Introduction
Tenderness has been specified as the most significant factor affecting the perception of taste and consumer satisfaction (Naveena and Mendiratta, 2001). Goat meat has less intramuscular fat, less subcutaneous fat, and more intramural body fat, resulting in a leaner and tougher meat than beef and mutton, so it is not generally preferred by consumers. Most of the toughness in the meat occurs due to changes in myofibrillar proteins (the actomyosin effect) or the amounts of connective tissue (background effect; Qihe et al., 2006). The main protein in the connective tissue is collagen, and this is involved in the change in tenderness due to connective tissue being related to the amount of collagen, the perimysium fiber diameter, and cross-linking (Light et al., 1985). In the meat industry, post-mortem aging of meat at chilled temperatures stimulates endogenous proteases to perform the cleavage of myofibrillar proteins, thereby improving tenderness (Lawrie and Ledward, 2006). However, endogenous proteases in meat from mammals do not cleave collagen, which is the main constituent of connective tissue (Purslow, 2005). Keeping meat for 3 weeks at a chilled temperature is a general aging method (Lee et al., 1996). However, this traditional aging process involves considerable chilled space requirements, functional costs, and power consumption (Dransfield, 1994). Therefore, enzymatic methods should be used to improve the softness of the meat with reduced aging time. A relatively advanced method for improving meat quality is the use of exogenous proteases to increase tenderness, which reacts differently on the myofibrillar and connective tissue of the meat. Presently, the USDA’s Food Safety Inspection Service (FSIS) classifies exogenous enzymes as ‘Generally Recognized as Safe (GRAS)’ and contains only five exogenous enzymes that have been studied including proteases from papain, bromelain, ficin, Aspergillus, and Bacillus (Foegeding and Larick, 1986). Most of these are plant-derived enzymes. However, these are limited mainly because of texture problems such as a mushy texture or over-tenderized meat. Therefore, an alternative way to avoid the problem has been reported by using bacterial collagenases replacing non-specific plant proteases for meat tenderization (Foegeding and Larick, 1986).
Amongst several proteases, like collagenase, bacterial proteases are the most important compared to fungal and animal proteases. Bacillus species are non-pathogenic strains and are specific producers of extracellular proteases. Collagenases are the only protease enzymes that degrade peptide bonds in native collagen into small fragments (Howes et al., 2015). Additionally, bacterial collagenases can play a significant role in the hydrolysis of proteins in meat. Sorapukdee et al. (2020) reported that collagenolytic proteases from Bacillus subtilis B13 and Bacillus siamensis S6 were indicated in powerful in vitro hydrolysis toward collagen, elastin, and beef intramuscular collagen with a low degradation of myofibrillar protein in beef. Although enzyme B13 and S6 had the maximum collagenolytic activity at 50°C and 60°C, respectively, they were able to retain 12.6%–25.2% of the relative activity at 4°C–20°C (Fig. 1). From reports from Zhao et al. (2008) and Zhao et al. (2012), they also stated that cold-adapted collagenolytic protease MCP-01, was extracted from Psudosciaena polyactis, had ability to maintain 12.4%–24.2% of the highest activity at 0°C–25°C. This cold adapted MCP-01 also showed higher activity at low temperatures (0°C–25°C) than the collagenase from Clostridium histolyticum that classified as the mesophilic enzyme (Zhao et al., 2008; Zhao et al., 2012). These characteristics imply that enzyme B13 and S6 may be a promising enzyme for meat tenderization at low temperatures. Furthermore, the use of collagenolytic proteases as a meat tenderizing enzyme in goat meat has not yet been reported. An ideal meat tenderizing enzyme should degrade collagen and have a slight effect on myofibrillar protein. The potential of tenderizing goat meat which possesses large amounts of connective tissue means that the reduction of toughness should be elucidated. Therefore, this research aimed to evaluate the effect of collagenolytic proteases from B. subtilis B13 and B. siamensis S6 on tenderization and goat meat quality during 21 days of wet aging.
