Introduction
Animal eggs have played an important role in the human diet all over the world. In addition to being an ingredient in many foods, eggs have been used for meals and baking. Duck eggs are nutritious and one of the most commonly eaten types of egg (Kaewmanee et al., 2009). Duck eggs consist of 10.87% shell, 54.73% egg white and 33.94% yolk. They provide plenty of complete, high-quality protein (which includes all amino acids essential for humans) and supply many substances with biological functions beyond basic nutrition (López-Fandiño et al., 2007).
Nevertheless, the quality of fresh eggs will degrade over time (Abdel et al., 2011). Pickling is an oldest technique in egg preservation because it not only prolongs the shelf life but also increases the flavour and acceptability (Ganasen and Benjakul, 2011). Zaodan is one of the oldest traditional pickling foods, having a long shelf life in China and being commonly popular among Chinese. It has also won warm praise from customers from Japan and Southeast Asia because of its rich bouquet with a long aftertaste. Zaodan originated from the Qing dynasty in China and it thus more than 270 years old. Traditionally, Zaodan is made by pickling duck eggs in rice wine with some salt, vinegar and black sugar at room temperature for more than 5 months (Meng et al., 2010). Generally, the egg white and yolk inch by inch become solidified and pasty. The formation of Zaodan is caused by the infiltration of ethyl alcohol and acetic acid through the eggshell and shellmembrane, resulting to chemical and physical property changes and gelation of the egg proteins.
At present, the traditional production process of Zaodan has a lot of problems. First, most of the factories pickle Zaodan at the condition of atmospheric pressure and room temperature, and the production cycle is almost 20 wk. But this method is affected by season and severely restricted the mass production of Zaodan. Second, the traditional curing method of Zaodan generally adopts manual operation, thus the production parameters are not fixed which may lead to uneven Zaodan quality. Third, the curing material liquid of Zaodan can’t use again, and its random discharge can cause to serious environment pollution.
Scientists have studied on new methods of Zaodan making to overcome the defects of traditional method which was time-consuming, inhomogenous quality and complex process. Thus, it is needful to find a technology to change this situation. In order to reduce the long pickling periods, one alternative is using the vacuum pickling method (Bampi et al., 2016). This technology has been considered in the last decades for both reducing the wet-pickling time and promoting a more homogeneous distribution of salt for different types of products, such as cheese (Hofmeister et al., 2005), fish (Corzo et al., 2006), cured ham (Barat et al., 2005), turkey and chicken meat (Deumier et al., 2003; Schmidt et al., 2008).
But vacuum impregnation has not been applied to pickle duck eggs. This study first used vacuum decompression technology to pickle duck eggs. Compared with the traditional method, vacuum decompression technology adopts automated production equipment with fixed production parameters which can ensure the production in the whole year and uniform quality of Zaodan. Besides, vacuum decompression technology realizes the recycling of material liquid and it can protect the environment from contamination. Last but not least, Zaodan processed by the application of vacuum technology could reduce the pickling time from 20 wk to about 9 wk and is associated with enormous economic benefits. There is no literature about the vacuum decompression technology used in Zaodan pickling and no studies on the physical, chemical properties and structural changes of the egg white of Zaodan pickled by vacuum decompression technology. Therefore, the objectives of the present work were to compare the physical and chemical properties in the egg white of Zaodan pickled by traditional method and vacuum decompression method, and investigate the structural changes of Zaodan pickled by vacuum decompression technology.
Materials and Methods
Fresh duck eggs weighing 70 to 80 g were purchased from a local market in Tianjin City. All cracked or thin-shelled eggs were dislodged. The duck eggs were cleaned and dried in the air.
Traditional methods: The egg shells of selected eggs were hit such that they cracked while keeping the egg membrane intact. The eggs were pickled in a mixture of Red Star Erguotou and yellow rice wine up to 25 vol (ethanol concentration) with 5% salt, 5% black sugar and 10% vinegar. The eggs were pickled at atmospheric pressure.
