Introduction
Fresh meat, recognized as one of the most perishable food, is very conducive to the growth of microorganisms, for its high water content (aw>0.85), optimal pH for microbial growth (5.5−6.5), as well as abundant nutrients that provide energy (Buncic et al., 2014; Doulgeraki et al., 2012; Nychas et al., 2008). It has been confirmed that the spoilage of fresh meat is caused mainly by microorganisms especially bacteria (Pellissery et al., 2020). Several studies have demonstrated that bacterial communities play an important role in spoilage of meat, and the spoilage status is closely related to the species and amount of bacteria under specific storage conditions (Kaur et al., 2021; Li et al., 2019; Mansur et al., 2019). The composition and change of spoilage microflora of fresh meat are primarily determined by many factors including the slaughter and processing environment, chilling method, packaging, and storage temperature (Erkmen and Bozoglu, 2016; Nychas et al., 2008; Pellissery et al., 2020).
In the process of fresh meat, chilling is applied to preserve the carcass from microbiological contamination (Reid et al., 2017). Carcass must be chilled immediately after slaughter and dressing to ensure quality and safety (Buncic et al., 2014; Nychas et al., 2008). Apart from the conventional chilling and fast chilling, spray chilling, multistep chilling and very fast chilling are also applied in modern meat production enterprises (Zhang et al., 2019). Very fast chilling can be recognized as a special chilling technology which demands the temperature of muscle should be chilled to −1°C within 5 h after slaughter (Joseph, 1996). Research has reported that very fast chilling could provide tender meat by inhibiting the occurrence of “cold shortening” (Zhang et al., 2019). Due to the faster decrease of carcass surface temperature, very fast chilling can reduce evaporative losses and improve the economic effectiveness in meat industry (McGeehin et al., 2002). The chilling rate of carcass is recognized as an important hazard control point for abattoirs, and the faster freezing rate showed stronger inhibition of microbial proliferation (Zhang et al., 2019). Compared to the conventional chilling treatment, very fast chilling treatment can significantly reduce the number of microorganisms on the surface of lamb carcasses (Omer et al., 2015). However, there are few studies about the effects of very fast chilling on the composition and change of bacteria in fresh meat during storage.
Storage temperature is considered the main factor that affects the shelf life of fresh meat (Nychas et al., 2008). Storage of foods at temperatures above freezing and below 15°C is known as refrigerated or chilling storage (Karel et al., 1975). Chilling and frozen storage are the most two popular storage techniques on the market in the past few decades (Lu et al., 2019). The application of frozen storage could extend the shelf life of fresh meat significantly compared to chilling storage. Freezing and thawing can result in the reduction of water holding capacity, the increase of drip loss and the denaturation of protein, which affect the quality of fresh meat (Leygonie et al., 2012). The temperature range of supercooling is between 0°C and below its usual freezing point without the phase change solidification (formation of ice crystals) occurring (Stonehouse and Evans, 2015). Moreover, supercooling storage could inhibit the activity of enzymes and the growth of microorganisms in fresh meat, thereby the shelf life of fresh meat could be prolonged by 1.4−4 times at least compared to ordinary chilling storage (Magnussen et al., 2008; Pomponio et al., 2018; Zhou et al., 2010). In recent years, superchilling and sub-freezing are explored to be a new storage technology (Lu et al., 2020; Soyer et al., 2010; Wang et al., 2018). The temperature range of superchilling is lowered to 1°C–2°C below the initial freezing point of the product, with the conversion of some water into ice, which makes it less available for deteriorative processes (Aune, 2003; Duun and Rustad, 2007). The temperature range of sub-freezing is between superchilling and freezing temperature. Based on the results of low field nuclear magnetic resonance, −6°C, −9°C, and −12°C were designated as sub-freezing temperatures (Qian et al., 2018). It has been reported that the growth of toxin-producing microorganisms and bacteria on the meat surfaces could be inhibited by the low storage temperatures (Magnussen et al., 2008). To date, there have been many studies focused on the quality of fresh meat, but the change of microbial composition under different storage temperatures has rarely been reported.
Thus, the purpose of the work was to evaluate the effect of different storage temperatures (4°C, −1.5°C, −4°C, and −9°C) on the shelf life, bacterial communities and dominant spoilage bacteria of very fast chilled fresh lamb meat. Spoilage indicators, including total viable counts (TVC), total volatile base nitrogen (TVB-N) and thiobarbituric acid reactive substance (TBARS) were used to evaluate shelf life. The dynamic change of spoilage-related bacterial communities and dominant spoilage bacteria were also determined. Additionally, the potential mechanism of spoilage bacteria was investigated by functional analyses. The results may provide a novel approach to extend the shelf life of fresh lamb meat.