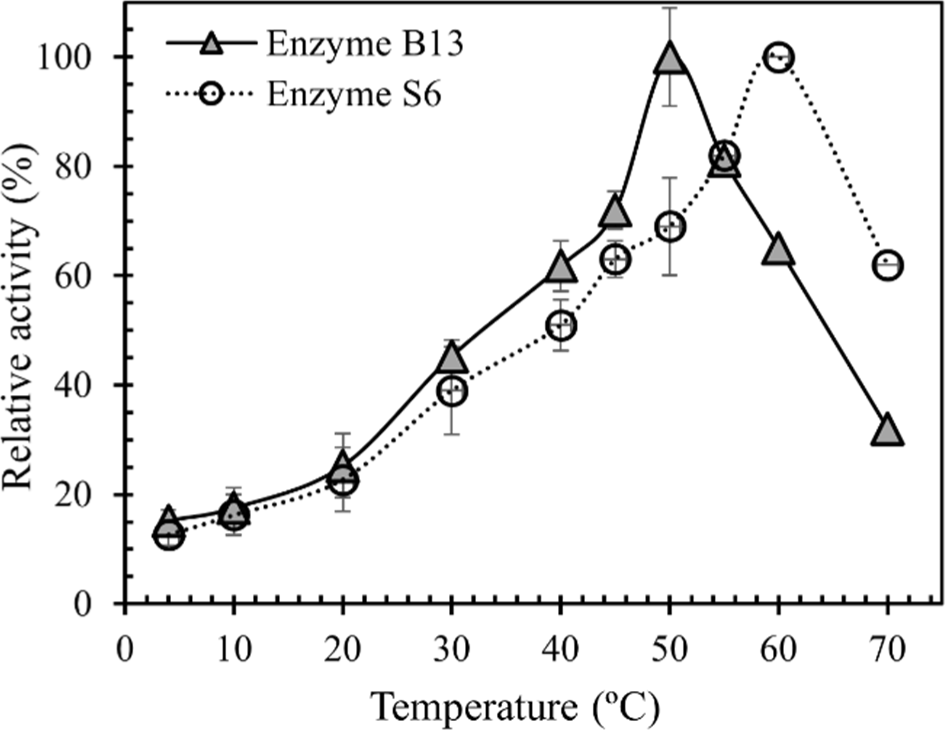
Materials and Methods
Two collagenolytic proteases were purified from B. subtilis B13 and B. siamensis S6 using a process previously described by Sorapukdee et al. (2020), then lyophilized, and stored at 20°C until use. These enzyme solutions were dissolved in distilled water and collagenolytic activity was determined using collagen from bovine Achilles tendon (C9879, Sigma-Aldrich, St. Louis, MO, USA) as a substrate based on the method described by Sorapukdee et al. (2020). Prior to use of these enzymes, the preliminary test by varying enzyme concentrations (0, 2.5, 5 and 10 U/mL) was prepared and injected into meat with 10% (v/w) of enzyme solution. Thereafter, the injected meats were vacuum packaged in plastic bags at 4°C for 5 days. The results showed that both 5 and 10 U/mL of enzyme had the lowest shear force than 0 and 2.5 U/mL (Fig. 2). Therefore, the final concentration of 5 U/mL of collagenolytic activity from B13 and S6 was assigned for this study.
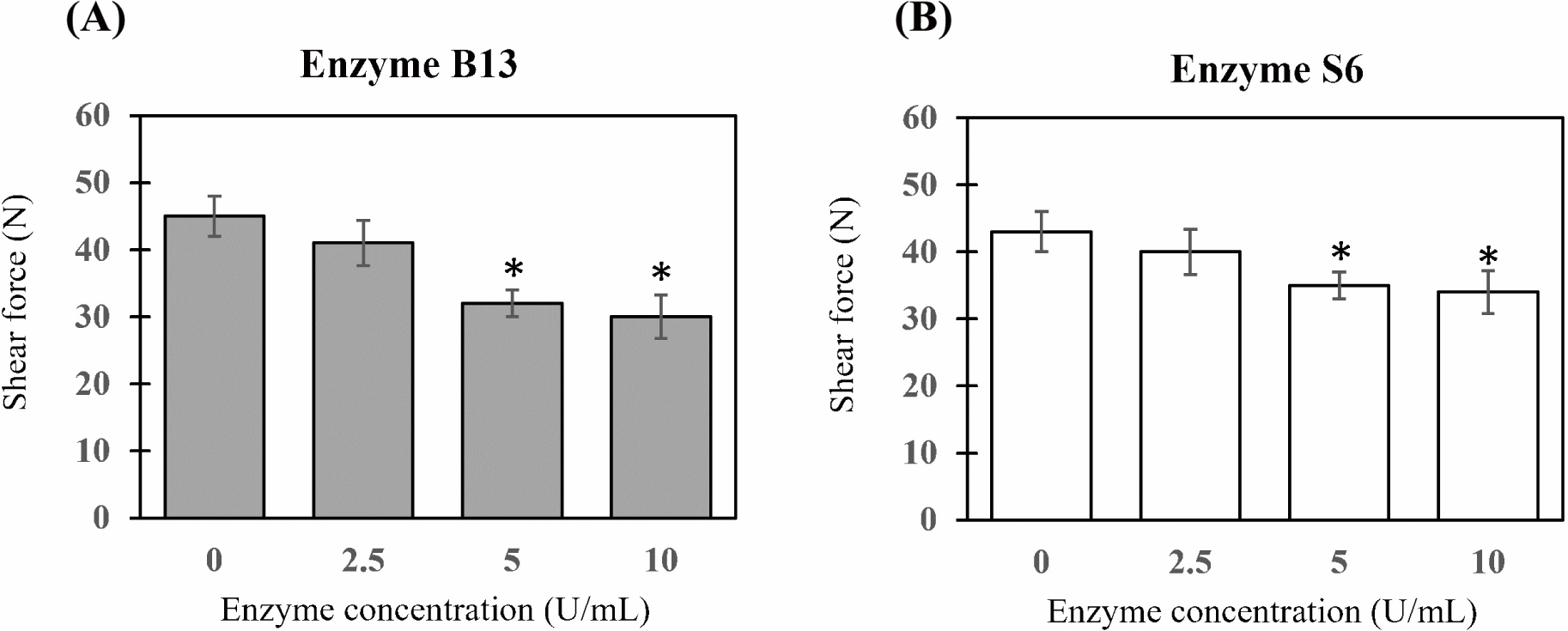
Goat meats were fabricated from the hind leg muscles of a goat after slaughter, which were purchased from a local market, cut to approximately 5.0 cm×2.5 cm×7.5 cm (height×width×length), and then stored for 1 day at 4°C. The meat was divided into 3 groups for treatments: control, collagenolytic protease B13, and S6. To inject the enzyme into the intercellular spaces of meat, each sample was injected with the 10% (v/w) of enzyme solution (based on the weight of the meat) using a syringe. For the control group, the meat was injected with distilled water with the same volume as the enzyme-treated samples. All samples were aged for 21 days at 4°C after being vacuum-packaged in plastic bags. On days 0, 3, 5, 7, 14, and 21, the meats were sampled to monitor changes in microbiological, meat textural, and physicochemical qualities. The experiment was evaluated in triplicate for all test samples (n=3).