Vacuum decompression methods: The pickling was conducted using vacuum processing equipment (YA-900A) which was custom designed for our laboratory at a temperature of 25°C, vacuum of -0.08 MPa until the pickled eggs were ripe. During the pickling processes, the samples were analysed on different days.
Three eggs were manually broken. The egg white was carefully separated from the yolk, and collected in the beaker. For each sampling time, the egg white of each sample was gently blend and stored at 4°C for the subsequent experiments.
At different pickling times, the pH, moisture content and salt content of the Zaodan egg white samples pickled by vacuum decompression method were compared with samples pickled by traditional methods.
The determination of pH was in accordance with the method of Benjakul et al. (1997). The moisture content was determined by AOAC method (2000). The salt content was determined according to AOAC (2000). To measure the salt content, 1 g egg white sample was added to 20 mL AgNO3 (0.1 M) and 10 mL HNO3. The mixture was boiled gently on a hot plate until all solids except AgCl2 were dissolved. Flowing water was used to cool the mixture. Then, 5 mL of 5% ferric alum indicator (FeNH4 [SO4]2 · 12H2O) was added. The mixture was titrated with a standardised 0.1 N KSCN until the solution became permanently light brown. The percentage of salt was then calculated as follows:
Where V1 is the volume of AgNO3 (mL), N1 is the concentration of AgNO3 (M), V2 is the volume of KSCN (mL), N2 is the concentration of KSCN (M), and W is the weight of the sample (g).For different pickling times, the protein content of the egg white samples pickled by vacuum decompression method was measured using an automatic Kjeldahl apparatus (K9840, China).
Zaodan egg white pickled by vacuum decompression method from different pickling times was mixed carefully. Each sample (1.5 g) was added to a 10 mL sodium chloride solution (1%). The total free sulfhydryl (SH) concentration of the samples was measured according to the method of Ellman (Ellman, 1959). 0.1 mL of albumen solution, 2.9 mL of SDS (sodium dodecyl sulfate) (0.5%) and 20 µL of the DTNB reagent (4 mg of DTNB, 1 mL of 0.2 M Tris–HCl buffer, pH 8.0) were added. The reagent blank was replaced by the sample with 0.2 M sodium phosphate buffer, pH 8.0. The sample blank was prepared in the same way, except that 0.2 M Tris–HCl (pH 8.0) was used instead of the DTNB solution. The absorbance of the mixture was monitored at 412 nm using a spectrophotometer (UV-2550PC) from Shimadzu. The total free SH concentration of the egg samples was then calculated as follows:
To 0.2 mL of egg white solution, 1 mL 10 M urea, 20 μL mercaptoethanol were added. The mixture kept stationary for one hour at 25°C. The egg white protein was deposited by 12% trichloroacetic acid solution. The precipitate was centrifuged for 10 min at 5,000 r/min. The residuum was washed with 12% trichloroacetic acid solution and dissolved in 8 mL of SDS (0.5%) and 20 µL of the DTNB reagent. Finally, the absorbance was measured at 412 nm against a reagent blank using a spectrophotometer (Ji et al., 2013). The SS bonds of the egg samples were then calculated as follows:
Where A412 is the absorbance change corrected for the reagent blank at 412 nm, D is the dilution factor, C is the sample concentration (mg/mL), N1 is the total free SH concentration, and N2 is the reduction of total free SH concentration.Protein surface hydrophobicity was determined using the method of Benjakul et al. (2001), with 8-anilo-1-naphthalenesulfonic acid (ANS) as a probe. Duck egg white solutions (0.005 to 0.025%, w/w) were prepared by diluting a stock protein solution in 0.1 M sodium phosphate buffer (pH 7.0). Then, 4 mL of the prepared solutions was mixed with 20 µL of 8 mM ANS. The fluorescence intensity was measured using a RF-5301PC spectrofluorometer (Shimadzu, Kyoto, Japan) at excitation and emission wavelengths of 374 nm and 485 nm, respectively. Protein hydrophobicity was calculated from the initial slope of the plot of fluorescence intensity against protein concentration determined by the Biuret method (Robinson and Hogden, 1940) using linear regression analysis. The initial slope was referred to as surface hydrophobicity (S0ANS).
