Introduction
Korea had the highest meat consumption in Asia at approximately 54 kg per capita in 2018. This amount is expected to increase in the future (Kim et al., 2019). Accordingly, the slaughter industry is predicted to develop in line with increasing meat consumption. In addition to meat consumed as a commodity, slaughter processing generates a large number of by-products, including various organs, blood, and bones. In Korea, 0.867 million cows, 17.370 million pigs, and one billion chickens are slaughtered every year as of 2018. This means that the number of by-products estimated could reach 0.355, 0.955, and 1.306 million tons of cattle, pigs, and chickens, respectively (Kim et al., 2019). The amount of meat by-products is anticipated to increase as meat consumption increases per year.
Meat by-products, including pork intestines, are better sources of nutrients, such as essential amino acids, minerals, and vitamins (Toldrá et al., 2012). Thus, they are consumed as healthy foods, as they are easy to be digested and absorbed (KFRI, 2012). Moreover, it has been generally reported that intestines are good sources of collagen, which is known for its beauty benefits, such as skin elasticity, which could be the reason for customers’ preference for pork large intestines (Jeon, 2013; Jeon and Kim, 2013; Kim, 2009). In general, pork large intestine belongs to intestine with high preference (Jeon and Kim, 2013). In contrast, many consumers dislike or refuse the large intestine owing to its characteristic off-odor, perhaps caused by excreta passing through it (KFRI, 2012).
Washing the intestines under running tap water with flour or salt has been used to eliminate the off-odor but does not completely solve the problems. Sometimes, some producers use laundry detergents or chemicals that are harmful to the human body to remove off-odors (Kim, 2013). Cooking offers some advantages as it can maximize sterilization effects, prolong shelf-life, and decrease off-odors in the pork intestine manufacturing process (KFRI, 2012).
In Korea, practically no data are available regarding off-odor reduction according to cooking time of pork intestines, including the large intestine. At present, in commercial practice, pork large intestines are produced through a cooking time of 40–50 min, but it is not enough to reduce overall off-odor, resulting in consumer rejection (KFRI, 2012). Therefore, the purpose of this study was to determine the optimal cooking time for cooking loss, shear force, and off-odor reduction of pork large intestines.
Materials and Methods
The frozen pork large intestines were purchased from a local market in Seoul, Korea, and thawed for 24 h at 4°C. Then, the samples were washed roughly under running tap water to remove only adhered feed remnants and feces. After draining the water, the samples were quartered perpendicularly and weighed. Each sample of the similar weight (approximately 900 g) were collected and cooked in a pot with 3.5 L of tap water at 100±1°C for 40, 120, 180, and 240 min. After cooking for the designated times, the samples were removed from the pot and kept at room temperature for 10 min to cool down. The samples were placed in vacuum-packaging bags and refrigerated at 4°C for further analysis.
The vacuum-packed large intestine samples were boiled in a water bath until the core temperature reached 72°C. The cooking loss of the pork large intestines (weight 100±5 g) was defined as a weight percentage before and after cooking according to the following equation:
After the cooking process, texture of the cooked samples was measured by Warner-Bratzler shear force (WBSF) analysis using a texture analyzer (TA1, Lloyd Instruments, Fareham, UK) with a cell load of 0.1 N and a crosshead speed of 200 mm/min (Lee et al., 2021). From each cooked block, the cooked samples (120 mm×40 mm; length×diameter) were cut perpendicularly to the lumen of the large intestine and placed under a Warner-Bratzler shear probe transversely to the lumen. Three samples were obtained and analyzed in triplicate.
The volatile compounds produced according to cooking were analyzed using an electronic nose (Heracles II, Alpha MOS, Toulouse, France; Lee et al., 2019). The samples were ground using a meat grinder (MG510, Kenwood, Hampshire, UK), and pooled from 3 replicates. The pooled sample (5±0.005 g) was weighed in a 20 mL vial and cooked for 10 min at 80°C to obtain volatile compounds. Then, the volatiles were injected into an electronic nose equipped with dual columns of MXT-5 and MXT-1701 (10 m×180 m×0.4 m; length×diameter×thickness; Restek, Bellefonte, PA, USA). Hydrogen was used as the carrier gas at a flow rate of 120 μL/s. The following temperature conditions were used: Injector at 200°C; temperature program including isotherm at 45°C for 5 s, 0.5°C/s ramp to 150°C, isotherm at 150°C for 5 s, 5°C/s ramp to 260°C, and isotherm at 260°C for 30 s. Each peak was integrated and identified using a retention time and relevance index indicating the matching probability percentage, based on the comparison of the Kovats retention index of the detected compound and the Kovats retention indices of known compounds from the AroChemBase library (Alpha MOS).
