Introduction
Animal blood was regarded as a food source in ancient civilizations. The blood is made up of 60% plasma and 40% blood cells, and its composition is similar among different species (Kuan et al., 2018). The term “liquid protein” is commonly used to describe blood because it is rich in high-quality protein (Ockerman and Hansen, 1999). Blood proteins are derived from whole blood or plasma and have high nutritional and functional qualities (Ofori and Hsieh, 2012). Plasma is the liquid fraction of the blood that remains after the removal of blood cells, and it is commonly employed in food processing due to its neutral flavor and lack of dark color influence on meals (Hsieh and Ofori, 2011). Therefore, one of the most significant biological by-products of animal slaughter is blood (Silva et al., 2003). In addition, it has been estimated that approximately 1,500,000 tons of porcine blood are generated annually in China alone, which is equal to the protein content of 2,500,000 tons of eggs or 2,000,000 tons of meat (Wang et al., 2007). If the animal blood is dumped as waste, valuable protein sources are lost.
Blood proteins are utilized in food industries as additives to modify the functional and nutritional aspects of meals (Parés et al., 2011), especially as binders but also as fat substitutes, emulsifiers, and natural color enhancers (Ofori and Hsieh, 2012). Blood proteins provide considerable nutritional, economic, and environmental benefits when used as food additives in food processing (Ofori and Hsieh, 2014). When bovine blood was used in sausages, it exhibited not only a significant reduction in fat but also increased protein and iron levels (Mathi, 2016). In addition, four antibacterial peptides were identified in bovine hemoglobin (Nedjar-Arroume et al., 2006). According to Rudolph (1999), farm animals including cattle and goats, have several significant abilities to produce pharmaceutical products. The drug marketed as Aimspro is produced from goat blood and can offer relief to sclerosis patients by improving their vision (Anaeto et al., 2010). In addition, goat blood serum contains 5.16 to 7.58 g of proteins and the glucose level is between 40 to 72 mg per 100 mL, which is critical for central nervous system function (Barakat and El‐Guindi, 1967). Therefore, the utilization of blood as a liquid protein from the slaughterhouse, offers nutritional, health, economic, and environmental benefits. However, only approximately 30% of slaughterhouse blood is used for its functional properties in the meat industry (Gatnau et al., 2001). The discarding of unused blood poses a severe environmental risk. The annual blood waste in a country such as the USA alone was 1.6 million tons with its 18% solid content and significant chemical oxygen demand (500,000 mg O2/L; Del Hoyo et al., 2007). Therefore, finding a technique to utilize blood not only solves environmental problems but also provides additional income to the meat industry (Ofori and Hsieh, 2014).
Animal blood, this protein source can be converted into bioactive peptide hydrolysates, which have the potential to be employed in the nutraceutical and pharmaceutical sectors (Bah et al., 2016b). Protein hydrolysis is a powerful tool for modifying the functional characteristics of proteins in food systems (Mune Mune, 2015). It involves the production of bioactive free amino acids or peptides from proteins that can be chemically or enzymatically produced (Tavano, 2013). The enzymatic process has positive effects on food processing, such as improving digestibility, modification of sensory quality, and health benefits including antioxidant capability or allergic ingredient decrease (Panyam and Kilara, 1996; Tavano, 2013). On one hand, enzyme hydrolysis produces shorter peptide chains than native proteins and thus it provides a viable alternative to solubilizing blood protein (Pérez-Gálvez et al., 2011). However, the hydrolysis conditions and proteases selection, such as enzyme-to-substrate ratio (E/S), pH, temperature, and hydrolysis duration, might affect the results of bioactive peptides (Bah et al., 2013; Liu et al., 2010).
Protein fragments known as bioactive peptides generally include 2–20 amino acid residues per molecule (Bhat et al., 2015) and have positive effects on bodily processes or circumstances, namely, the digestive, cardiovascular, nervous, and immune systems (Kitts and Weiler, 2003). It also possesses antimicrobial, antihypertensive, antioxidative, antithrombotic, opioid, cholesterol-lowering, mineral absorption or bioavailability enhancement, and immunomodulatory properties (Shimizu, 2004). Therefore, bioactive peptides produced from blood by-products may be used in the nutraceutical and pharmaceutical sectors, which would offer their financial, nutritional, and environmental advantages (Bah et al., 2013).