Each sample pickled by vacuum decompression method (approximately 2.0 g) was added into the attenuated total reflection unit (ATR Harrick crystal, ZeSe Prism) to obtain a film. The infrared spectra were measured with a VECTOR 22 instrument from Bruker, and the infrared spectra were collected between 2,000 and 650 cm-1 for 1 min with a resolution of 4 cm-1, a scan velocity of 2.5 kHz and 160 spectra recorded per sample. For the liquid samples, a water spectrum was first subtracted from the sample spectrum. The secondary structure of the proteins was analysed based on the amide I band found in the range of 1,700-1,600 cm-1. The FTIR data were pre-treated by applying Fourier self-deconvolution, second derivative, and band curve-fitting. The relative contents of the different secondary structures were determined according to the area under the curve after band curve-fitting (Wang et al., 2011).
SDS-PAGE was applied to prove the changes in the protein components of the egg white. After 1.0 g of the egg white was dissolved in 9 mL of 0.5 M NaCl, the suspensions were centrifuged at 10,000×g for 10 min. Then, 200 µL of the supernatant was mixed with 500 μL of sample buffer (1 M Tris–HCl, pH 6.8, containing 10% SDS, 20% glycerol, 10% β-ME and 0.3% bromophenol blue). Electrophoresis was conducted in accordance with the method of Laca et al. (2010), using a 3.5% stacking gel and 12% separating gel. The loading volume was 50 μL and a vertical gel electrophoresis (Omni PAGE [DYY-III], Beijing Liuyi Co., Ltd, China) unit was used to separate at 20 mA/plate. Coomassie blue R250 was used to stain the gels and 40% methanol and 10% acetic acid was used to destain.
