Introduction
Eggs are an essential component of the human diet and are widely consumed around the world. Eggs are utilised in a variety of ways, including breakfast, home meal preparation, baking, and as ingredients in a variety of meals. In addition, eggs are considered a nutrition powerhouse (Wahba et al., 2014). Eggs are a vital source of critical nutrients since they are well-balanced and high in fatty acids, iron, phosphorus, trace minerals, vitamins A, B6, B12, D, E, and K, as well as high biological value proteins (Stadelman et al., 2017). More recently, research has shown that eggs supply significant amounts of carotenoids, possibly playing a role in disease prevention. The nutritional composition of eggs makes a valuable contribution to the overall nutritional balance of the diet. It is a very economical source of protein, vitamins, and minerals that are very important for elderly people, infants, and children and is the most suitable food for low-income families to fulfill their nutrient requirements (Applegate, 2000).
Eggs continue to be a well-liked food in every nation, and the egg industry is a significant component of the global food economy. Egg-based products are equally important because they provide additional nutrients and tastes (Mahfuz et al., 2018; Maslova et al., 2020; Swanson et al., 2011). Several preservation methods extend the shelf life during egg product processing. Egg products come in a variety of forms, including liquid, frozen, fermented, and dried. Peeled hard-boiled eggs and other speciality goods are made for both commercial and retail use (Cotterill, 1995). The hard-boiling, cooling, and peeling of eggs for the simplest ready-to-eat egg products are distributed to retail markets, the food service industry, and food manufacturers (Stadelman et al, 2017). Currently, most restaurants and other food-related industries prefer peeled hard-boiled eggs over raw eggs because hard-boiled eggs have homogenous quality and appearance. Furthermore, these eggs are easy to use and free from fecal-contaminated shells, increasing consumer food safety (Miranda et al., 2015). In addition, most microorganisms, including Salmonella species, are destroyed at high temperatures. This is an added advantage in terms of shelf life (Stadelman et al., 1982).
The egg industry frequently uses traditional cooling techniques to cool hard-boiled eggs, such as air blasts and slow air (Caro-Corrales et al., 2002). Cooling is mainly performed to reduce the temperature of the eggs to prevent microbial growth and preserve the eggs. These rapid cooling techniques increase food safety and enhance product quality by minimizing the loss of nutrients (Erdoğdu et al., 2005). However, refrigeration and cold storage techniques are the most common methods used in the egg production industry. Cold storage temperatures of less than 8°C inhibit the growth of most microorganisms and reduce the loss of internal quality (Humphrey, 1994). Refrigeration slows the chemical and biological processes in foods and accompanies deterioration and loss of quality. In addition, it is more effective at preserving egg quality than surface coating (Nongtaodum et al., 2013).
Since eggs are a great culture medium for microorganisms, keeping the color, taste, scent, and texture of boiled eggs under refrigeration is a challenge that many restaurants and other sectors must overcome (de Souza et al., 2019). During cold storage, egg whites become rubbery, granular, watery, and separate into small crumbs or layers (Woodroof, 1942). The mechanical actions of the generated ice crystals appear to be the origin of the freezing-induced damage to boiled egg white. The production of small crystals can be encouraged by techniques like super-cooling or the addition of calcium carbonate fine granules, which can lessen the damage. The pH of the egg white, boiling time, temperature, freezing method, and additives influence the quality of frozen-boiled eggs (Davis et al., 1952). However, these methods are not used because of their high production costs. Storing at room temperature for a long time is also not possible because of microbial spoilage. It is important to meet the consumer demand for high quality and physical appearance (Jones and Musgrove, 2005). Most customers are misled by synthetic eggs and hesitant to eat them because cold storage of cooked eggs causes textural changes that make them unpleasant to eat. Additionally, eggs stored at room temperature can cause food poisoning. Therefore, it is important to find a suitable method for storing hard-boiled eggs without deteriorating their physical, chemical, and microbiological properties at the industrial level. Eggs may show early signs of deterioration or quality changes within the first few days of storage, therefore a 48 h period could offer useful information on how different storage temperatures affect the physicochemical qualities of the eggs early in the storage process. Therefore, the objective of this study was to determine the structural and physicochemical changes in hard-boiled eggs stored at different temperatures (25°C, 4°C, and –18°C) for up to 48 h.