The discarding of slaughterhouse blood as waste is not only an environmentally serious problem but also results in losing valuable protein sources. Therefore, it is necessary to discover the functional characteristics of blood proteins for optimal utilization in the nutraceutical and pharmaceutical industries. Although several researchers have documented the potential of blood plasma activities, there have not been many studies on cattle and goat blood plasma. Therefore, this study approached to examine the functional qualities of blood plasma and its hydrolysates produced from black goats and Hanwoo cattle.
Materials and Methods
Slaughterhouse blood was collected from both Hanwoo cattle (Saesuncheon Livestock, Suncheon, Korea) and black goats (Gaon Livestock, Gangjin, Korea) by using the anticoagulant, ethylenediaminetetraacetate (EDTA), is used to collect it, and its most effective concentration of 1.2 mg/mL of blood. The following enzymes are acquired from Sigma-Aldrich (St. Louis, MO, USA): Protease from Bacillus licheniformis (Alcalase® 2.4 L; ≥2.4 U/g solutions; P4860), papain from papaya latex (≥10 U/mg protein; P4762), elastase from porcine pancreas (≥4.0 U/mg protein, E1250), thermolysin from Geobacillus stearothermophilus (30–350 units/mg, T7902) and α-chymotrypsin from bovine pancreas (≥40 U/mg protein; C4129). Other chemicals were purchased from Sigma-Aldrich as well as Daejung Chemicals (Siheung, Korea).
The blood plasma was separated from the cells by centrifuging the collected blood at 3,000×g for 20 min at 4°C. Then, the separated plasma was dialyzed to remove the EDTA, which was added during blood collection and lyophilized using a freeze dryer (Lyoph-Pride, LP03; ilShin BioBase, Dongducheon, Korea). Lyophilized blood plasma was re-dissolved at a 20 mg/mL concentration for hydrolysis. Following that, pH was adjusted to optimal conditions for each enzyme [protease from B. licheniformis (Bacillus protease) pH 6.5, α-chymotrypsin pH 7.6, papain pH 6.5, elastase pH 7.8, thermolysin pH 7.5] at room temperature. The samples were kept at their optimal temperatures for 24 h incubation (Bacillus protease at 55°C, papain at 37°C, α-chymotrypsin at 37°C, thermolysin at 37°C, and elastase at 25°C) with an enzyme to substrate ratio of 1:100. Incubation was stopped at 0, 3, 6, 9, 12, and 24 h by heat inactivation in a water bath at 100°C for 15 min. Fifteen percent (15 %) of sodium dodecyl sulfate polyacrylamide gel electrophoresis (SDS-PAGE) gel electrophoresis was used to assess the degree of hydrolysis. The most suitable hydrolysis conditions were obtained by observing the SDS-PAGE images. All treatments were performed in triplicate.
Functional characteristics were assessed in vitro using selected hydrolysis methods. Accordingly, the antioxidant capacity [thiobarbituric acid reactive substances (TBARS) and diphenyl picrylhydrazyl (DPPH) assays], metal chelation ability (Fe-chelation and Cu-chelation), and ACE inhibitory activity were measured. Without applying any additional treatments, analyses were carried out on hydrolysates made from solutions containing 20 mg/mL of blood plasma protein.