Results and Discussion
Changes in physical and chemical properties of the Zaodan albumen were monitored during pickling under the condition of atmospheric pressure and vacuum decompression. Compared with traditional method, the effect of vacuum decompression technology on the pH, moisture and salt contents of Zaodan was noticeable (Figs. 1 to 3), and the changes were noticeable in the primary pickling period compared with the latter. As time prolonged, an increase in salt content with coincident decreases in pH and moisture content in the Zaodan egg white were observed whether under the condition of atmospheric pressure or vacuum decompression.
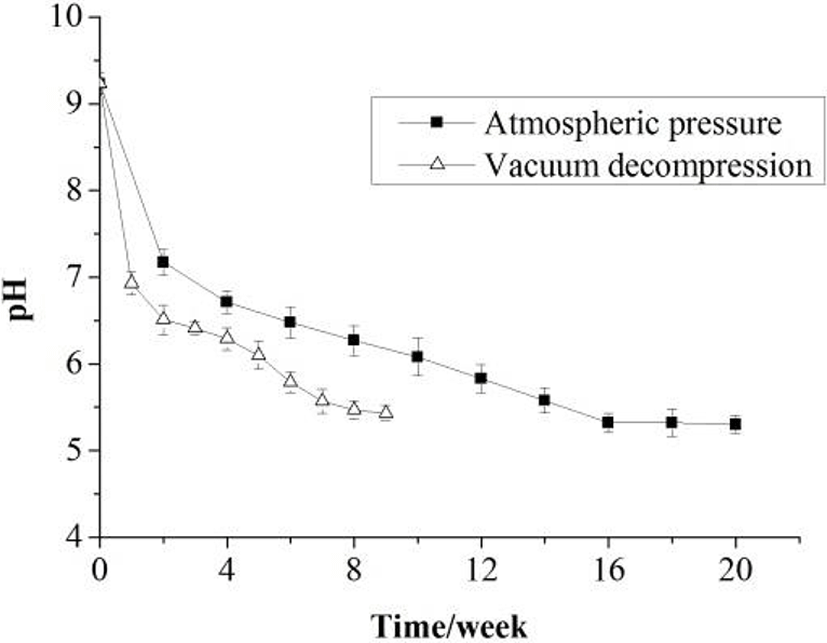
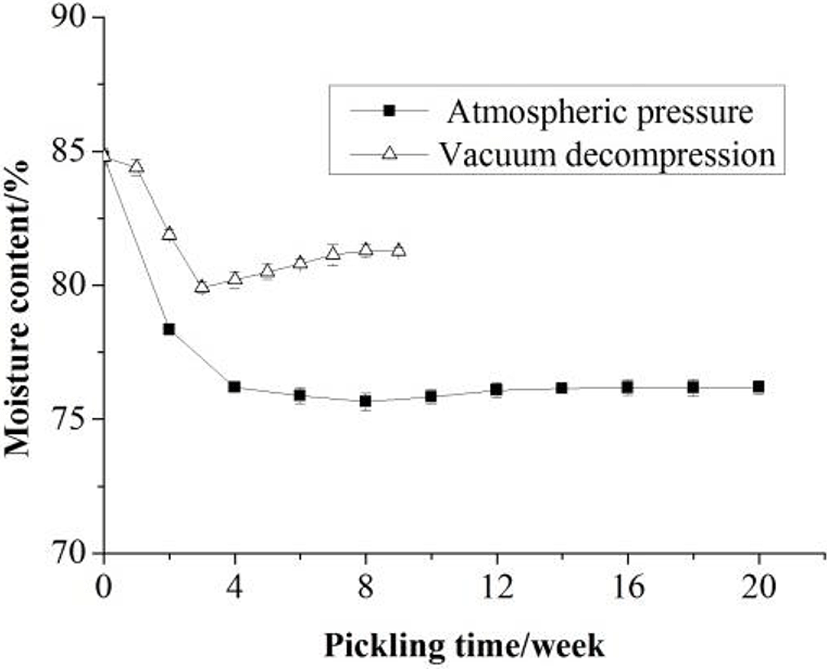
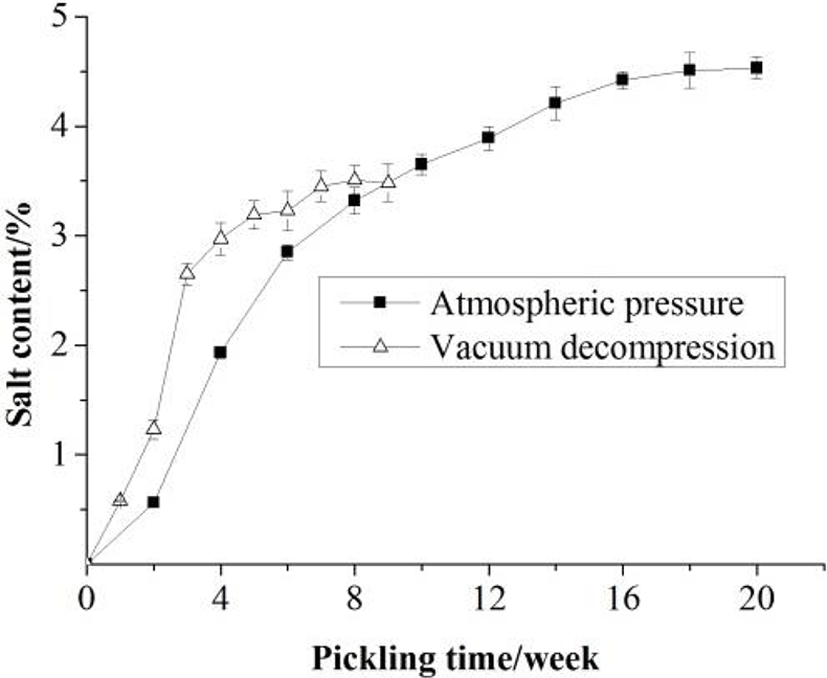
From Fig. 1, the pH of Zaodan egg white decreased, especially in the primary period. The pH of Zaodan pickled by traditional method was reduced from 9.23 to 5.3, and pickled by vacuum decompression method was reduced to 5.43. The pH of Zaodan egg white pickled by two methods had little difference. The reason of reduction in the pH was that part of the ethyl alcohol transformed into acetic acid due to oxidation, and acetic acid permeated from the pickling solution into Zaodan.