Materials and Methods
A total of 24 seven-month old male lamb carcasses (small-tail Han sheep) with the same feeding system were collected at a commercial abattoir (Hebei Jinhong Halal Meat, Baoding, China). After slaughtered, the lamb carcasses were immediately chilled in a chilling room at −35°C air temperature with air velocity of 3 m/s. Room temperature was recorded with a temperature probe (LK-U, Changzhou blue light electronic, Changzhou, China) placed in the surrounding of carcass area, and the core temperature of carcass was measured by inserting a temperature probe into the 12th to 13thMusculilongissimusthoracis (M. longissimus) about 2 cm. When the temperature of the center of M. longissimus decreased to −1°C, carcasses were transferred to the conventional chilling room (0°C−4°C) for 24 h. The muscle samples of M. longissimus from both sides of each carcass were cut off and the visible fat and connective tissues were removed immediately after chilling. The M. longissimus from one lamb carcass were packed into one sterile sampling bag (Shockmixer-1, Guangdong Huankai Microbial Sci. & Tech., Guangzhou, China). All the sterile sampling bags were transported to the laboratory on ice. In the laboratory, every piece of M. longissimus was divided equally into 5 pieces and totally 10 pieces were obtained from one lamb carcass. Every piece of lamb meat was packed into a polypropylene tray (14.2 cm×10 cm×2 cm) and covered with a film with an O2 permeability of 18,500 cm3 m−2 day−1 at 25°C and 1 atm. The packages from one lamb carcass were randomly stored at different temperatures and marked as chilling, supercooling, superchilling and sub-freezing, respectively. Every treatment had 60 samples and 6 pieces of lamb meat from 6 lamb carcasses in each treatment were collected randomly at sampling timepoints. The collected samples were placed on ice for the determination of TVC, TVB-N and TBARS. 10 g of lamb meat were segmented from each samples and stored at −80°C for bacterial analysis.
The TVC was measured in accordance to a reference method of Wang et al. (2020a) with minor adjustment. 10 g of lamb meat were weighed accurately by using sterile scissors and added into a sampling bag (Shockmixer-1, Guangdong Huankai Microbial Sci. & Tech., Guangzhou, China) containing 90 mL of sterile saline. The collected samples were extracted in a sterile equalizer mixer (JN-400i, Ningbo Jiangnan Instrument Factory, Ningbo, China) for 1 min. The extraction was serially diluted with sterile saline containing 0.9% NaCl and 0.1 mL of diluent was plated onto agar plates (CM101, Beijing Luqiao Technology, Beijing, China) for counting. The plates were incubated at 36±1°C for 48 h. The results were expressed in Log CFU/g.
The TVB-N concentration was measured in accordance to Chinese standard GB 5009.228 (National Health and Family Planning Commission of China, 2016). The fresh lamb meat was minced by a meat mincer (JYL-C022E, Joyoung, Jinan, China). Minced lamb meat (10 g) was transferred into the distillation tube. Then 75 mL distilled water was added and homogenized for 30 min. At last, 1 g magnesium oxide was added into the distillation tube containing the processed sample. The content of TVB-N was determined by the Automatic Kjeldahl nitrogen analyzer (K9840, Hanon Instruments, Jinan, China). The results were expressed as mg of TVB-N per 100 g of lamb meat.
The TBARS concentration was measured in accordance to a reference method described by Sinnhuber et al. (1977) with minor modifications. Minced lamb meat (2 g) was weighed into the 50 mL centrifugation tube containing 3 mL of 1% thiobarbituric acid solution, 17 mL of 2.5% trichloroacetic acid-HCl solution, and 1 mL of butylated hydroxytoluene. The mixture was heated in boiling water for 30 min and then cooled to room temperature. Then the mixture was mixed with isometric chloroform, followed by centrifugation at 845 g for 10 min. The absorbance of the supernatant was determined at 532 nm. The TBARS value was expressed as mg of malonaldehyde/kg of the minced lamb meat.
The total DNA was extracted from lamb samples using the FastDNA® Spin Kit for Soil (MP Biomedicals, Solon, Ohio, USA), following the manufacturer’s instructions. 1% agarose gel was used to check the DNA extract, and NanoDrop 2000 UV-vis spectrophotometer was used to determine the DNA concentration and purity. To investigate the bacterial community diversity, the hypervariable region V3–V4 of the bacterial 16S rRNA gene were amplified with primer pairs 338F (5'-ACTCCTACGGGAGGCAGCAG-3') and 806R (5'-GGACTACHVGGGTWTCTAAT-3') by an ABI GeneAmp® 9700 PCR thermocycler (ABI, CA, USA). The PCR mixtures were carried out in 20 μL reactions including 0.8 μL (5 μM) forward and reverse primers each, 4 μL 5×TransStart FastPfu buffer, 2 μL 2.5 mM dNTPs, 0.4 μL TransStart FastPfu DNA Polymerase, 10 ng template DNA, and complemented ddH2O to 20 μL. The amplification of the 16S rRNA genes consisted of initial denaturation at 95°C for 3 min, followed by 30 cycles of denaturing at 95°C for 30 s, annealing at 55°C for 30 s, extension at 72°C for 45 s, single extension at 72°C for 10 min, and end at 10°C. The amplified product was visualized on 2% agarose gel and further purified using the AxyPrep DNA Gel Extraction Kit (Axygen Biosciences, Axygen, CA, USA) according to the manufacturer’s instructions. The target segment was sequenced on Illumina MiSeq PE300 platform (Shanghai Majorbio Bio-pharm Technology, Shanghai, China). The raw sequence data was submitted to the NCBI as BioProject PRJNA700819.