Antioxidant activity was evaluated using the approach reported by Abeyrathne et al. (2014) with a few adjustments. A polytron homogenizer (D-500, Scilogex, Rocky Hill, NJ, USA) was used to homogenize 1 g of pure refined soyabean oil (Sajo, Seoul, Korea), tween-20 (100 μL), and distilled water (100 mL) at the highest speed for 2 min to make an oil-in-water emulsion. After mixing 8 mL oil-in-water emulsion, 0.5 mL of distilled water, 0.5 mL of 200 ppm FeSO4, and 1 mL of blood plasma hydrolysates, the mixture was incubated at 37°C for 16 h. Then, 50 μL of 10% butylated hydroxyanisole in 90% ethanol and 2 mL of TBA/TCA (20 mM 2-thiobarbituric acid/15% trichloroacetic acid) solution were added to 1 mL of the incubated sample in the 15 mL centrifuge tube. This solution was vortexed and incubated in a water bath at 90°C for 15 min before being centrifuged at 3,000×g for 15 min. A UV-visible spectrophotometer (Selecta s.a., Spain) was used to measure the mixture’s absorbance at 532 nm in comparison to a blank made with 1 mL distilled water and 2 mL TBA/TCA solution. The malondialdehyde level was calculated using a standard curve and represented as milligrams of malondialdehyde per liter (MDA mg/L) of the emulsion.
The antioxidant capacity of blood plasma hydrolysates was evaluated using the DPPH scavenging test, which was slightly modified from the technique reported by Blois (1958). Briefly, distilled water (18 mL) was added to the 2 mL of test samples and homogenized. The mixed solutions were centrifuged at 3,000×g for 10 min. After mixing 0.4 mL of supernatant with 2 mL of DPPH (0.2 mM in methanol) and distilled water (1.6 mL), the mixture was stored in dark for 1 h. The mixture’s absorbance was examined at 517 nm.
The ferrozine method of Carter (1971) was used to determine the Fe-chelating activity of the test samples. In a 15 mL centrifuge tube, the blood plasma hydrolysates (100 μL) were mixed with 1 mL of 10 ppm Fe2+ (Fe2SO4) and 0.9 mL of distilled water and incubated at room temperature for 5 min. The mixture was added to 11.3% trichloroacetic acid (900 μL) before being centrifuged at 2,500×g for 10 min. The supernatant (1 mL), ferroin color indicator (200 μL), distilled water (1 mL), and 10% ammonium acetate (800 μL) were vortex-mixed in the test tube. After being incubated for 5 min at room temperature, the mixture’s absorbance was measured at 562 nm. The following question was used to calculate the iron-chelating activity.
The Cu chelation ability of blood plasma hydrolysates was evaluated according to Kong and Xiong (2006) with a few adjustments. The blood plasma hydrolysates (1 mL) were mixed with 1 mL of 0.2 mM CuSO4 in a centrifuge tube before being incubated at a standard temperature for 5 min. The mixture was then treated with 1 mL of 11.3% trichloroacetic acid (TCA) solution before centrifugation at 2,500×g for 10 min. Then, the supernatant (2 mL) was mixed with 1 mL of 10% pyridine and 20 μL of 0.1% pyrocatechol violet (Sigma-Aldrich) in a test tube before being incubated at room temperature for 5 min. The supernatant absorbance of the test samples was examined at 632 nm after centrifuging them at 2,500×g for 10 min.
The ACE inhibitory activity of blood plasma was determined by the technique of Miguel et al. (2007) and Yu et al. (2012) with a few adjustments. A 100 μL aliquot of the test hydrolysates was combined with 100 μL of 0.1 M borate buffer (pH 8.3) mixing 5 mM Hippuryl-Histidil-Leucine, 20 μL of ACE (0.1 U), and 0.3 M sodium chloride before incubation at 37°C for 30 min. The reaction was halted after incubation by adding 150 μL of 1 M hydrochloric acid. The produced hippuric acid was extracted using 1,000 μL of ethyl acetate before being centrifuged for 10 min at 1,500×g. An organic solvent was used to evaporate the organic phase (750 μL), which was then transferred to a test tube. The dried material was vortex-mixed with 800 μL of distilled water and the absorbance was examined at 228 nm in comparison to a blank produced with distilled water (100 μL) in place of the sample.
Results and Discussion
The activity of proteolytic enzymes degrades proteins into target-specific peptide cleavage bonds at appropriate temperatures and pH (Tapal and Tiku, 2019). In this investigation, Hanwoo cattle and black goat plasma were hydrolyzed using five different types of enzymes at optimum temperature and pH for 0, 3, 6, 9, 12, and 24 h: Papain from papaya latex, protease from B. licheniformis (Bacillus protease), α-chymotrypsin from bovine pancreas, elastase from porcine pancreas, and thermolysin from G. stearothermophilus. However, papain, α-chymotrypsin, and Elastase did not hydrolyze plasma proteins efficiently at their optimum pH and temperature conditions, even up to 24 h (data not shown).