All experiments were performed in triplicate with 3 observations. Statistical analysis for the pork large intestines with different cooking times was performed using one-way analysis of variance (ANOVA), and significant differences were identified using the Tukey’s multiple range test in the SAS statistical software program (SAS, Release 9.4; SAS Institute, Cary, NC, USA; p<0.05). Results are reported as mean values with a SEM. Principal component analysis (PCA) was performed using MetaboAnalyst 4.0 (www.metaboanalyst.ca) in accordance with Kim et al. (2020), to verify the difference in volatile organic compound (VOC) composition among the treatment samples.
Results and Discussion
Cooking loss and shear force values of pork large intestine were affected by different cooking times (Table 1). When the samples were cooked for 240 min, the cooking loss was higher than at other cooking times. However, there was no difference between the 40, 120, and 180 min cooking times (p<0.05). Increasing the cooking time resulted in a lower trend in shear force values (Table 1). A 240 min cooking time induced a lower shear force value than other cooking times (p<0.001).
Traits | Cooking time (min) | SEM | |||
---|---|---|---|---|---|
40 | 120 | 180 | 240 | ||
Cooking loss (%) | 7.96b | 7.88b | 8.03b | 10.92a | 0.559 |
Shear force (N) | 19.56a | 10.94a | 14.75a | 4.45b | 2.776 |
Natural intestines contain large amounts of collagen (Jeon, 2013). Before cooking, collagen has a quasi-crystalline structure with a high elastic modulus. When collagen is cooked between 59°C–65°C, collagen molecules deform from the crystalline state to an amorphous structure (Lepetit, 2008). This means that gelatin is generated through partial thermal hydrolysis of the collagen polypeptide chain and cross-linkage (Hashim et al., 2015). The gelatin could be evenly dispersed in the space of collagen fibers, filling the gap among the collagen fibers as a filler with interaction. Agban et al. (2016) mentioned that mutually strong interaction by the addition of nanofiller in collagen induced significantly increased tensile strength for collagen material. However, heating energy over a certain level could create the higher weight loss and lower toughness. When the cooking temperature reaches 280°C–370°C for 9 min, collagen and gelatin polymeric chains thermally degrade with weight loss in the collagen/gelatin composite film (Xiao et al., 2021). From previous studies, it could be assumed that the increased cooking loss in this study during the 240 min cooking time was due to collagen and gelatin polymeric chain degradation. The thermal energy may have been sufficient to cut the chemical bonds and degrade the polymeric chains due to the longer cooking times or higher temperatures. Thus, the cut-polymeric chains could also have had a low shear force. KFRI (2012) reported that cooking (around 65°C–77°C of core temperature) tenderized connective tissue through the conversion of collagen to gelatin and cooking time played a crucial role in tenderizing connective tissues. This report showed decreasing hardness values with increasing cooking time.