Materials and Methods
Ninety-three unwashed, clean, brown-shell, medium-sized (45–55 g) eggs were obtained from the local market and stored for three days after collecting from the farm. Eggs were boiled at 95°C–100°C for 15 min. The boiled eggs were stored at three different temperatures, i.e., room temperature (25°C), refrigerated (4°C), and frozen (–18°C), for 0, 12, 24, 36, and 48 h, and analyzed for physical, structural, and chemical changes.
The textural profile analysis of hard-boiled eggs was calculated based on the Peleg (2019) with a few modifications. Boiled eggs stored either frozen or refrigerated were thawed before analyzing the texture profile in a 10 mm height and 10 mm width manner using a cork borer (Korea Ace, Seoul, Korea) at a distance of 15 mm from the probe attached to a cylindrical aluminium probe and equipped with a texture profile analyzer blade attachment (TA-XT2 2i, Stable Micro System, Godalming, UK). The texture profile analysis (TPA) parameters, such as hardness (kg·f), cohesiveness (ratio), chewiness (kg·f), gumminess (kg·f), and springiness (%), were calculated from the force-time curves recorded for each sample using the equipment mentioned above. The test and pre-test speeds were equipped with 80% compression, 2.0 mm/s crosshead, 50 kg full-scale load and subsequently, a 2.0 s waiting time was considered.
The color of the egg white was measured using a colorimeter (CR410 chromometer), and the Hunter color value CIE L* was determined. The egg white was minced, the mixture was spread equally on the cup, and the cup surface was flattened. Before analysis, the device was calibrated using a standard black-and-white plate. Every measurement was made in triplicate (n=3).
The surfaces of the egg whites were observed using a gemological microscope (KWs8000, PAT Częstochowa, Częstochowa, Poland) using egg white slices (1–2 cm, thickness). Surface photographs of the samples were obtained using a digital camera (SONY1880, Sony, Tokyo, Japan).
Fourier transform infrared spectroscopy (FTIR) analysis was performed using Bruker ALPHA spectroscopy for each egg at every time point and temperature condition. A small section was removed from the boiled eggs and placed in an FTIR machine. Averaging 32 scans were done for each spectrum (scan 4,000–400 cm–1), which was recorded at a resolution of 2 cm–1. Measurements were made of the absorbance unit’s variation with wavenumber.
The presence of Salmonella sp. was examined in boiled eggs held at various temperatures using a modified version of the technique published by Grijspeerdt and Herman (2003) with some modifications. The egg yolk was separated from the egg white and the white was homogenized in 10 mL of buffered peptone water and xylose deoxycholate agar media and incubated at 37°C for 24 h, and the presence of the colonies was determined.
According to Hughes et al. (2002) with some adjustments, the free amino acid content of hard-boiled eggs held for 48 h at three different temperatures was ascertained. The egg whites were minced separately. Then, 3 g of minced egg whites were mixed with 27 mL 2% (w/v) trichloroacetic acid solution and homogenized for 1 min at 13,500 rpm. After homogenization, the samples were refrigerated for 1 h, followed by centrifugation at 17,000×g for 15 min and filtered through a 0.45 μM membrane filter. The samples were analyzed using HPLC. The conditions were as follows: cation separation column (LCAK07/li), 4.6×150 mm; buffer change (A: pH 2.90, B: pH 4.20, C: pH 8.00; lithium citrate buffer solution) with a buffer flow rate: 0.45 mL/min, ninhydrin flow rate: 0.25 mL/min, column temperature: 37°C during the analysis. The free fatty acid content was expressed as mg/100 g of egg white.