The fifteen percent (15%) Tris-glycine SDS-PAGE patterns of hydrolyzed Hanwoo cattle and black goat blood plasma are shown in Figs. 1 and 2. According to visual investigations, the Bacillus protease almost completely hydrolyzed the plasma proteins into peptides with lower molecular weights less than 75 kDa after 3 h of incubation (presented with the box in Fig. 1). Abeyrathne et al. (2014) pointed out the 15% SDS-PAGE gel did not retain peptides with extremely low molecular weight.
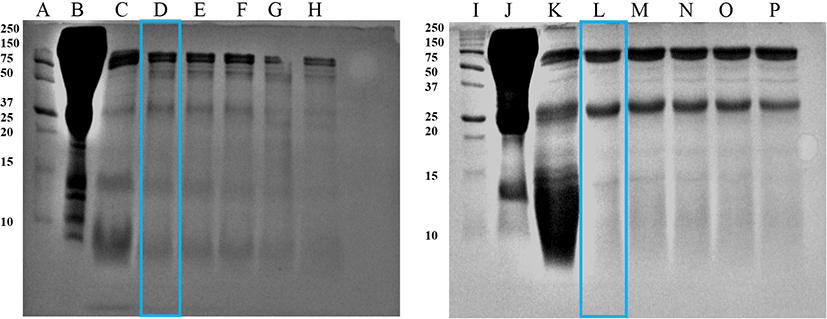
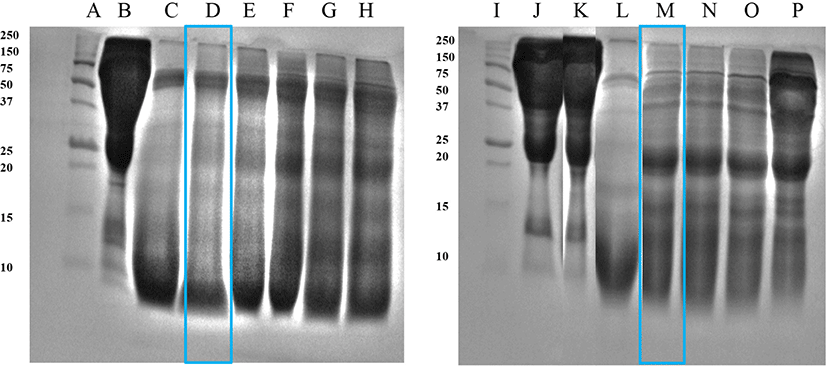
In a previous study, bovine blood plasma hydrolyzed with fungal protease produced more bioactive peptides after 4 h of incubation, almost threefold more than the plant protease hydrolysate after 24 h (Bah et al., 2016b). Hyun and Shin (2000) also observed that hydrolyzing bovine plasma protein with alcalase exhibited the highest peptide yield, and pepsin could also hydrolyze moderately, but neutrase and papain hydrolysis could not hydrolyze the proteins efficiently. Therefore, the specific application properties of the hydrolysate product must be considered when choosing enzymes and hydrolysis conditions (Lahl and Braun, 1994).
Fig. 2 shows the hydrolysate products obtained after hydrolysis using thermolysin. After 3 h of incubation, cattle plasma protein was hydrolyzed, but it was not termed as a complete hydrolysate since a smear appeared at approximately 50 kDa. Goat blood plasma was hydrolyzed after 6 h of incubation. Its hydrolysates displayed increased precipitation and turbidity, as well as the formation of a smear between the 25–10 kDa band.
Wei and Chiang (2008) affirmed that porcine red blood corpuscles in a membrane reactor may generate smaller bioactive peptides for health-promoting products using a variety of proteases including thermolysin. However, excessive protein hydrolysis must be avoided under conditions that can cause unfavorable effects including the production of bitter-flavored peptides (Jung et al., 2005).