Eating quality attributes including tenderness, flavor, and juiciness can affect the purchasing decisions of consumers (Troy and Kerry, 2010). Among the eating quality traits, tenderness is the most important property for meat (Destefanis et al., 2008). Kim (2013) mentioned that trained panelists positively evaluated chewy texture in intestine-related sensory evaluation (1 point, least chewy; 5 point, most chewy). In addition, Warner-Bratzler shear force was positively correlated with the sensory evaluation variables directly, especially chewiness (Choe et al., 2016; Oh et al., 2019). Thus, we expect that a 240 min cooking time may negatively affect pork large intestine texture owing to the low shear force values but it should be confirmed by a sensory analysis
Table 2 presents the VOCs for pork large intestines cooked at different times. Ten VOCs, including aldehydes, esters, and alcohols, were detected in the pork large intestines. The amount of methyl pentanoate and methyl isobutyrate decreased as cooking time increased (p<0.05, Table 2). The pork large intestine is an aisle for excretion, generating the characteristic off-odor (KFRI, 2012). In terms of fecal odor, volatile fatty acids (VFAs) with long carbon chains (butyric acid, valeric acid, caproic acid, and caprylic acid) and branched chains (iso-butyric acid, iso-valeric acid, iso-caproic acid, and iso-caprylic acid) had a higher correlation with pork fecal odor intensity than short carbon chains (formic acid, acetic acid). Thus, VFAs with long carbon chains and branched chains were expected to be used as odor indicators (Zhu et al., 1999). Overall, methyl pentanoate and methyl isobutyrate could be transformed by pentanoate and isobutyrate, which can be used as fecal odor indicators via methyl esterification. Heat is required to form fatty acid methyl esters (FAMEs). FAMEs are typically generated by a base-catalyzed reaction between fatty acids and methanol (Yu et al., 2010). Feces generally have a pH 8–12 (NSA, 2010), which might affect the pH of the large intestine. Kim et al. (2019) reported that heat from boiling and large intestine alkaline conditions induced methyl esterification. In this study, it was assumed that the amount of VFAs, which act as substrates for the esterification reaction, did not change after cooking for longer than 120 min. In addition, it was expected that methanol was a residue of post-methyl esterification.
Compound | RI1) | Cooking time (min) | SEM | Aroma description | Odor threshold (ppm) | References | |||
---|---|---|---|---|---|---|---|---|---|
40 | 120 | 180 | 240 | ||||||
Propenal | 82.91 | 100,844 | 55,455.89 | 80,005.78 | 163,801.27 | 46,970.212 | Pungent | 0.0036 | Chemical book (2016) |
Methyl formate | 75.00 | 88,311.06 | 82,097.86 | 68,926.26 | 107,400.01 | 36,676.450 | Pleasant | 130 | Chemical book (2016) |
Methyl pentanoate | 89.00 | 34,038.25a | 17,528.71ab | 23,371.84ab | 5,163.85b | 6,079.401 | Fruity | 0.0022 | Chemical book (2016) |
Ethene, 1,2-dichloro, [E]- | 88.20 | 18,552 | 12,936 | 5,115.73 | 2,937.31 | 8,421.834 | Pleasant | 17 | Chemical book (2016) |
2-Pentanol | 92.43 | 11,609.95a | 3,785.65b | 5,370.25b | 1,501.59b | 1,047.442 | Alcoholic | 0.29 | Chemical book (2016) |
Methanol | 77.37 | 9,096.96a | 5,245.72ab | 6,332.87ab | 4,621.71b | 945.892 | Pungent | 100 | NCBI (2019) |
Dichloromethane | 76.55 | 7,587.68 | 5,514.41 | 2,669.88 | 1,590.76 | 2,008.755 | Sweet, pleasant | 23.4 | NCBI (2019) |
Bromochloromethane | 88.28 | 3,046.95a | 2,543.68ab | 2,004.01ab | 1,124.84b | 389.723 | Sweet chloroform-like | 2100 | Ruth (1986) |
Methyl isobutyrate | 80.59 | 1,955.38a | 812.51b | 619.02b | 342.76b | 107.376 | Fruity | 0.0019 | Nagata and Takeuchi (2003) |
1-Propanol | 61.50 | 1,476.33a | 622.26b | 490.33b | 372.70b | 91.29 | Alcoholic | 0.094 | Chemical book (2016) |
Propenal had the highest concentrations, with a low odor threshold value among all VOCs. Propenal acts as an intermediate agent in the synthesis of DL-methionine, an essential amino acid, glycerin, glutaraldehyde, and other organic compounds (De Woskin, 2003). This compound could also be detected in the feces (HMDB, 2021). In addition, propenal accelerates the cross-linking of protein collagen (De Woskin, 2003). Thus, this compound contributed to the toughness of the large intestine.
