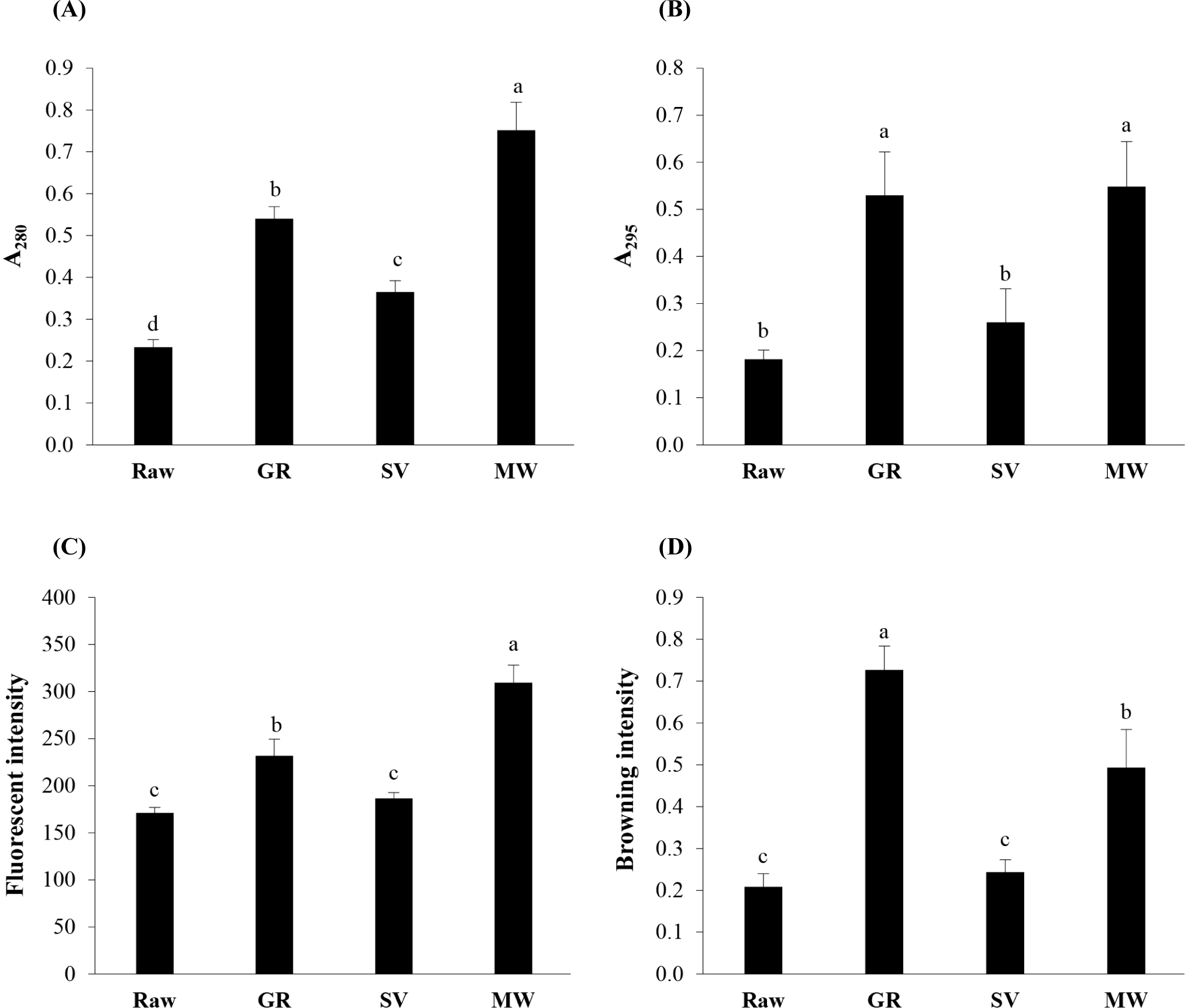
The total intensity (% peak area) of volatiles in samples, categorized by their sources and chemical families, is presented in Figs. 4A and B, respectively. Notably, lipid-derived compounds (i.e., aldehydes, ketones, alcohols, and hydrocarbons) were more abundant than those of Maillard-derived compounds (i.e., pyrazine, pyrroles, pyridines, and thiazoles) for all samples tested (Fig. 4A). Raw sample showed the highest total lipid-derived compounds (84.96%) with the lowest Maillard-derived compounds (15.04%), suggesting that lipid compounds and their changes played a more significant role in the odor or flavor of the raw goat meat. However, an increase in the ratio of Maillard- to lipid-derived compounds was observed for all cooked samples, with the highest proportion of total Maillard-derived compounds obtained in GR. This result was in accordance with a higher degree of Maillard reaction occurring in this sample, as indicated by the highest browning intensity (Fig. 3D). Considering the chemical families (Fig. 4B), alcohol, aldehyde, and hydrocarbon, were the major groups in Raw, constituting more than 15%. Notably, the ratio of them changed after cooking. An increase in aldehyde and ketone, as well as nitrogen-containing compounds, was clearly observed in all cooked samples, suggesting lipid oxidation and protein degradation during cooking, respectively. The increased proportion of aldehydes in cooked samples, which corresponded to the higher TBARS (Fig. 2B), could be linked to a loss of meatiness, possibly due to rancidity (Ortuño et al., 2021). While the increased proportion of nitrogen-containing compounds, which may result from protein degradation and the condensation of amino acids with the intermediate products of the Maillard reaction in Strecker degradation, led to the formation of carbonyl total volatile compounds (VOCs), particularly pyrazines and their derivatives, these, along with alcohols, and hydrocarbons, contribute to the rich aroma of cooked meat (Bassam et al., 2022). Moreover, all heat cooking methods in the present study