The raw 16S rRNA gene sequencing reads were demultiplexed, quality-filtered by FASTP version 0.20.0 (Chen et al., 2018) and merged by FLASH version 1.2.7 (Magoc and Salzberg, 2011). In brief, the operational taxonomic units (OTUs) with 97% similarity cutoff were clustered using UPARSE version 7.1 (Edgar, 2013; Stackebrandt and Goebel, 1994), and chimeric sequences were identified and removed to guarantee the tags effectively. The taxonomy of each OTU representative sequence was analyzed by RDP Classifier version 2.2 (Wang et al., 2007) against the 16S rRNA database (silva 138/16s_bacteria) using the confidence threshold of 0.7.
All of the tests were carried out in sextuplicate. One-way ANOVA implemented in SPSS 20.0 software (IBM, Chicago, IL, USA) was used to analyse the differences in mean values for TVC, TVB-N, and TBARS. Duncan's multiple range test was performed to verify significant differences (p<0.05) among samples. For sequencing data, Mothur program (version: 1.30.2) and QIIME pipeline were implemented to analysis the alpha and beta diversity. The bacterial community structural component was analyzed based on the statistical software package R (version 3.1.2). PICRUSt2 and Genomes (KEGG) pathways were applied to predict the functional genes of bacterial communities.
Results and Discussion
Microorganism is one of the main factors affecting the shelf life of fresh meat (Hong et al., 2012). Changes in TVC of all four treatments were shown in Fig. 1A. The initial count for TVC was about 3.8 Log CFU/g for four treatments and increased with the prolonging of storage time. The TVC values of chilling and supercooling increased remarkably at 10 d and 21 d respectively compared with 1 d (p<0.05). The shelf life of chilling and supercooling treatment were 14 d and 28 d, respectively. At the end of storage, the TVC values of chilling and supercooling were up to 7−8 Log CFU/g, which exceeded the upper tolerable limit for fresh meat (Bolton et al., 2009), whereas the TVC values for superchilling and sub-freezing were still below 5 Log CFU/g. These results indicated that the low temperatures could inhibit the activity of microorganisms, consistent with previous results (Lu et al., 2019; Pellissery et al., 2020).
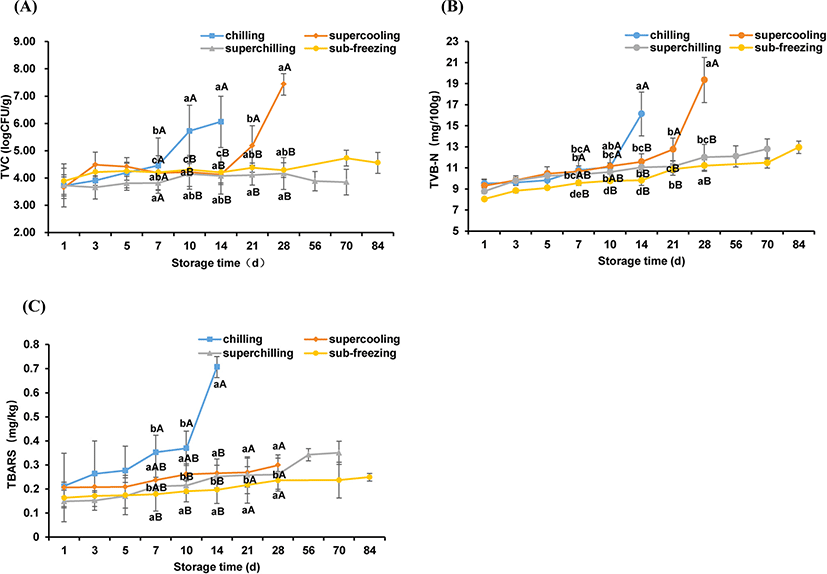
TVB-N is an important index to reflect the freshness of meat (Wang et al., 2020b). As shown in Fig. 1B, the TVB-N values showed an increasing trend for all treatments during storage. The TVB-N of chilling exhibited the highest rate of growth from 11.07 mg/100 g to 16.12 mg/100 g on the 14 d. The significant change of TVB-N occurred after 21 d storage for supercooling (p<0.05). Meanwhile, the TVB-N of superchilling and sub-freezing were still lower than 15 mg/100 g even at 84 d. According to the current Chinese hygienic standard (GB 2707–2016), the upper limit of TVB-N value in fresh meat is 15 mg/100 g (Lu et al., 2019). It has been reported that spoilage bacteria metabolites could cause the protein to be degraded, which lead to the production of TVB-N, and the low temperatures can inhibit bacterial reproduction (Chung et al., 2019; Mansur et al., 2019; Muela et al., 2010). These results indicated that the increase of TVB-N was influenced by the storage temperature. In the commercial refrigeration condition, the shelf of fresh lamb meat is less than 2 weeks, hardly over-passes 21 days (Berruga et al., 2005; Karel et al., 1975). Based on the results of TVC and TVB-N, the shelf life of fresh lamb meat could extend more than 70 days at superchilling or sub-freezing storage.