Furthermore, the amounts of 2-pentanol and 1-propanol declined significantly after cooking for longer than 120 min. The VOCs in the pork large intestine are highly influenced by fat because of the high composition ratio of fat (19.54%) in the large intestine (Seong et al., 2014). Alcohols, such as 1-propanol and 2-pentanol, are mainly generated by lipid oxidation (Górska-Horczyczak et al., 2017; Lorenzo et al., 2013). When meat is cooked, lipids are liquefied and leak out (Yang et al., 2009). It was supposed that increased cooking time induced lipid leakage from the large intestine and decreased the amount of oxidizable lipids. Eventually, 2-pentanol and 1-propanol declined at a 120 min cooking time owing to lipid leakage, but no difference was found after cooking for longer than 120 min. Methyl formate, methanol, and bromochloromethane might contribute less to off-odors because of the high odor detection threshold.
PCA is a widely used chemometric method for visualizing multidimensional data, modeling, and compression to check for obtained data visualization (Wiśniewska et al., 2016). Fig. 1 shows 10 analyzed principal components where the first two principal components are considered significant. It illustrates the PCA score plot (A) and the loading plot (B) with the volatile compounds in pork large intestines. In the score plot, PC1 and PC2 showed 70% of the data variance (PC1=53.9%, PC2=16.1%). There was a clear difference in the relative positions of the samples according to cooking time.
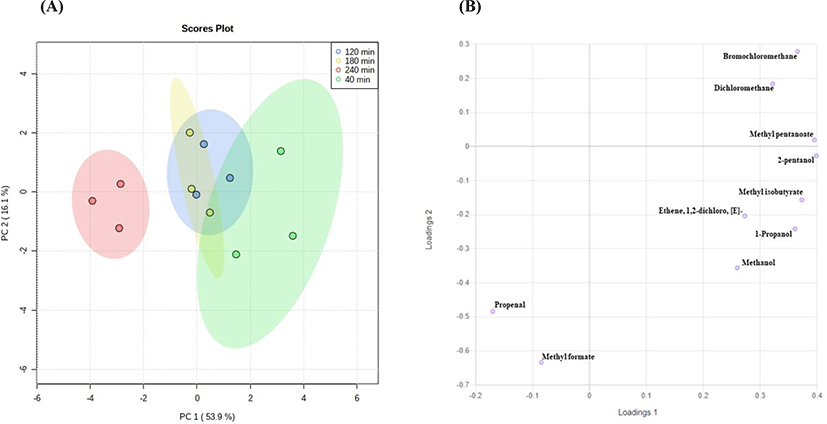
The first component (PC1) in Fig. 1A explains the left-oriented pattern based on the increase in cooking time. In particular, the results observed after the 40 min cooking time was different among the three overlapping groups, except for the 240 min group. A 240 min cooking time was placed on the left part of the PC1 and far from other cooking times. Fig. 1B shows the loading plot, suggesting group-differentiating compound concentrations. As Fig. 1B shows, the separation at a 40 min cooking time was highly affected by some volatile compounds such as bromochloromethane, dichloromethane, 2-pentanol, methyl isobutyrate, 1-propanol, and methanol. Given the low odor threshold values (Table 2), the changes in 1-propanol, 2-pentanol, and methyl isobutyrate concentrations might be key for the separation characteristics of the samples cooked for 40 min. Moreover, propenal and methyl formate on the loading plot may be relevant to the characteristic volatile pattern, where 240 min was separated. However, these compounds were not significant for the 240 min separation due to an insignificant distinction of amounts with increase in cooking time and the high odor threshold relative to methyl formate. Only a 40 min cooking time showed a practically separated pattern, suggesting that a 120 min cooking time could be the optimum condition.
Conclusion
This study aimed to investigate the best cooking time in terms of cooking loss, shear force, and off-odor reduction for pork large intestines. Cooking pork large intestines for 120 min may be optimal to induce positive quality outcomes and off-odor reduction. Cooking pork large intestines for 240 min yielded a higher cooking loss and might affect the texture negatively. When it comes to the amount of methyl ester, such as methyl pentanoate and methyl isobutyrate, and lipid oxidation-related compounds (2-pentanol and 1-propanol), no change was found for cooking times longer than 120 min. Cooking the intestines for 120 min is quite economical because it had similar consequences as the 180 min cooking time within a shorter period. The findings of the present study may help promote the consumption of pork intestines. Furthermore, future studies on sensory evaluation would be helpful to convince this data more.