The total aerobic count (TAC) of the samples was determined according to the technique of AOAC (2012). A sample (25 g) of meat was blended with 225 mL of sterile saline solution (0.85% NaCl). The samples were homogenized using a stomacher for 1 min at room temperature. For enumerating microbes, 1 mL serial dilutions (1:10 diluent and sterile saline solution) of meat homogenates were mixed in culture for enumerations of TAC in Plate Count Agar (Merck, Darmstadt, Germany). Then, the agar plate for TAC was incubated at 37°C for 48 h. The number of colonies was counted and shown as Log CFU/g.
Cooked meats from cooking loss determination (as stated below) were used to evaluate shear force values. Five rectangular samples for each treatment (1 cm×1 cm×2.5 cm) were taken. Each sample was sheared perpendicular to the myofibrillar direction using an Instron universal testing machine (Instron, Norwood, MA, USA). Shear force values were expressed in newtons (N).
Texture profile analysis (TPA) value was assessed in cooked meats using an Instron universal testing machine with a compression plate surface. The meat samples were cut into five cube samples (2 cm×2 cm×2 cm) and placed on the instrument’s base. The TPA textural parameters were evaluated with the following testing conditions: cross-head speed was 60 mm/min and compressed twice to 40% of their original high. Data were collected and processed by using the Bluehill 2 software (Instron). The force-time curves generated for each sample were calculated for TPA parameters.
Ground samples (1.5 g) were homogenized with 13.5 mL of 5% (w/v) trichloroacetic acid (TCA) using a homogenizer. The homogenate was kept on ice for 30 min, and centrifuged at 5,000×g for 20 min. The supernatant of soluble peptides was evaluated according to the procedure of Lowry et al. (1951). The standard of tyrosine was used, and values were expressed as μmol tyrosine/g sample.