The moisture content of Zaodan pickled by traditional method was decreased from 84.8% to 76.19%, while pickled by vacuum decompression method was decreased to 81.27% (Fig. 2). The loss of water from the Zaodan egg white was most likely due to the greater migration of water from the egg yolk to the egg white and then to the environment through the egg shell via osmosis, as governed by pore size and structure of the shell (Kaewmanee et al., 2009). During the primary pickling period, the loss of water was greater than during any other period because of the greater difference in concentration.
At the end of Zaodan pickling, the salt content of Zaodan pickled by traditional method was 4.53%, while pickled by vacuum decompression method was 3.84%, the increase in the salt content indicated salt penetration (Fig. 3). Salt might be associated with the degeneration and aggregation of albumen proteins, leading to the formation of a gel-like structure (Ganasen and Benjakul, 2010).
The protein content in the Zaodan albumen pickled by vacuum decompression method was measured during pickling. With respect to the extension of the pickling time, the protein content in the albumen decreased slowly (Fig. 4). The protein content of the Zaodan egg white (8.25 g/100 g) was lower than that in fresh egg white (9.85 g/100 g). During pickling, the penetration of ethyl alcohol and acetic acid through the egg shell and membrane led to physical and chemical property changes and the gelation of egg proteins. Meanwhile, some proteins were also degraded into free amino acids (Meng et al., 2010).
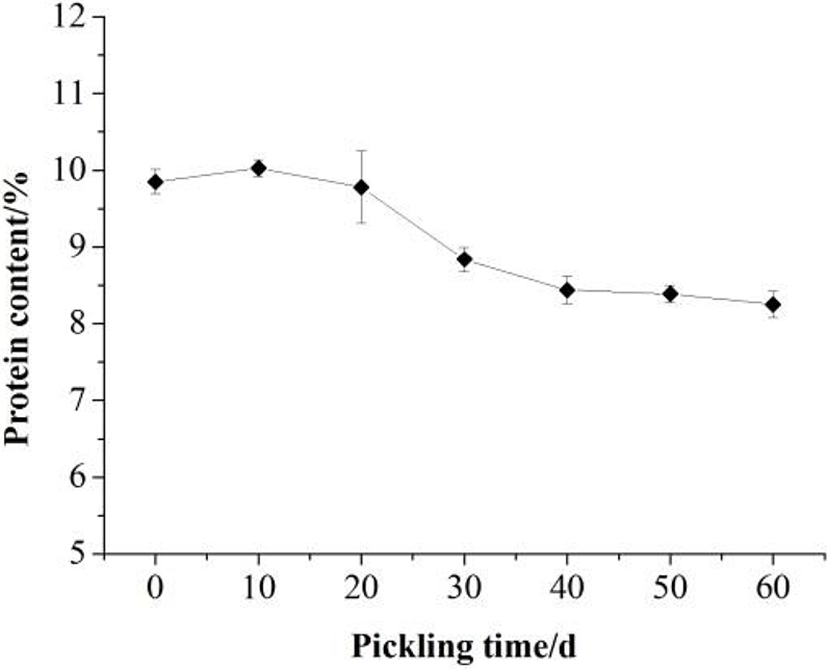
Changes in the total SH group content and SS bonds of the Zaodan pickled by vacuum decompression method egg white solution over the decompression time are shown in Fig. 5. The highest total SH group content (Fig. 5A) in the Zaodan egg white was 2.43×10-3 mol/g after pickling for 30 days, whereas the lowest content of SS bonds (Fig. 5B) was 23.15×10-3 mol/g.