increased the presence of sulfur-containing compounds (>5%), which was responsible for meaty flavor (Liu et al., 2024). The volatile profiles of our cooked samples were similar to those reported by Madruga et al. (2010), who stated that the predominant aroma compounds of the cooked goat meat were aldehydes (Strecker and lipid-derived), alcohols, ketones, pyrazines, and sulfurs.
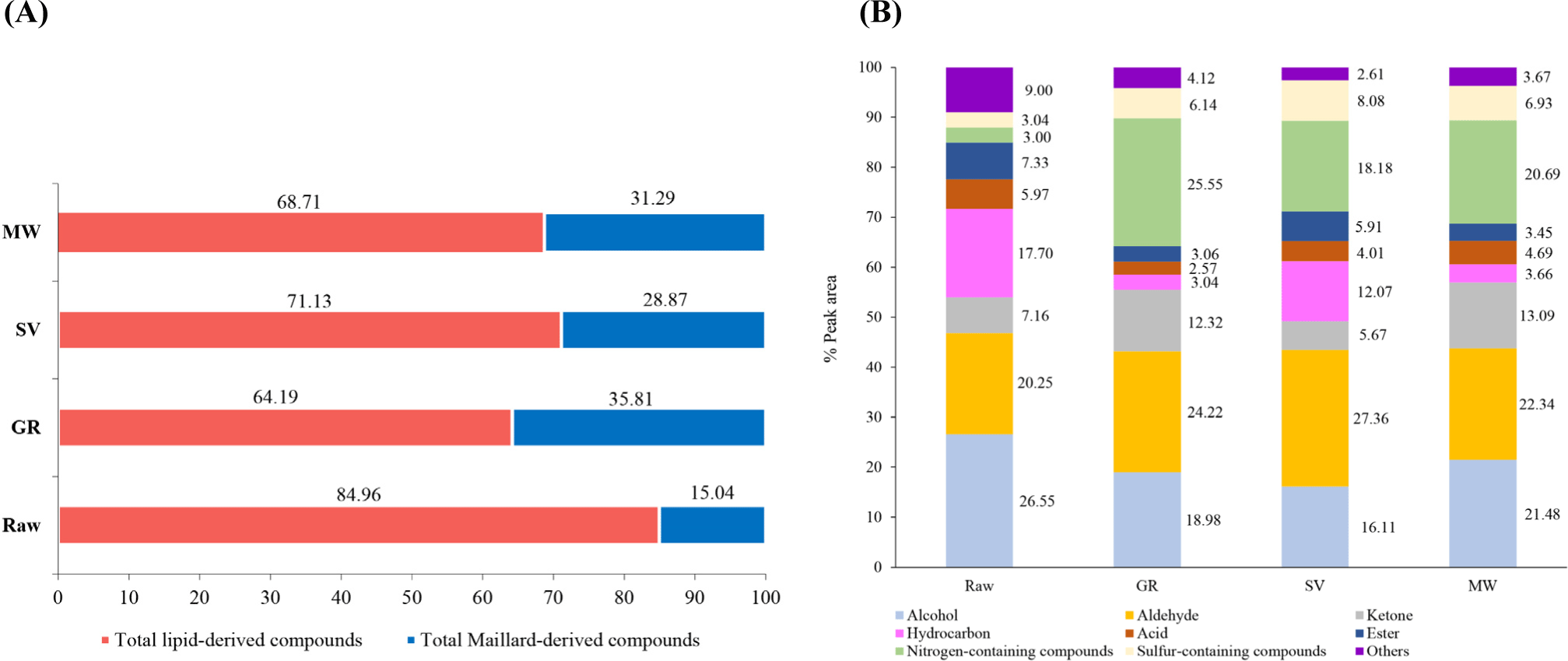
Among all the identified VOCs, VOCs showing more than 2% of the total peak area were reported in Table 3. Different cooking methods resulted in variations in the composition of VOCs in goat meat. For the Raw, 1-butanol, 2-butanol, and 1-octen-ol were the dominant alcohols (>5%), which all were found to be lower after cooking (p<0.05). Also, lower or not detectable hydrocarbons were noted in cooked samples, indicating the chain breakdown of lipids, leading to the formation of other VOCs with a shorter chain. For the cooked goat meats, 2,5(6)-dimethylpyrazine (roast beef), hexanal (green), and 1-octen-3-ol (mushroom), were noted as the most predominant VOCs in GR, SV, and MW, respectively. The predominance of hexanal in SV might be attributed to its multiple synthesis pathways, such as the oxidation of oleic, linoleic, and arachidonic acids (Ortuño et al., 2021). The hexanal/3-methyl-butanal ratio (HX/3MB) was used to evaluate the balance between lipid oxidation and amino acid degradation in meat (Del Pulgar et al., 2013). Here, the HX/3MB ratio of GR (1.75) implied a higher rate of Strecker degradation than those of SV (3.64) and MW (2.08). The higher rate of Strecker degradation in grilled meat, compared to SV-cooked meat, was reported for cooked lamb by Ortuño et al. (2021). Furthermore, Wong et al. (1975) reported that the presence of 4-methyloctanoic acid in goat meat and mutton contributed to an undesirable goaty odor note. Thus, the reduction in the proportion of acids and esters after cooking, particularly GR and MW, such as hexanoic acid and its ethyl esters, suggested the occurrence of alkylation during cooking, potentially contributing to the reduction of