Results and Discussion
The eggs were boiled for 15 min at 95°C–100°C. Hard-boiled eggs stored at different temperatures were evaluated at 0, 12, 24, 36, and 48 h and presented in Table 1. Among the three conditions (25°C, 4°C, and –18°C), frozen eggs were the hardest and caused an egg white structure turnover when frozen, forming ice crystals. The size of the ice crystals determined the hardness of the egg white, and the hardness increased with larger ice crystals (Cotterill, 1995; Davis et al., 1952). The hardness increased gradually during storage (p<0.05). The refrigerator condition led to a lower hardness value than the room and freezing temperatures, which could be attributed to proteolytic enzyme activity being affected at different temperatures. A decrease in water content, pH, and temperature causes a decrease in protease activity (Shu et al., 2016). It appeared that the combined effect of temperature, aw, and pH significantly influenced enzyme activity compared to the influence of single environmental factors. The water in the cooked egg white’s elastic gel (a denatured protein) migrates as it freezes, growing the size of the ice crystals. A portion of the elastic tension was released as the crystals expanded, penetrating the gel and separating the structure. The gel structure contracted as a result of the migration of water from within, the force of ice crystal development, and the release of elastic tension through mechanical fracture. As evidenced by the residual liquid-filled holes, this constriction was largely irreversible. As a result, the texture of the frozen egg white was different from the others in this study (Cotterill, 1995; Davis et al., 1952).
As storage time extended, there was a significant difference in the hardness of eggs stored at ambient temperature and under refrigeration (p<0.05), although not in a predictable way. When comparing the three conditions together with the storage time, there was a significant difference (p<0.05) among the treatments. The chewiness of boiled egg white gradually increased under freezing conditions compared to that of the other two conditions. Woodward and Cotterill (1986) reported that when boiled egg whites were frozen, they developed a rubbery texture. Therefore, the chewiness of the egg white increased under freezing conditions. Eggs stored under freezing conditions showed significant differences with increased storage times (p<0.05). However, the room temperature and refrigeration conditions did not show any significant differences with storage time (p>0.05). In addition, there was a significant difference (p<0.05) between the treatments with respect to temperature.
The gumminess of boiled eggs is mainly affected by freezing conditions. Freezing of hard-boiled egg white resulted in ice crystal formation. Large ice crystals left large holes and clefts. This was especially pertinent because no appreciable amount of water forced from the coagulum structure during freezing was re-absorbed during thawing. Practically irreversible water separation occurred, and the egg white became watery. Furthermore, this watery texture increased the gumminess of hard-boiled egg whites (Cotterill, 1995; Davis et al., 1952). It showed a gradual increase under freezing conditions compared with the other two conditions. However, the refrigerated and freezing conditions showed significant differences with storage time (p<0.05). The cohesiveness of boiled eggs stored under freezing conditions was higher than that of eggs stored under room and refrigerator conditions (p<0.05). In addition, cohesiveness increased as the freezing time increased (p<0.05).
Eggs stored at room temperature and refrigerated temperatures showed similar values. However, springiness did not change, except for 12 h storage under the three conditions. All eggs have two edible parts: white (or albumen, W), and yolk (Y). The egg white proteins are ovalbumin, ovomucin, and ovomucoid, which form intermolecular linkages, yielding the gel structure of the egg white (Abeyrathne et al., 2013). During boiling, egg white proteins are denatured. This is the reason for the springiness of egg whites. A higher gelling ability or gel strength results in better springiness (Jirgensons, 1936). Eggs stored under both conditions did not show any significant difference in springiness with storage time (p>0.05).
The production and maintenance of tiny ice crystals during freezing is one technique to reduce the disruption of the cooked egg white’s structural integrity. Small crystals grow as a result of supercooling. The ideal temperature range for supercooling cooked egg whites is freezing at –18°C to –12°C (Cotterill, 1995). In addition, freezing destroys the cell membrane, resulting in an increase in free electrolytes, which increases the egg’s conductivity and results in differences in all TPA parameters during freezing storage (Fuentes et al., 2013). The textural changes in boiled eggs have not been determined. However, there is some explanation for textural changes in meat products (Sheldon and Kimsey, 1985). The current analysis suggests that freezing hard-boiled eggs may become watery and rubbery upon thawing, and the yolks may turn brittle or acquire an unpleasant texture that can affect their overall quality. Although the values of the eggs maintained at room temperature and those kept in the refrigerator were comparable, holding hard-boiled eggs at room temperature increases the chance of bacterial development and deterioration, raising questions about possible food safety. However, putting hard-boiled eggs in the fridge reduces moisture loss, preserving the eggs’ texture and flavour and stifling the growth of bacteria.