The TBARS value is mainly used as a marker of lipid oxidation of meat by evaluating the formation of the secondary products (Dai et al., 2014). As shown in Fig. 1C, the TBARS values for all treatments increased linearly throughout the storage period. The TBARS value of chilling exhibited a higher rate of increase compared to other treatments at d 14 (p<0.05), which is same as TVC and TVB-N for chilling. It is reported that when the TBARS value exceeds 0.5 mg/kg, the trained sensory panels could detect some unpleasant flavors (Hansen et al., 2004). On the last day of storage, the TBARS values of supercooling, superchilling and sub-freezing were still lower than the limit of 0.5 mg/kg. The accumulation of TBARS may contribute to the hydroperoxide production by lipid oxidation and the hydroperoxide is likely to be degraded rapidly, and these reactions is correlated with the occurrence of rancidity and the activity of spoilage bacteria (Ganhão et al., 2011; Li et al., 2019). The present results indicated that the lower temperature (−1.5°C, −4°C, and −9°C) could restrain lipid oxidation to some degree.
For all treatments, there is a good correlation between TVC and TVB-N (R2>0.92), which is in agreement with previously reported results (Hernández-Macedo et al., 2012; Li et al., 2019). These results indicated that TVC and TVB-N could be used as spoilage indicators of fresh lamb meat. Except for superchilling and sub-freezing samples, TVC and TBARS were correlated well (R2>0.92) (Table 1). The TBARS values are affected by the activity of microorganisms and the oxidation of meat itself (Resconi et al., 2018; Tuboly et al., 2003). Compared with 4°C and –1.5°C storage, the lower temperature (−4°C and −9°C) inhibited the activity of microorganisms and the oxidation process of meat itself. Therefore, TVC and TBARS were poorly correlated for superchilling and sub-freezing treatment.
According to the results of TVC, the samples representative time points were selected including d 1, d 7 and d 14 for chilling, d 1, d 7, d 14, and d 28 for supercooling, superchilling and sub-freezing in the present study. Data of 90 samples in total at 15 representative time points were analyzed. A total of 4,038,356 sequences were retained, after removing short and low-quality reads, singletons, triplicates, and chimeras. The total abundance, 4027 OTUs were clustered (identity 97%), ranging from 29,783 to 59,934 (Table S1). Good's coverage (more than 99%) indicated all the sequencing data could cover the most of bacteria in lamb meat samples (Table S2). The richness and diversity of bacterial communities were evaluated by Chao1, Sobs and Shannon indexes (Yang et al., 2018). For d 14 of chilling, d 28 of supercooling, superchilling and sub-freezing, the values of Chao1, Sobs and Shannon in chilling and supercooling were lower than the initial values, which was contrary to the results of superchilling and sub-freezing (Table S3). The change of Chao1, Sobs and Shannon indicated that the richness and diversity dropped rapidly in chilling and supercooling and some bacteria became the dominant spoilage bacteria in samples with the extension of storage time. It has reported that various kinds of initial bacteria may contaminate the meat, whereas only a small number of species related to meat spoilage become the major spoilage bacteria at the end of storage (Benson et al., 2014; Chaillou et al., 2015). These results indicated that the change of bacterial community structure and the formation of dominant spoilage bacteria were influenced by storage temperature, consistent with previous results (Kaur et al., 2021). Furthermore, superchilling and sub-freezing storage may extend the shelf of lamb meat by inhibiting the growth of dominant spoilage bacteria and reduce the further risk of microbial at a certain extent.
To compare the differences in bacterial community composition among samples, PCoA was an effective method (Fig. 2A). The samples of chilling stored on d 7 were separated compared to d 1, suggesting that the effect of storage time for the bacterial community composition was significant. Meanwhile, the samples of chilling stored on d 7 and d 14 were clustered together, suggesting that the bacterial community composition trended to be stable. The bacterial community composition of supercooling and superchilling became stable after d 7, which had the same trend as chilling. There was an interesting observation that the change of bacterial community composition was not significant for sub-freezing during storage period, probably due to that the low temperature (−9°C) inhibited the growth of the main spoilage bacteria, thereby resulting in a relatively stable bacterial structure. In addition, the Venn diagram revealed that 23 taxa were common to all samples including Pseudomonas, Ralstonia, Acinetobacter, Brochothrix, Psychrobacter, Lactococcus, Vagococcus and Macrococcus, etc. (Fig. 2B), which explained the relative content of these bacteria is highly related to the structure of the bacterial community.