Thiobarbituric acid reactive substances (TBARS) values in extracts from examined meat samples were used to estimate the lipid oxidation of products. According to the practice of Buege and Aust (1978), samples (2.5 g) were disseminated in 12.5 mL of thiobarbituric acid solution, 0.0375% (w/v) TBA, 15% (w/v) TCA, and 0.25 N HCl. The mixture was homogenized for 1 min and heated in a laboratory water bath at 100°C for 10 min, cooled, and centrifuged at 3,600×g for 20 min. The absorbance of the supernatant was read at 532 nm. The TBARS values were computed from a standard curve of 1,3,3,3-tetra-ethoxypropane and shown as mg MDA/kg sample.
The samples were weighed and boiled in a laboratory water bath until reaching 71°C for the core temperature, detected by a digital thermometer (Fluke, Everett, WA, USA). Then, the samples were cooled to room temperature for 30 min and weighed. Cooking loss was calculated with the following formula:
The effects of enzyme-treatment and aging time as well as interaction were assessed for statistical significance (p<0.05) using the GLM procedure of SAS Version 9.1. Significantly different means were then identified using Duncan’s multivariate range test. The least square means were reported for significant main effects and interaction.
Results and Discussion
The numbers of TAC in goat meats from the control and various enzyme-treated samples during aging are presented in Fig. 3. Generally, aged meat would be unacceptable or spoiled at bacterial counts lower than 7 Log CFU/g (Dainty and Mackey, 1992). Regarding the effect of aging time, all samples showed an increase in TAC value when aging time increased (p<0.05). These counts started from 3.20 Log CFU/g on the initial day to an acceptable value of 6.47 Log CFU/g on day 14. However, on day 21, the TAC values of all samples were 7.46, indicating unacceptable meats. The levels of bacterial counts throughout the 14 days of storage in the present study were consistent with Ali et al. (2021) and Sabow et al. (2016), who reported these values in wet-aged goat meat. For the effect of enzymes, B13- and S6-treated samples showed a higher TAC value than the control (p<0.05). However, the bacterial population in B13- and S6-treated samples aged for 5 days were safe (5.28 and 5.25 Log CFU/g, respectively) according to the Agricultural Commodities and Food Standards for goat meat production (Thai Agricultural Commodity and Food Standard, 2006), which