According to the enzyme treatments, the hydrolysates from Hanwoo cattle and black goat plasma [cattle plasma hydrolysate by Bacillus protease 3 h 55°C (CP), goat plasma hydrolysate by Bacillus protease 3 h 55°C (GP), cattle plasma hydrolysate by Thermolysin 3 h 37°C (CT), and goat plasma hydrolysate by Thermolysin 6 h 37°C (GT)] were chosen and utilized as excellent for the analysis of functional qualities.
Antioxidants are substances that can delay or inhibit the oxidation process. It is particularly to prevent the harmful effects of free radicals in the human body, as well as the deterioration of fats in meals (Molyneux, 2004). Recently, antioxidants have received increased attention due to their relation to cancer prophylaxis, longevity, and therapy (Kalcher et al., 2009). TBARS is a common technique for assessing lipid oxidation. MDA is produced by polyunsaturated fatty acid peroxidation and forms an adduct with 2-TBA molecules, resulting in a pink color (Dasgupta and Klein, 2014). The lipid oxidation of Hanwoo cattle and black goat plasma hydrolyzed with Bacillus protease and thermolysin, as determined by TBARS values are presented in Fig. 3. Overall results presented that only intact goat blood plasma (G) had a lower TBARS value than the control (oil emulsion), indicating antioxidant activity. The intact cattle plasma and other hydrolysates exhibited weak antioxidant properties. Protein hydrolysates’ antioxidant activity is determined by their amino acid composition, which changes with enzyme activity, hydrolysis process, and enzyme-substrate ratio (Shahidi and Zhong, 2008). Liu et al. (2010) indicated that the inhibition of TBARS formation was more pronounced with an increase in the hydrolysis degree. When porcine plasma protein was digested with alcalase at 55°C for varied incubation times, the maximum antioxidant activity was found after 4 h of incubation (Chang et al., 2007). According to the TBARS value, the ability of intact goat plasma is capable to control and completely protect lipid oxidation against reactive oxygen species. Albumin, a prominent protein in the blood plasma, is thought to be high in goat plasma, which is known to be a major circulating extracellular antioxidant (Halliwell, 1988; Roche et al., 2008) and could contribute to the antioxidant activity identified. However, cattle plasma and the other hydrolysates required optimal hydrolysis conditions for antioxidant activity because there could have been a large number of poorly soluble, undigested proteins. When assessing a hydrolysate’s in vitro functional characteristics, solubility is a crucial consideration (Kim and Yoon, 2020).
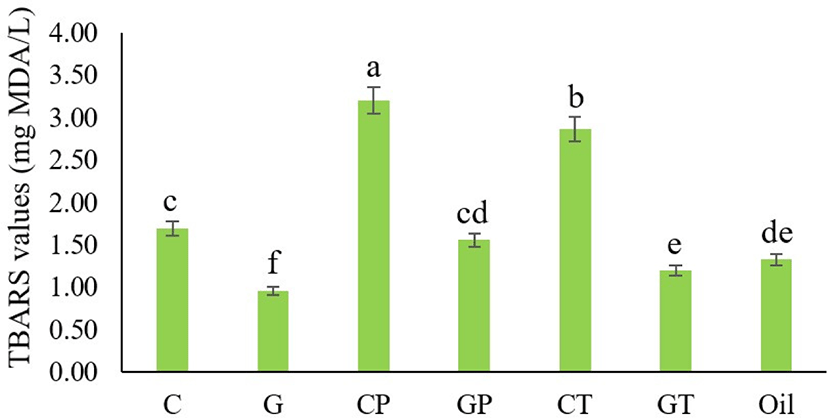