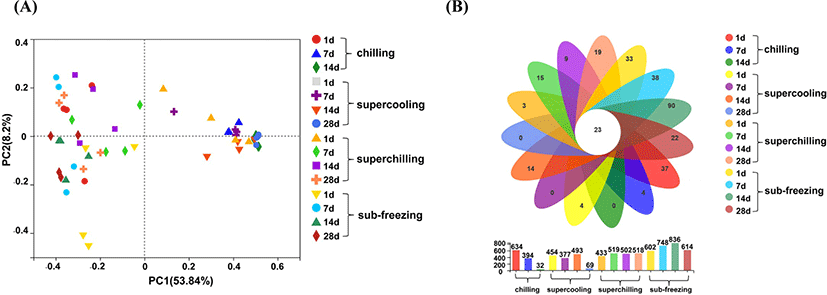
At the phylum level, a total of four bacteria were identified including Proteobacteria, Firmicutes, Actinobacteriota and Bacteroidota. Proteobacteria and Firmicutes (together represented >80% of all OTUs) were the major bacteria during storage (Fig. 3A). As the storage time increased, the proportion of Actinobacteriota and Bacteroidota decreased from 11%−14% to <3% for chilling and supercooling. Conversely, the proportion of Actinobacteriota and Bacteroidota increased from 6% to 14% for superchilling. The proportion of Actinobacteriota and Bacteroidota had no significantly change during storage for sub-freezing.
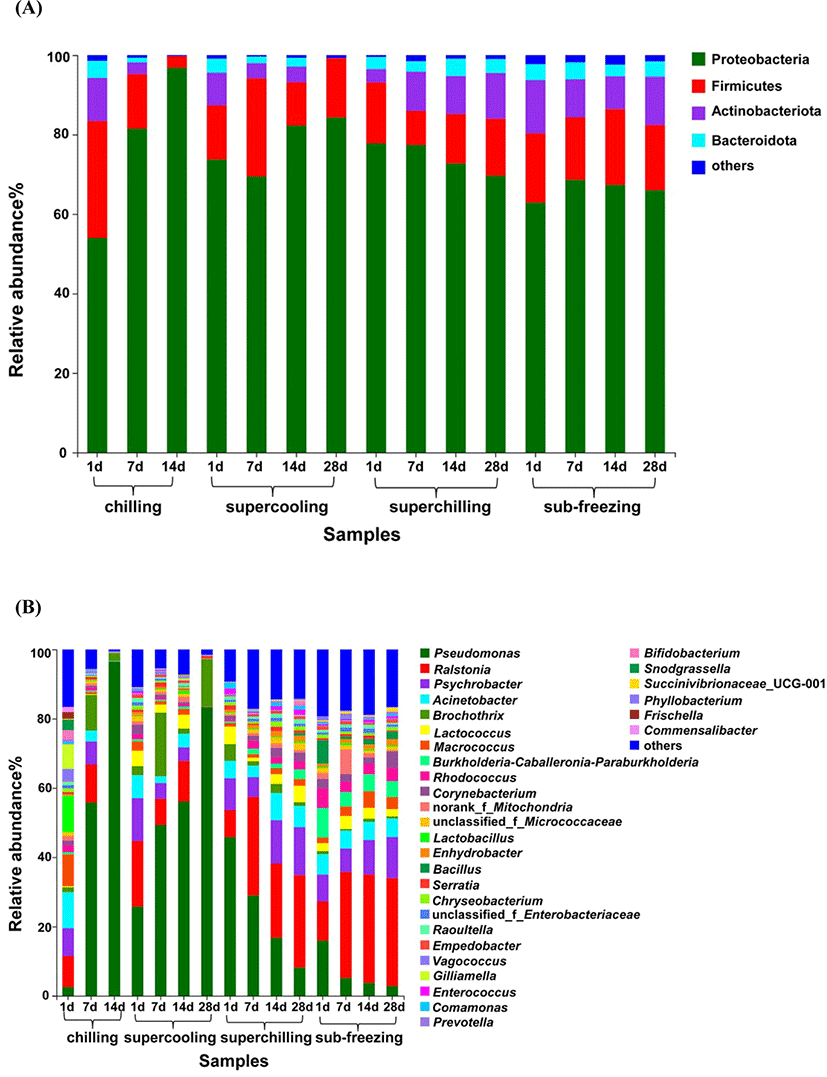
Bacterial community dynamics were also evaluated at the genus level (Fig. 3B). At the beginning of storage, the major bacteria including Acinetobacter, Macrococcus, Lactobacillus accounted for 29% and Pseudomonas accounted for only 3% for chilling. At the end of storage (d 14), Pseudomonas accounted for more than 96% which lead to a significant decrease in the bacterial diversity compared to d 1. Similar observations have been reported that Pseudomonas could account for 96% of the spoilage flora in air packing (Bailey et al., 1979; Enfors et al., 1979; Kumosinski, 1988). Pseudomonas and Brochothrix were the dominant genus during storage and they had a significant increase compared to d 1 for supercooling. At the early storage period, Psychrobacter and Pseudomonas were recognized as the main spoilage bacteria for superchilling and sub-freezing, and the proportion gradually decreased with the storage time prolonged. At the end of storage (d 28), Ralstonia became the dominate spoilage bacteria for superchilling and sub-freezing. In another study, Ralstonia was also isolated in dairy and meat processing environments (Liu et al., 2016). Ralstonia could enhance the incorporation of E. coli O157:H7 into dual-species biofilms under various temperature and nutrient availability conditions (Liu et al., 2015). There are rare reports about Ralstonia in meat, it could provide potential transference routes for foodborne pathogens (Lynch et al., 2009). The present result indicated that we need pay attention to the Ralstonia at superchilling and sub-freezing temperature. At the end of storage for chilling and supercooling, the relative content of Ralstonia was significantly lower than that of Pseudomonas (p<0.05). The result demonstrated that Ralstonia was less competitive than Pseudomonas during storage at chilling and supercooling temperature. Meanwhile, the bacterial diversity of superchilling and sub-freezing were also higher than that of supercooling on d 28, explaining that most of bacteria were inhibited and the dominated spoilage bacteria did not occur at –4°C and –9°C, which was in line with the results of TVC and TVB-N. These results indicated that the dynamic changes in bacterial community were greatly affected by the storage temperature.