stated that up to 5.7 Log CFU/g is acceptable for consumers. Meanwhile, TAC in the control sample aged for 7 days (5.16 Log CFU/g) remained lower than the regulation guidelines. The addition of microbial enzymes in aged meat could cleave peptide bonds and disintegrate muscle protein structures. This evidence was considered to promote substrates for spoilage bacteria growth, which decreased the shelf life of the enzyme-treated group.
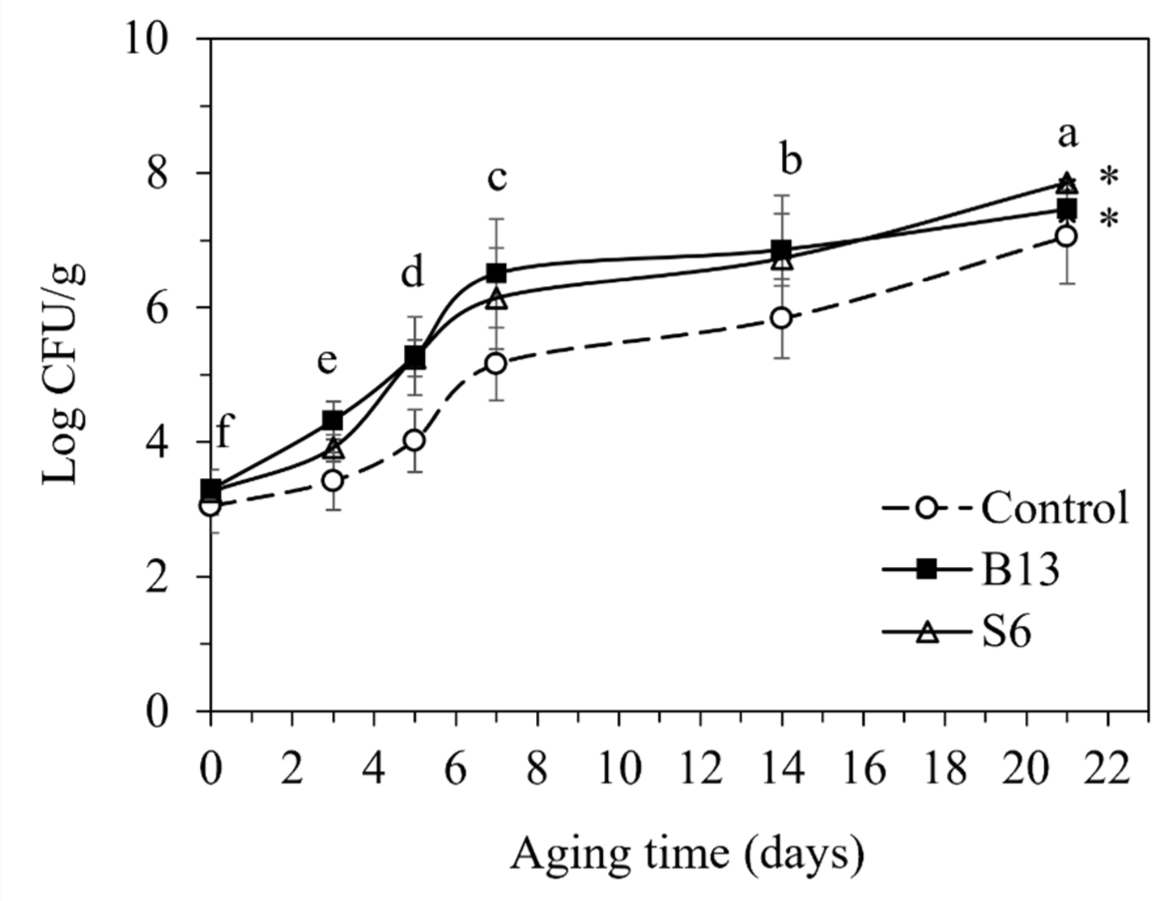
Tenderness plays an important role in the quality of meat, and is one of the most significant attributes of consumer acceptance. Comparing three treatment samples, the Warner-Bratzler shear force (WBSF) values were significantly lower in B13- and S6-treated samples than in the control samples (p<0.05; Fig. 4). As aforementioned in the TAC part, both enzyme-treated samples aged for 5 days at 4°C had an acceptable microbial quality. This condition produced meats with 30% and 26% reductions in WBSF for B13 and S6, respectively, as compared to the control. The B13- and S6-treated meats aged for 5 days also had similar WBSF values (31.42 and 33.41 N, respectively) as compared to the control aged for 21 days (32.53 N). Naveena and Mendiratta (2004) revealed that buffalo meat treated with proteolytic enzymes had reduced shear force values compared to the control. Aging time could improve tenderness as described by the reduction in WBSF in all treatments (p<0.05). The highest WBSF value was found on day 0 at 45.74 N, but was then dramatically reduced to 34.93 N on day 7, and showed the lowest value of about 31.39–30.41 N on days 14 and 21 (p<0.05). Our results agreed with Duckett et al. (1998) who stated that the shear force values of lamb loin chops aged for 24 days decreased with aging time, with the maximum reduction in shear force value occurring from day 1 to day 12. Abdullah and Qudsieh (2009) revealed that aging meat from lambs for 7 days reduced the force from 28.3 N on day 1 to 20.7 N on day 7. Without adding exogenous proteases, the decrease in the WBSF value of aged meat is normally caused by endogenous proteases (mainly from calpains) that can cleave the myofibrillar structure. During aging, Ca2+ accumulation in sarcoplasm muscle leads to the stimulation of μ-calpain, which in turn causes loss of the intact myofibrillar structure by degrading myofibrillar proteins involving titin, filamin, troponin-T, and desmin (Lomiwes et al., 2014).