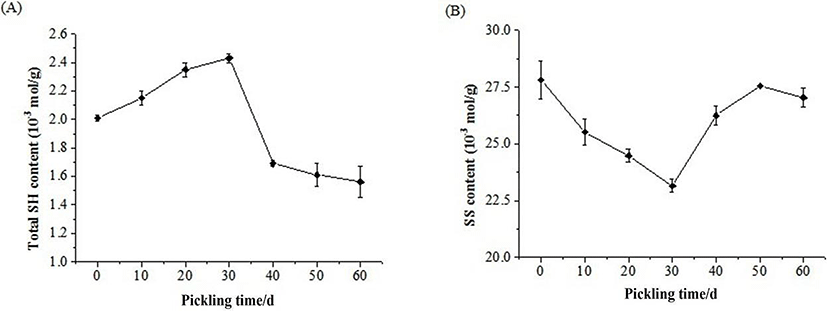
Before 30 days of pickling, the viscosity of the egg white decreased to a large extent, becoming watery. Ovalbumin contains four free SH groups, which are initially buried in the interior of protein (Mine, 1995). The cause of the increase in the SH group content during the initial phase was maybe due to damages to the natural structure of the albumen proteins. With the gradual penetration of ethyl alcohol and acetic acid, the natural structure of the protein slowly unfolded, and the secondary and tertiary structures were damaged. Most SH groups existed in the interior of protein are then exposed because of the damage to the protein structure. In addition, this process created some SH groups due to the disruption of SS bonds. SS bonds have a significant role in maintaining the conformation of protein and in SS-SH exchange. The changes in total SH group content were in agreement with the significant decrease in the disulphide content of the preserved eggs white (Fig. 5). After 30 days of pickling, egg white protein began to aggregate and solidify, SS bond had been formed, thus, the SH group decreased and SS bond increased. Other egg white fractions (ovotransferrin, ovomucoid and lysozyme) contain S-S bridges. An index of protein denaturation was the exposure of both SS and SH groups induced by the penetration of organic acids (Van der Plancken et al., 2005).
The surface hydrophobicity of the Zaodan pickled by vacuum decompression method egg white solution over time during pickling is depicted in Fig. 6. The surface hydrophobicity decreased from 181.65 to 36.02 and then increased to 160. The lowest point of surface hydrophobicity was observed at 30 d. The decrease in the surface hydrophobicity during the initial pickling phase was possibly caused by the interaction between different types of protein hydrophobic domains located at the surface. The increase during subsequent time points was most likely due to the exposure of hydrophobic proteins that occurred after denaturation as more and more acid penetrated the egg white (Wall, 1971). The level of protein unfolding can be monitored through the content of the surface hydrophobicity (Kaewmanee et al., 2011). Therefore, the protein fractions can be predicted to have been completely denatured. This study indicates that the low pH induced protein unfolding and the exposure of hydrophobic domains, facilitating the aggregation of proteins by reduced hydrophobic interactions during the pickling process.