Color is an important organoleptic parameter in terms of eating quality. Egg white is normally white, and off-colors are considered undesirable (Wahba et al., 2014). The CIE L* mainly focuses on CIE L*, and during the storage of the hard-boiled eggs, the CIE L* was reduced in all three storage conditions (Table 2). However, the level of reduction was not significant under refrigerated conditions (p>0.05), whereas, at room temperature, a significant reduction (97.69±1.15 to 93.84±1.27) was observed (p<0.05; Table 2). The reduction in CIE L* of the frozen eggs was not significant after 12 h of storage (p>0.05). Therefore, storing eggs under refrigerated conditions does not change the CIE L*. One explanation for this might be that refrigeration enhances the quality of boiled eggs by lowering the rate of lipid oxidation in the yolk and browning processes in the egg whites. Therefore, proper refrigeration can help extend the shelf life and maintain the quality of boiled eggs.
The egg white and yolk proteins are denatured by heat treatment. For instance, the egg white’s ovomucin creates a structure that resembles gel. These changes are mainly responsible for the rigid, solid structure of boiled eggs, and the cooking temperature and time determine the solid structure of boiled eggs (Luo et al., 2020; Modi et al., 2008; Sheldon and Kimsey, 1985). A gemological microscope provides an image of a solid structure that can be evaluated when light passes through it. Based on the images taken, a change in the egg white surface can be observed after 48 h of storage