the goaty odor to some extent. With this reason, SV may have a more intense goaty flavor than others. This description aligned with the highest aldehyde proportion (Fig. 4B) of the SV sample, which was supported by Roldán et al. (2015), who noted that SV-cooking induced the Strecker degradations of amino acids, resulting in 2-methylpropanal and 3-methylbutanal, leading to a stronger meaty flavor with roast notes. The obviously higher nitrogen-containing heterocycles, such as pyrazines, pyridines, and pyrroles, in the cooked samples could be explained by the reduced Lys amount (Table 1), which had undergone cyclization, decarboxylation, and deamination involved in Maillard and Stecker reactions as well as lipid oxidative degradation (Liu et al., 2022; Zhang et al., 2022). Notably, a significantly higher proportion of 2,5(6)-dimethylpyrazine and pyrrole in GR, followed by MW (p<0.05), was reasonable for aldehyde-amine condensation as well as the formation of heterocyclic nitrogen compounds, contributing to the desirable roast flavor and odor (Liu et al., 2022). For sulfur compounds, SV had the highest proportion of carbon disulfide (p<0.05), which is associated with a burnt cabbage odor, distinguishing it from other cooked samples. In contrast, GR and MW possessed a similar proportion of hydrogen sulfide and methanethiol, resulting in a rotten egg-like odor. Although this odor was unpleasant, it was expected to be masked by more pleasant odor, such as roast and caramel. This explanation coincided with the presence of thiophene in these two samples, indicating that GR and MW displayed a more pungent aroma. The formation of thiophene involved the Strecker degradation of Cys, which producing hydrogen sulfide and ammonia, thus reacting with IMP and ribose degradation products (Madruga et al., 2010). This compound, in combination with other sugars, i.e., mannose-6-phosphate, glucose, and myo-inositol, could suppress the unpleasant flavors in lamb and sheep meat (Grabež et al., 2019).