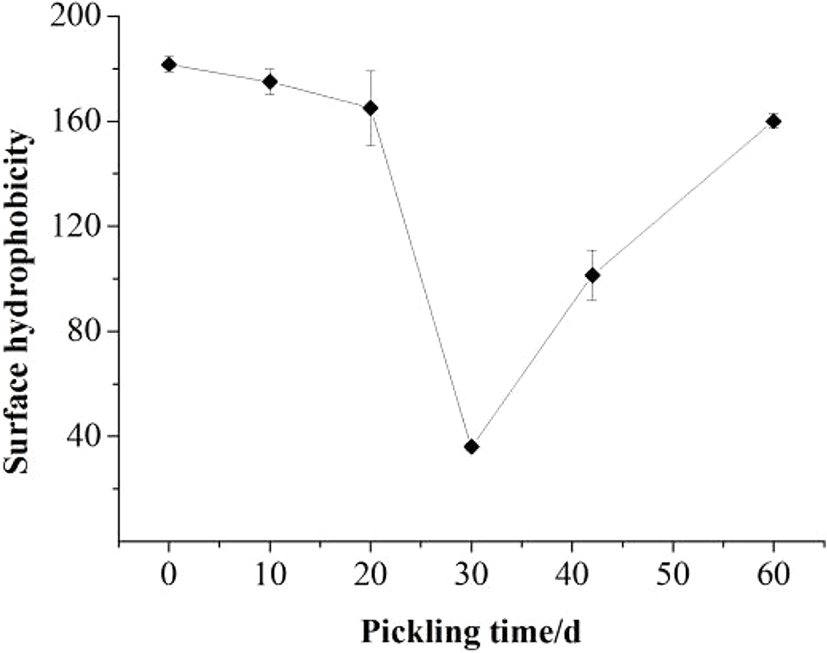
FTIR was used to demonstrate the changes in the secondary structure of Zaodan pickled by vacuum decompression method egg white proteins that occur during pickling. The amide I band (ranging from 1,700 to 1,600 cm-1) contains information that might reflect the protein secondary structure. The absorption bands observed in the amide I band correspond to β-turn (1,700-1,660 cm-1), α-helix (1,660-1,650 cm-1), and β-sheet (1,640-1,618 cm-1) structures (Brickley et al., 2007) and reflect changes in the egg white protein secondary structure during Zaodan pickling.
The FTIR spectra of egg white pickled for different days are shown in Fig. 7. The secondary structure analysis of the data is presented in Fig. 8. The quantitative analysis for the relative areas under the bands of the Gaussian curve-fit (GCF) result is given in Table 1. As the pickling time increased, the relative secondary structure content underwent a series of changes. The α-helix content increased from 21.72% to 29.53% at 20 d, and the random coil content increased from 11.35% to 28.40%. The β-sheet content decreased from 36.98% to 12.40%, and the β-turns changed little. These results indicate a structural shift from an ordered structure to random coils at 20 d. The changes during pickling were maybe due to the unfolding of the albumen proteins by the acidic conditions.
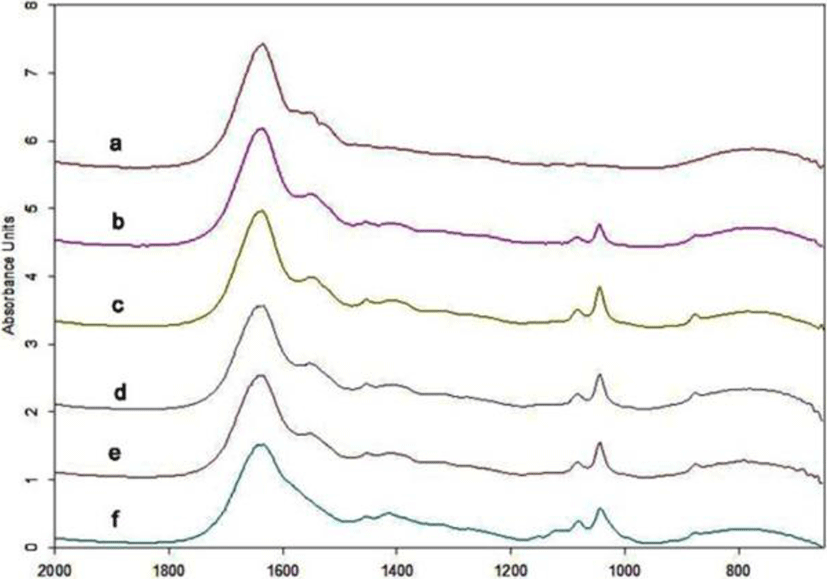
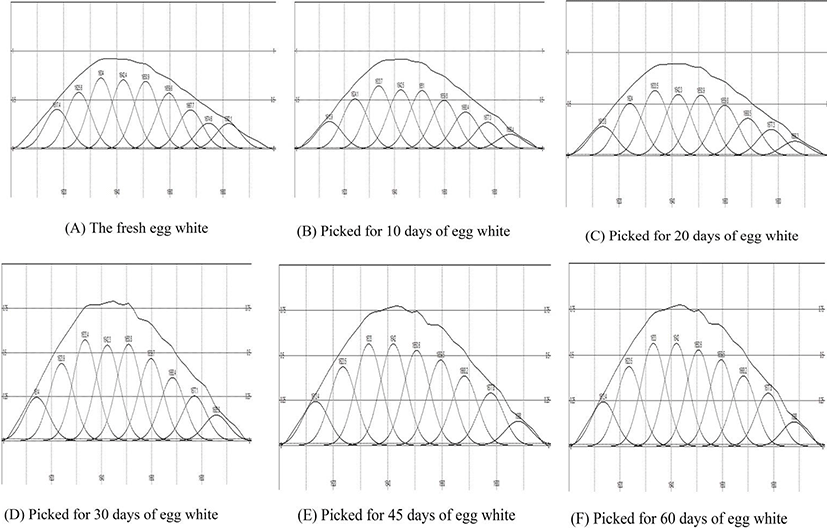