under all three temperature conditions (Fig. 1). The structure of the egg yolk is harder in the frozen condition than in the rest. Compared with the control (freshly boiled eggs), the structural breakdown of the egg white is lower at 48 h of storage in the refrigerated eggs than in the other two storage conditions. The breakdown of the structure in frozen eggs may be due to the thawing and freezing of eggs.
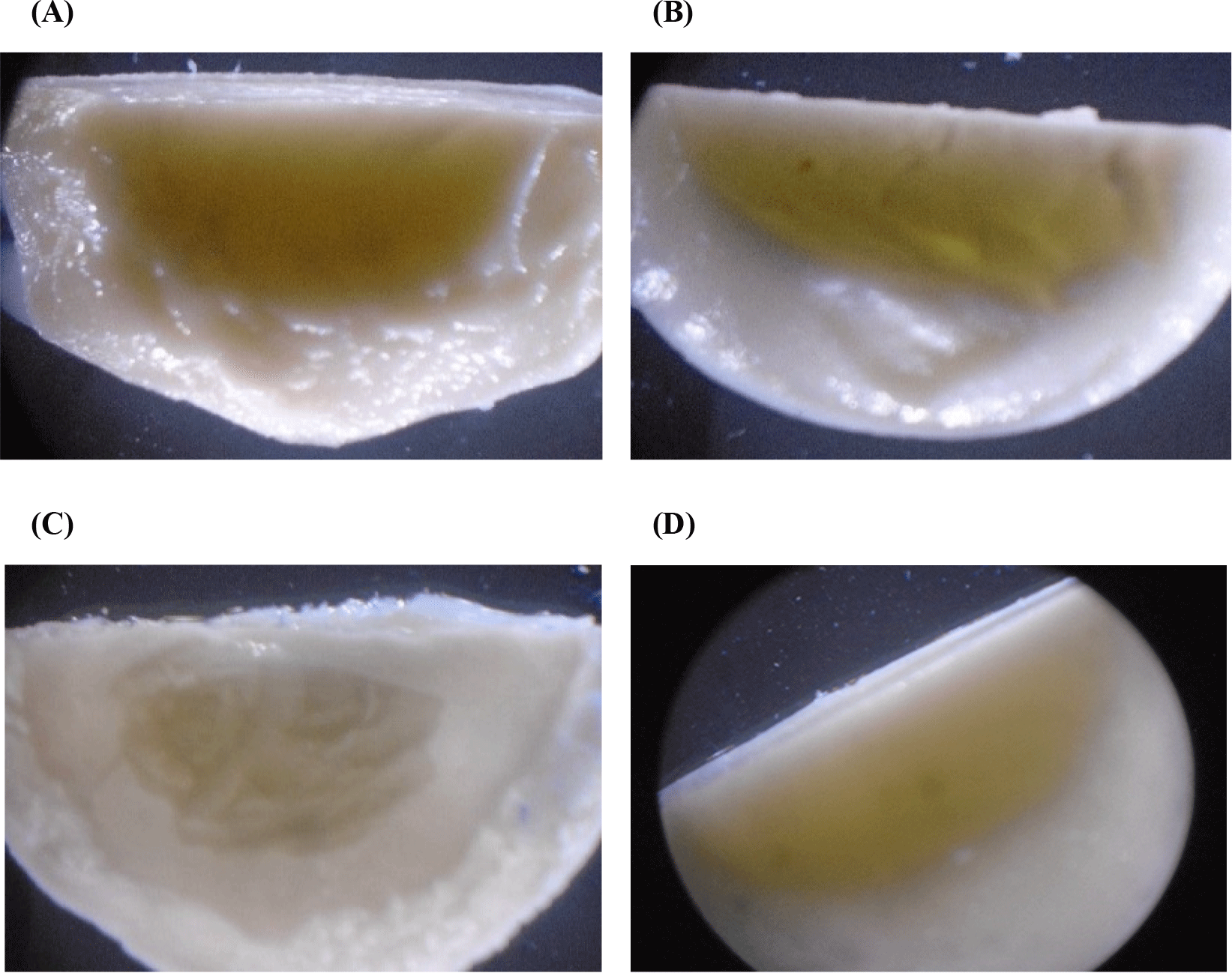
FTIR results obtained in the range of 400–4,000 cm–1 showed that chemical changes occurred in boiled eggs stored at room temperature, refrigerated, and frozen storage conditions with different storage times. From curve fitting, secondary structures were identified in the range of 2,000–3,000 cm–1 (Großhans et al., 2018). Accordingly, two main peaks were observed in the range of 3,500–3,000 cm–1 and 2,000–1,500 cm–1 (Fig. 2), which showed differences in the chemical structures of boiled eggs stored at different temperatures. When considering egg albumin stored under three conditions, peaks were observed owing to the bonds at 3,275 cm–1 for amide A (N-H stretch in resonance with amide II overtone), 1,626 cm–1 for amide I (mainly C=O stretch), and 1,538.95 cm–1 for amide II (N-H bend in the plane and C-N stretch; Garidel and Schott, 2006). The bigger peaks at 3,275 cm–1 and 1,626 cm–1 indicated that the eggs showed chemical changes in the bonds of amide I (mainly C=O stretch) and amide II (N-H bend in a plane and C-N stretch). These bonds were observed in all the eggs stored under the three storage conditions.