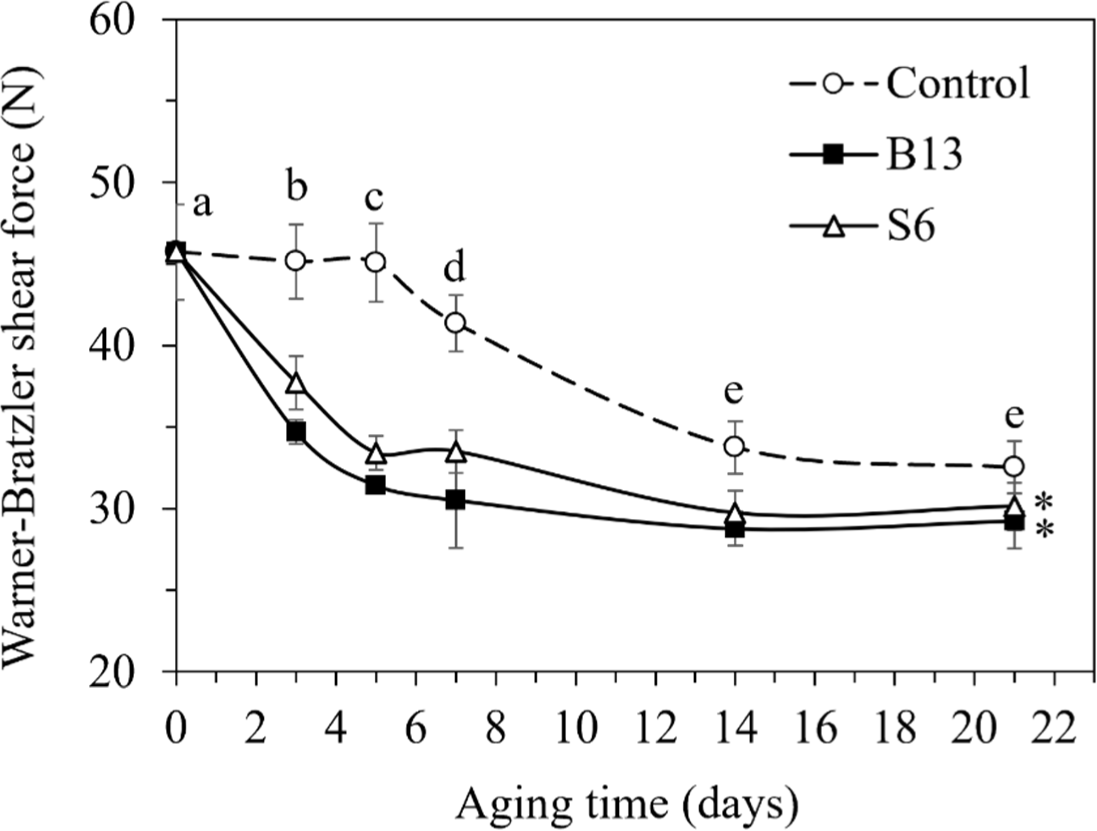
Parameters for TPA consist of hardness, cohesiveness, gumminess, springiness, and chewiness, which are useful to predict the texture of cooked meat. In the present study, the effect of collagenolytic proteases on TPA in goat meat during aging is shown in Table 1. All samples showed the textural changes during aging in terms of a decrease in hardness, gumminess, and chewiness with an increase in springiness, especially during the first 7 days of aging (p<0.05). Meanwhile, the cohesiveness of all treatments did not change significantly during the 21 days of aging (p>0.05). For the effect of enzyme-treated samples, meat samples had lower hardness, gumminess, and chewiness, but higher springiness in B13- and S6- treatments compared with the control (p<0.05). Again, there were no significant differences in cohesiveness among treatments (p>0.05). When considering WBSF combined with TPA in terms of hardness, gumminess, and chewiness, it was found that enzyme-treated samples of both B13 and S6 were more tender than the control. Qihe et al. (2006) also reported that beef meat treated with elastase from Bacillus sp. EL31410 had lower hardness during 100 hours of storage than the control.
The extent of proteolysis among treatments during the aging time of goat meat was also determined by TCA-soluble peptides. The number of soluble peptides significantly increased over aging time (p<0.05). It was found that these peptides increased from 1.55–1.78 μmol tyrosine/g sample at the beginning (day 0 to day 3) to 3.96 to 4.18 μmol tyrosine/g sample at the end of aging (day 14 to day 21; Table 2). The endogenous proteases in meat like μ-calpain and cathepsin could degrade myofibrillar and sarcoplasmic proteins together with the action of added bacterial enzyme decomposing oligopeptides into small peptides and free amino acids. Specifically, samples treated with B13 and S6 had higher TCA-soluble peptides than the control (p<0.05). It was clear that collagenolytic proteases from B13 and S6 had the potential to be meat tenderizers which still showed hydrolytic properties during aging at 4°C and produced a softer meat texture with lower values of WBSF and hardness. In our previous study, these two collagenolytic proteases preferred to degrade connective tissue protein (both collagen and elastin) rather than myofibrillar protein. In any case, B13 had strong activity for selectively cleaving intramuscular collagen, whereas S6 greatly hydrolyzed elastin (Sorapukdee et al., 2020).