A PCA biplot was used to assess the interdependence of volatiles and non-volatiles in the flavor development of goat meat under various cooking methods (Fig. 5). The total variability of all the data accounted for 78.70%. PC1 (63.48%) clearly separated the characteristics of the cooked samples, with GR on the right side and SV and MW on the left side, while PC2 (15.22%) encouragingly discriminated between SV and MW, with the top and bottom parts, respectively. On the right side of the biplot, a strong positive correlation was observed between Glu/Gln, Asp/Asn, IMP, UAAs, TBARS, nitrogen-containing compounds, and Maillard volatiles, indicating that they correspond to the GR. This correlation was consistent with the above-mentioned findings regarding GR, wherein grilling had the most potential to accelerate the Maillard reaction, extend lipid oxidation and protein degradation, resulting in enhanced umami flavor development in the final product. Conversely, a positive correlation between lipid volatiles, total sugars, sulfur-containing compounds, PV, and hydrocarbons was observed on the left side of the biplot, which corresponded to the SV. This could suggest that the distinct flavor development in SV was primarily influenced by primary lipid oxidation, with a lower rate of Strecker reactions and protein degradation, resulting in the development of an intensely meaty flavor while preserving some of its original goaty aroma with a hint of umami in the final product. Moreover, it was also observed that none of the volatiles and non-volatiles exhibited a strong correlation with the development of flavor in MW. This might suggest that the characteristics of MW-cooked goat meat may not be as distinctive as those of the other two samples, possibly due to the combination of moderate Maillard and Strecker reactions dominant in grilling and SV-cooking, respectively, both of which occur during microwave processing. Overall, this study highlighted how different cooking methods rendered unique flavor development in terms of the association between volatiles and non-volatiles, which can help describe the variety of flavors and tastes in the products, catering to consumer preferences to some extent.