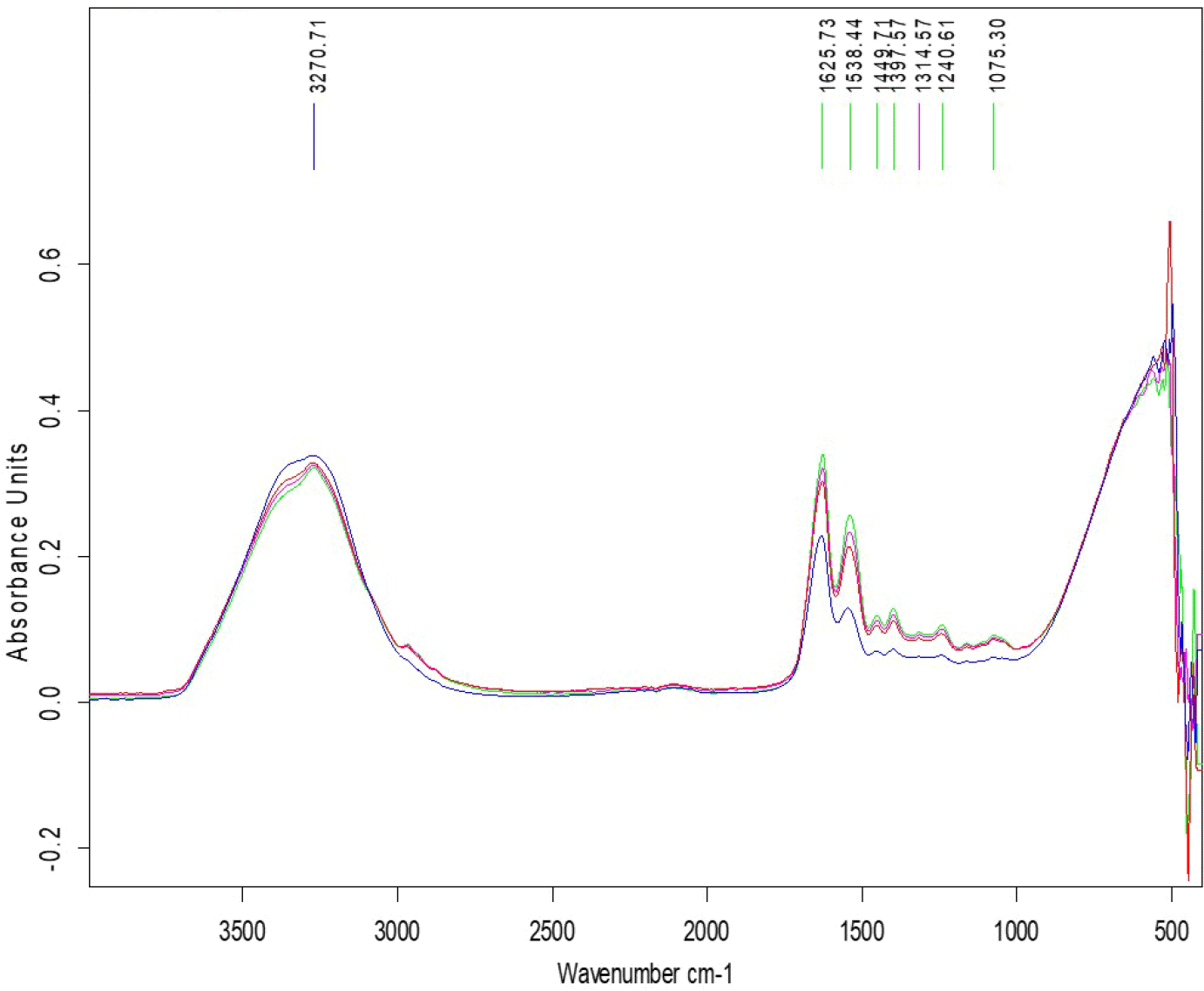
Proteins are the major component of egg whites, and many types of proteins are found in egg whites. The most abundant proteins in egg whites are ovalbumin (54%), ovotransferrin (12%), ovomucoid (12%), ovomucin (3.5%), and lysozyme (3.0%; Rathnapala et al., 2021). By comparing the FTIR spectrums obtained for individual major proteins, ovalbumin included C-H stretching, Amide I, Amide III and phenylalanine peaks; lysozyme included C=O stretching, C-N stretching, N-H stretching, OCN bending, Amide I, Amide II and Amide III (Großhans et al., 2018); ovomucoid included Amide I and sugars (Giosafto et al., 2016); ovomucin included the peaks with the 3,272.20 cm–1 bonds of Amide A (N-H stretch in resonance with amide II overtone), the 1,629.14 cm–1 bonds of Amide I (mainly C=O stretch), the 1,534.53 cm–1 bonds of Amide II (N-H bend in plane and C-N stretch), the 1,395.99 cm–1 C=O stretch of COO-, 1,314.91 cm–1 for Amide III (N-H bend in plane and C-N stretch) and 1,236.18 cm–1 for Amide III (N-H bend in plane and C-N stretch; Garidel and Schott, 2006); ovotransferrin include the peaks with 3,273.54 cm–1 the bonds of Amide A (N-H stretch in resonance with amide II overtone), 2,961.32 cm–1 the bonds of asymmetric CH2 stretching, 2,934.55 cm–1 the bonds of asymmetric CH2 stretching, 2,874.84 cm–1 the bonds of symmetric CH2 stretching, 1,631.74 cm–1 the bonds of Amide I (mainly C=O stretch), 1,534.23 cm–1 the bonds of Amide II (N-H bend in plane and C-N stretch), 1,390.52 cm–1 for C=O stretch of COO- and 1,240.15 cm–1 for Amide III (N-H bend in plane and C-N stretch; Garidel and Schott, 2006) were not changed in the eggs stored at different temperatures (Table 3). This indicates that the storage temperature does not have an impact on the structural changes in hard-boiled eggs up to 48 h of storage.