Zaodan samples pickled by vacuum decompression method for different days were prepared to analyse protein. The SDS–PAGE patterns of the Zaodan egg white are shown in Fig. 9. Fresh duck albumen contains five major proteins: ovomucin, ovotransferrin, avidin, ovalbumin and ovomucoid, with molecular masses of 110, 76, 68.3, 44.5 and 28 kDa, respectively. Ovalbumin is the most abundant protein in egg white (54%) (Kaewmanee et al., 2009). The electrophoresis results showed that fresh egg white contained ovomucin, ovotransferrin and ovalbumin bands and that degradation of ovomucin in the Zaodan egg white was pronounced at 10 d of pickling, with similar protein patterns observed for the other stages. The degeneration of the egg white mainly depended on the environment, such as pH, ionic strength and temperature (Sun and Hayakawa, 2001). The degeneration of the egg white proteins occurred along with protein aggregation after 10 d of pickling, suggesting that a low pH condition could lead to duck egg white denaturation and degradation when pickling eggs in an acidic solution for ages.
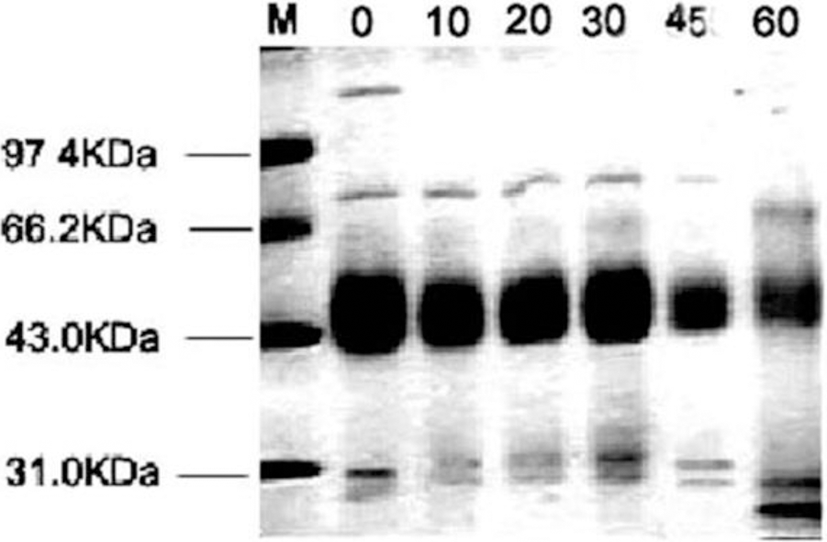
Conclusion
The study indicated that the physical, chemical properties and protein structure of Zaodan were effect by vacuum decompression technology. The decrease in the pH and the increase in the salt content indicated the migration of acetic acid from the pickling solution and salt penetration, respectively. The SH and SS groups were transformed into each other over different pickling times. The surface hydrophobicity and secondary structures of the egg white proteins were changed during the pickling time. Electrophoresis showed that ovomucin disappeared at the end of pickling.