In aerobic packing, Pseudomonas and Brochothrix are identified as the main spoilage bacteria for raw meat (Doulgeraki et al., 2012). More accurately, Pseudomonas is particularly involved in the spoilage of beef and pork at chilling temperatures (5°C) (Ercolini et al., 2006; Liu et al., 2006). Compared with Brochothrix, Pseudomonas became the dominate spoilage bacteria owing to its faster growth during storage period. In a study by Tsigarida et al (Tsigarida et al., 2003), they applied the gel cassette system to replace broth to simulate the spoilage environment and drew the similar conclusion. Glucose, as the first source of energy, which is metabolized more rapidly by Pseudomonads rather than Brochothrix (Erkmen and Bozoglu, 2016). Pseudomonads could oxidize glucose and glucose-6-phosphate to form D-gluconate, pyruvate and 6-phosphogluconate (Gill, 1976). Once the glucose reserves are exhausted, lactic acid is the next energy source, and then followed by amino acids (Casaburi et al., 2015). At this period, protein also can be used as the source of energy (Gill, 1976). The change in available nutrients is beneficial to the growth of microorganisms that can decompose protein (Nychas et al., 2008). In aerobically packaged meat, that the Pseudomonas could become the main spoilage bacteria was due to the ability to decompose protein (Doulgeraki and Nychas, 2013). In addition to Pseudomonads, some bacteria also have the spoilage potential such as Acinetobacter, Moraxella, and Flavobacterium (Ercolini et al., 2006). These bacterial species could compete with other organisms for oxygen, thereby promoting the growth of Pseudomonads (Gill, 1976). Among different spoilage organisms, Pseudomonas favors the breakdown of proteins and amino acids, and could produce off-odor in meat (Casaburi et al., 2015; Smit et al., 2009). Pseudomonas, as the lipolytic bacteria, could promote the lipolysis (rancidity) to produce aldehydes, ketones, and short-chain fatty acids, which results in the formation of rancid flavors (Erkmen and Bozoglu, 2016). In addition, Pseudomonas, Acinetobacter and Moraxella have been reported that they are the main spoilage bacterial in aerobical packing meat at different storage temperature (–1°C−25°C) (Pellissery et al., 2020). Pseudomonas, as the common spoilage bacteria, are also isolated from spoiled meat at low temperatures (4°C) in aerobic storage, consistent well with this study (Ercolini et al., 2007; Ercolini et al., 2010). In this study, the growth of Pseudomonas was obvious to be inhibited owing to the fact that storage temperature was far lower than the optimal growth temperature (25°C−35°C) of Pseudomonas such as −4°C and −9°C. Therefore, the bacterial community diversity increased compared to the initial storage (d 1) for superchilling and sub-freezing. These results indicated that lamb meat stored below −4°C can effectively inhibit the reproduction of Pseudomonas and then prolong the shelf life of fresh meat. However, there are still many unknown bacteria worthy of further research.
The spoilage potential of microorganism depends on their ability to produce metabolites related to spoilage (Li et al., 2019). As shown in Fig. 4 (A, B, C, and D), for samples of chilling and supercooling, the abundance of genes related to carbohydrate metabolism and amino acid metabolism began to increase after 1 d, but these two metabolic pathways were just the opposite in the samples of superchilling. For sub-freezing, the amino acid metabolism showed a trend of falling at the beginning and subsequent rising, but the carbohydrate metabolism was no regular change. For chilling, the abundance of amino acid metabolism gradually exceeded the abundance of carbohydrate metabolism with storage time increased. This may result from the fact that Pseudomonas as the dominant spoilage bacteria and it exhibited the ability to promote lipolysis and break down the protein. The abundance of two metabolic pathways decreased for superchilling and sub-freezing and this may be due to the less proportion of Pseudomonas. From this point, Pseudomonas, as the main spoilage bacteria, was also in line with the trend of two main metabolic pathways. The results also presented that the longer the storage time was, the stronger abundance of metabolism was inhibited at low storage temperature. Moreover, the abundance of metabolic pathways was also compared on d 14 for all treatments. As shown in Fig. 4E, the top two metabolic pathways are carbohydrate metabolism and amino acid metabolism, which confirms the results of the previous studies (Li et al., 2019; Reis et al., 2016).