Salmonella is one of the most typical germs to cause food poisoning worldwide (Galiş et al., 2013). The presence or absence of Salmonella is an indicator in microbiological analysis to determine microbial contamination of the stored eggs. The methods used to produce eggs, store them, handle them, and prepare food are all potential sources of Salmonella contamination (Food and Drug Administration, 2009). The present findings showed that Salmonella spp. contamination was only found in eggs kept at room temperature for 48 h. Most of the bacteria can flourish and proliferate quickly between a range of temperatures, commonly referred to as the “danger zone” (about 4°C to 60°C; Fetterman et al., 2016). In addition, the bacteria might have had enough time to multiply and reach detectable levels during the 48 h period. One explanation for this could be that hard-boiled eggs were stored at room temperature, which creates a perfect habitat for any remaining bacteria that survived the cooking process, including salmonella, to grow quickly. Keeping hard-boiled eggs at a cool temperature significantly reduces the risk of bacterial growth and helps maintain their safety and quality. It was concluded from this that keeping boiled eggs at room temperature is not a viable storage strategy.
Egg proteins and amino acids are important nutritional supplements. The total amino acids range from 10.0–10.1 mg/g (Attia et al., 2020). However, the composition of the amino acid content varies based on the hen’s diet (Attia et al., 2020; Jones and Musgrove, 2005). The amount of free amino acids was significantly higher in boiled eggs stored at room and refrigerated temperatures than in other groups (Table 4; p<0.05). The highest amino acid content was observed when hard-boiled eggs were stored at room temperature. The amino acid composition of frozen and freshly boiled eggs was similar for all the tested amino acids. With storage at room temperature and under refrigerated conditions, auto degradation of proteins may occur. This could increase the levels of free amino acids during storage. Boiling egg whites that were stored at high temperatures had a larger concentration of free amino acids than those that were stored at low temperatures (Luo et al., 2020). The possibility is due to the fact that amino acids were more readily produced from proteins, polypeptides, and proteases
2) Sum of aspartic acid, threonine, serine, asparagine, glutamic acid, glycine, alanine, and lysine.
stored at high temperatures as opposed to low temperatures. The free amino acid content can influence the flavor of the egg. A total of 13 essential amino acids (DESHGTAYVMWIP) have been found in egg whites (Goto et al., 2021). However, these values are much lower than those recorded in previous studies. This shows that the level of amino acids produced during storage may not affect the final product’s flavor under any storage condition. Even though the total free amino acid content in eggs stored at refrigerated temperature (44.89 mg/100 g) was higher than that in freshly boiled eggs (13.70 mg/100 g), the ratio between the non-bitter and bitter amino acids was not significantly different (p>0.05). According to the present investigations, when compared to freshly hard-boiled eggs, the presence of more free amino acids at room temperature and refrigerator storage could potentially cause flavor alterations. Free amino acids play a role in flavour creation and can affect how food tastes overall. Therefore, storing hard-boiled eggs at room temperature or in the refrigerator increases their free amino acid content, potentially leading to richer flavors compared to freshly boiled eggs.
Conclusion
According to our observations, boiled eggs can be stored under refrigerated conditions (4°C) without significant physical, structural, or chemical changes for up to 48 h. The freezing of eggs destroys the structure of boiled eggs, while room temperature leads to microbial spoilage. This study profiled the quality characteristics of boiled eggs stored at different temperatures. However, further studies on the functional compounds at different storage- temperatures are necessary.