Lipid oxidation in meat is a very significant factor because it can cause the deterioration of quality in fresh meat, especially in color, flavor, texture, and nutritive value (Kim et al., 2018). Table 2 shows the changes in lipid oxidation as indicated by TBARS values in goat meat during aging. Differences between exogenous protease-treated samples and the control on lipid oxidation were not found (p>0.05). However, lipid oxidation increased with aging time (p<0.05). The levels of lipid oxidation gradually increased during the first 5 days of aging, then dramatically rose during days 7 to 14, before remaining constant after days 14 to 21 (p<0.05). At the end of the aging time, lipid oxidation reached about 2.07 to 2.18 mg MDA/kg sample. The criterion value of TBARS of approximately 5 mg MDA/kg sample is used to identify a detectable unusual flavor development in meat (Insausti et al., 2001), which was not reached in the present research. Chemically unstable fats, especially polyunsaturated fatty acids, are susceptible to oxidation during aging. Lipid oxidation results from free radical generation leading to the production of malondialdehyde or/and other oxidation products (Falowo et al., 2014; Morrissey et al., 1998). This finding concurs with the previous report stating that refrigerated storage had a significant impact on lipid oxidation (Adeyemi et al., 2016; Kim et al., 2018).
Cooking loss is a quality term to refer to the water-holding capacity (WHC) of meat during heating, which is necessary for both the industry and consumers. Table 2 shows the cooking loss of goat meat during aging. Collagenolytic protease B13 and S6 treatments had a lower cooking loss than the control (p<0.05). In addition, the highest cooking loss in all samples was found in the first 3 days of aging, followed by day 7 and days 14–21, respectively (p<0.05), which exhibited lower cooking loss or higher WHC when the aging time increased. These results were consistent with the research of Kristensen and Purslow (2001) who described the WHC of meat decreasing during the first 2 to 7 days post-mortem, and finally increasing during aging. Similar outcomes have been published by Kannan et al. (2006) stating that goats had lower cooking loss on days 4, 8, and 12 than at the beginning of storage. The formation of a ‘sponge effect’ due to muscle structural breakdown leads to the disruption of channels for water loss, resulting in the improvement of WHC with long-term meat aging (Farouk et al., 2012; Huff-Lonergan and Lonergan, 2005), as well as collagenolytic protease-treated meat.
The meat color depends upon various factors and their interactions. Goat meat has revealed lower intramuscular fat on goat carcasses, resulting in lower CIE L* and higher CIE a* than lamb (Babiker et al., 1990). Table 3 shows the color measurements of goat meat with collagenolytic protease treatment during aging. The collagenolytic protease-treated samples (B13 and S6) exhibited lower CIE L* (p<0.05) than the control, while CIE a* and CIE b* had no significant differences among treatments (p>0.05). Moreover, all treatments showed a similar profile of color changes, which decreased in CIE L* and CIE a* with an increase in CIE b* during aging (p<0.05). CIE L* decreases might be related to the sponge effect and the change in the WHC of the meat. Collagenolytic protease-treated samples and prolonged aging allowed the condition for protein degradation and muscle structure disintegration, resulting in greater water retention in the structure. The lower amount of water loss in meat refers to greater myoglobin presence within the meat structure. In addition, a decrease in water loss on the surface of the meat causes the light to reflect less. This might be the reason why enzyme-treated meat and a longer aging time showed lower CIE L*. A decrease in CIE a* can be associated with myoglobin oxidation due to the loss of metmyoglobin reducing activity (MRA) that led to an accumulation of metmyoglobin in the meat during aging (Xue et al., 2012). Seydim et al. (2006) stated that the oxidation of myoglobin affects the reduction of CIE a*. Regarding CIE b*, Karami et al. (2011) also showed that the CIE b* of Kacang goat meat was significantly increased by aging time, which was related to an increase in lipid oxidation.
Conclusion
The collagenolytic proteases could be applied to produce more tender wet-aged goat meat as compared with the control. Both B13- and S6-treated meat aged for 5 days at 4°C were shown to improve the tenderness of goat meat to be as tender as the control aged for 21 days, without adversely affecting meat quality as specified by microbiological quality, lipid oxidation, WHC, and color. Therefore, the application of collagenolytic proteases from these Bacillus strains could reduce the aging time and improve the quality of goat meat, in terms of tenderness.