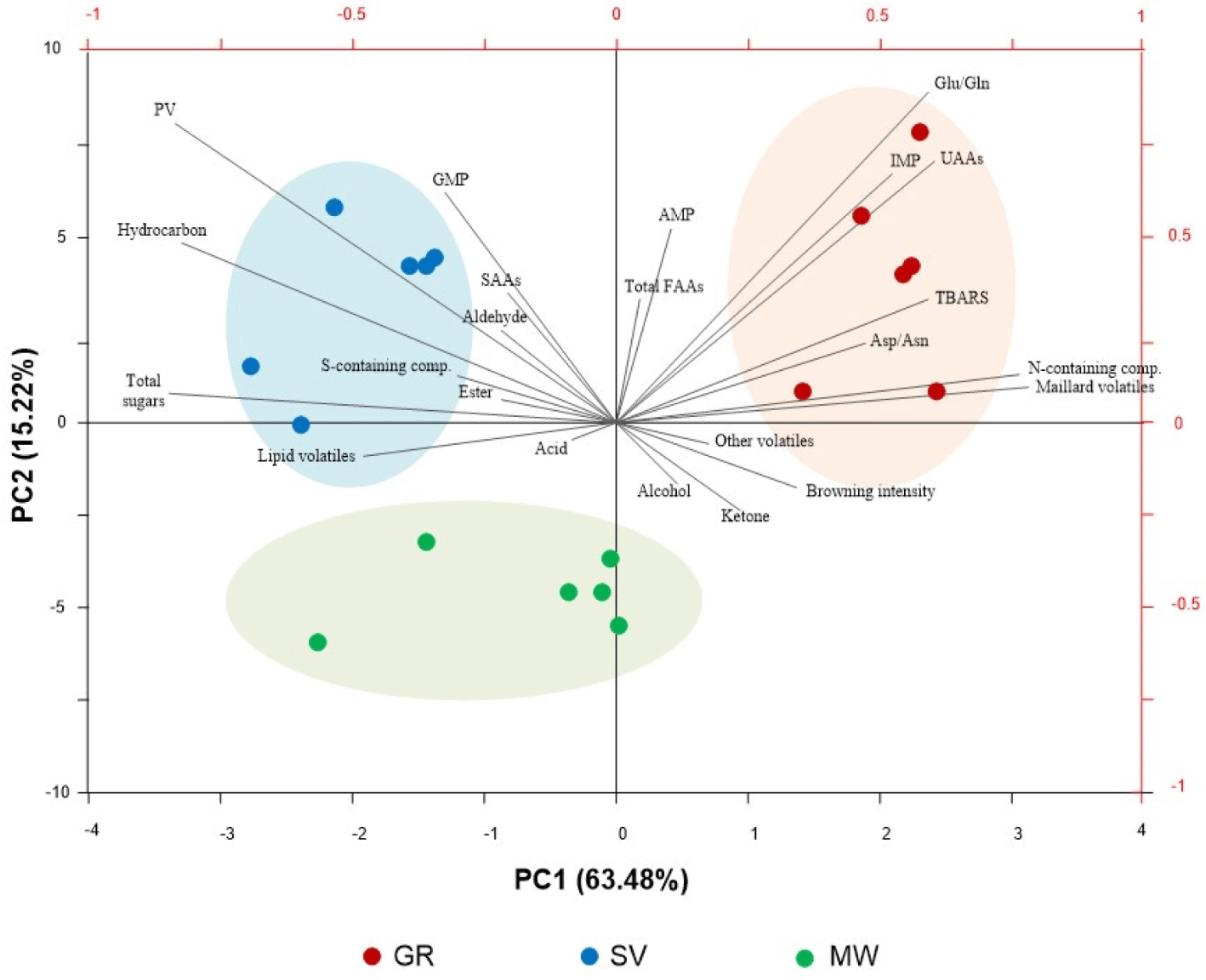
Conclusion
Different cooking methods, including grilling, SV cooking, and microwave heating, had an impact on the flavor development of goat meat in terms of volatiles and non-volatiles. GR was found to have a more palatable flavor and taste than SV and MW due to a greater degree of Maillard reaction, protein, and lipid oxidation. The highest TAVs of Glu/Gln and IMP, as well as EUC, were noted in this sample (p<0.05), confirming its desirable taste and flavor. In contrast, SV exhibited a similar meaty flavor to the Raw, indicating that the low cooking temperature preserved the natural flavor of goat meat while retarding the development of its desirable flavor. Based on PCA results, the associations among volatiles and non-volatiles dominant for each cooking method were reported, linking them to their unique flavor and taste. In light of the flavor development of goat meat, these findings could therefore serve as a basis for determining the suitable cooking method. Moreover, the information related to customer acceptance i.e. sensory evaluation should be taken into consideration in future work, to better comprehend product preferences.