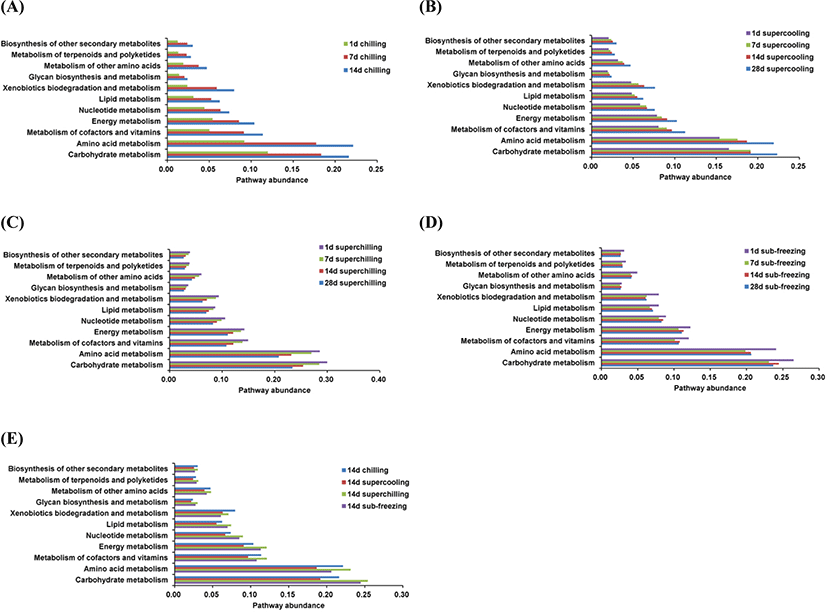
On the level of the thirdly metabolic pathway, the microbial metabolism was also inferred (Fig. 5A and B). The abundance of main metabolic pathways including ABC transporters, Biosynthesis of amino acids, Two-component system and Carbon metabolism for chilling were higher than other treatments. For chilling, the dominant microorganisms were Pseudomonas on d 14. The cold adaptability of Pseudomonas is related to various mechanisms of tolerance to cold-induced stress and the high level of unsaturated fat in the cell membrane (Moreno and Rojo, 2014). Pseudomonas could accelerate the oxidation of free fatty acids, the promotion of proteolytic degradation, and the formation of some volatile compounds including biogenic amines and sulfide formation, etc. (Dainty et al., 1985; Kakouri and Nychas, 1994; Lambert et al., 1991). Meanwhile, the metabolic pathways for chilling related to fatty acid and amino metabolisms such as arginine and proline metabolism, pyruvate and glycerophospholipid metabolism were higher than other treatments. At the same time, the TVC, TVB-N and TBARS values in chilling were higher than these in supercooling, superchilling and sub-freezing significantly (p<0.05) on d 14. Previous study showed that the content of TVB-N in aerobic packing beef is higher, which is likely to be caused by Pseudomonastaetrolens and Pseudomonasfragi (Ercolini et al., 2011). The present results proved that Pseudomonas is significantly positively correlated with TVB-N for chilling and supercooling (p<0.05) (Fig. 6). This finding confirmed that Pseudomonas could have a great impact on the spoilage process of meat. It could infer that Pseudomonas promotes the breakdown of protein and fat by improving related metabolic activities, which leads to the increase of TVB-N and TBARS values.
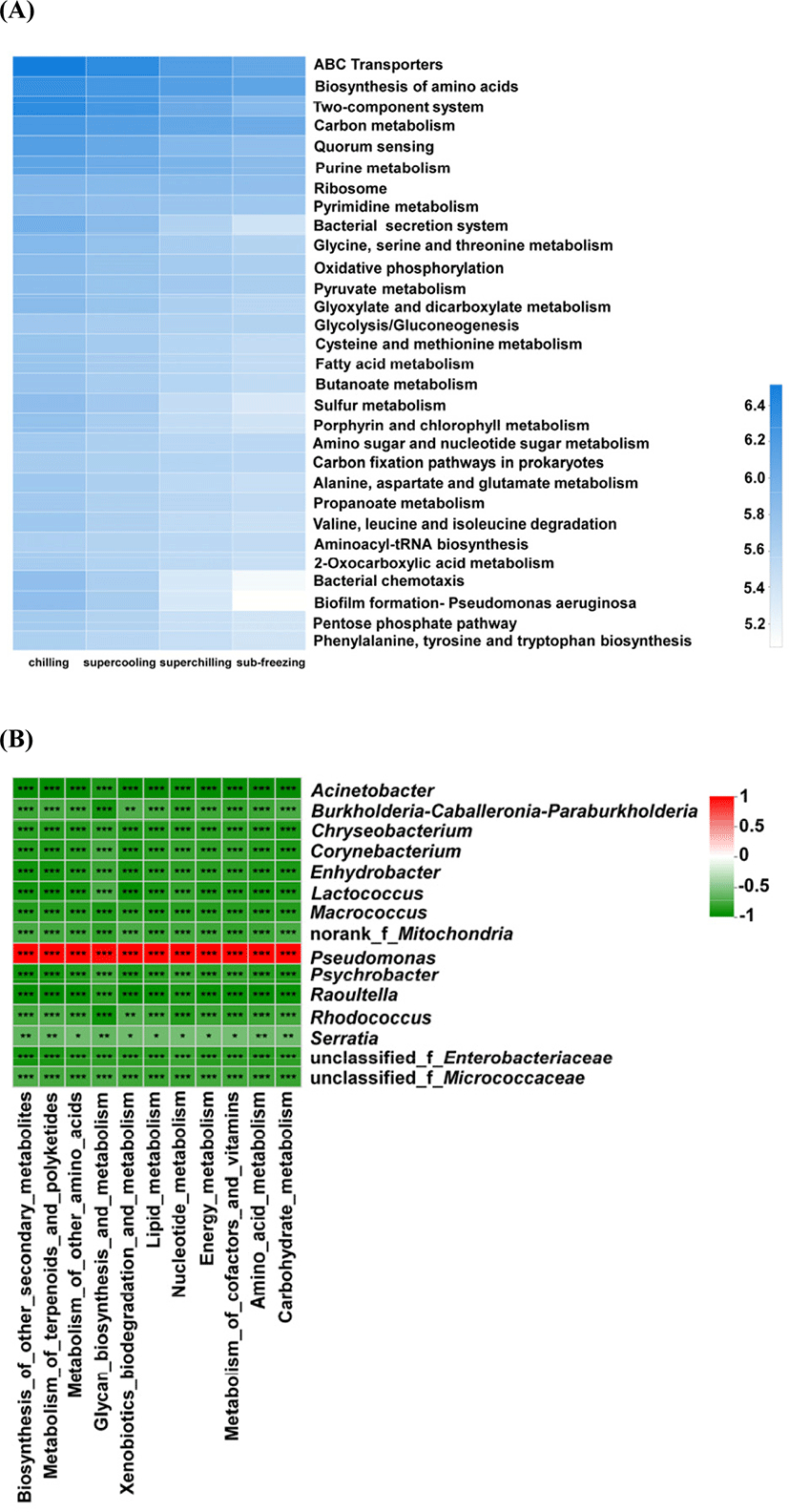
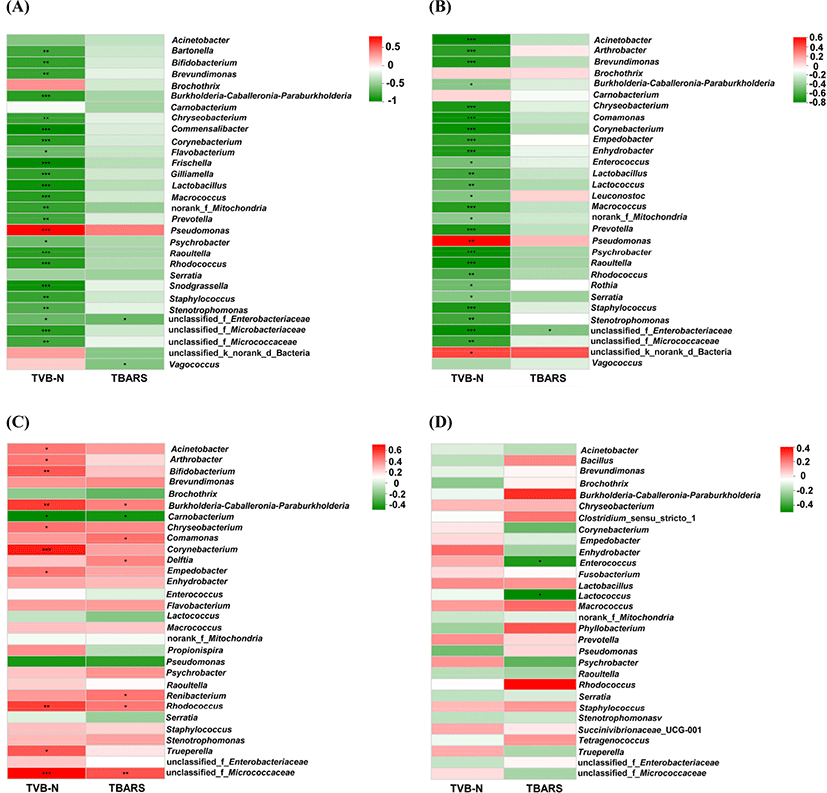
Conclusion
Storage temperature could significantly affect the shelf life and bacterial communities of the very fast chilled tray-packaged lamb meat. Compared to store at chilling or supercooling, the shelf life of very fast chilled lamb meat could be extended to over 70 d when stored at superchilling or sub-freezing. At the genus level, the dominant microorganisms were Pseudomonas followed by Brochothrix for chilling and supercooling storage, and the dominant microorganisms were Pseudomonas, Ralstonia, Psychrobacter, Acinetobacter and Brochothrix for superchilling and sub-freezing storage. Pseudomonas was the core spoilage microorganism that might improve the related metabolic activity (carbohydrate metabolism and amino acid metabolism) to promote the decomposition of protein and lipid to produce metabolites. Accordingly, it can monitor the abundance of Pseudomonas to predict the freshness of fresh tray-packaged lamb meat. Further studies on the response mechanism of spoilage microorganisms to the environmental factors are deserved to explore.