The antioxidant capacity of blood plasma from black goat and Hanwoo cattle was determined based on its DPPH radical-scavenging activity (Fig. 4). When DPPH radicals encounter a substance that donates a proton, such as an antioxidant, the radical is scavenged by changing its color from purple to yellow, and its absorbance value is decreased (Zhang et al., 2008). The present studying indicated that the DPPH radical scavenging activity of non-hydrolyzed cattle and goat plasma had free radical scavenging activity (20.25±3.12% and 17.64±2.55%) respectively. In addition, this study found that both Hanwoo cattle and black goat plasma hydrolysates showed very limited radical-scavenging activity. Bah et al. (2016a) also reported that the strong DPPH radical scavenging activity (21.3±1.5%) was obtained from the intact cattle blood plasma protein. Frei et al. (1988) also assessed that the blood proteins can offer 10%–50% of the peroxyl radical scavenging activity of plasma. In addition, Seo et al. (2015) mentioned that although bovine plasma was hydrolyzed with alcalase in different hydrolysis processes, the maximum DPPH radical scavenging activity was obtained only at 51.66°C in 7 h (hydrolysis condition). The cattle plasma treated with fungal protease had higher values of soluble peptides that contributed to antioxidant activity (Bah et al., 2016b). Therefore, both non-hydrolyzed Hanwoo cattle and black goat plasma proteins possess free radical scavengers. This may be due to enzymatic antioxidant systems in the blood plasma, which can be attributed to scavenging activities such as superoxide dismutase, catalase, and glutathione peroxidase (Wang et al., 2018), which constitute the main system for preventing oxidative cell damage. However, the hydrolysates produced from the current proteases and hydrolysis conditions were not effective in DPPH scavenging activity.
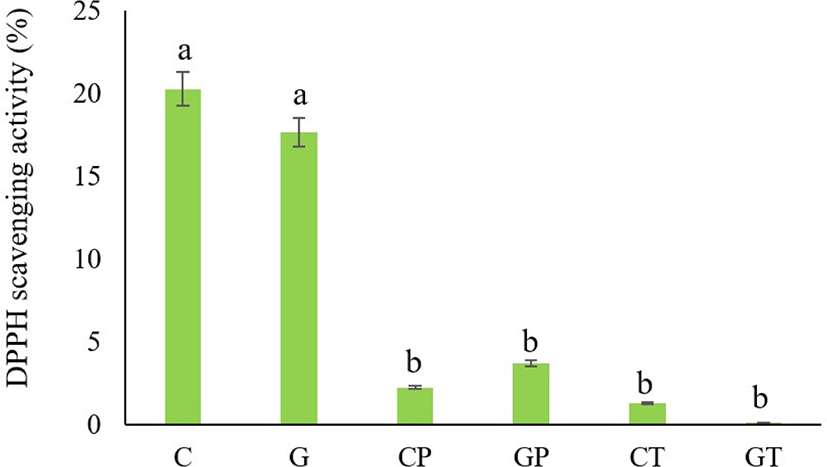
Transition metal ions, especially Fe and Cu can catalyze the generation of reactive oxygen species primarily superoxide anions (O2–) and hydroxyl radicals (oOH; Repetto et al., 2012); thus, the chelation of metal ions contributes to antioxidation and subsequently prevents food rancidity (Zhang et al., 2010). The iron-chelating activities of Hanwoo cattle and black goat plasma are shown in Fig. 5. The iron chelating activities increased after proteolytic degradation except for Bacillus protease-treated cattle blood plasma. The black goat plasma hydrolysates treated with Bacillus protease (GP) had the strongest iron-chelating effect (11.26±2.05). In previous studies, when bovine blood plasma was hydrolyzed with alcalase in different hydrolysis processes, the maximum iron-chelating point was obtained after 6 h of incubation at a temperature of 55.34°C (Seo et al., 2015). The capacity of the protein hydrolysates to chelate metals depends on the amino acid residues that are acidic (Glu and Asp) and basic (Arg and Lys; Rajapakse et al., 2005). Furthermore, it is likely that the size and sequence of amino acids in peptides affect the ability of protein hydrolysates to function as antioxidants (Chen et al., 1998). However, hydrolysis conditions and enzyme activity can change amino acid composition, implying that the chelating activities of various hydrolysates may differ. In the present study, the intact cattle blood plasma (C) and its hydrolysates produced from Bacillus protease (CP) could not detect the iron chelating activity even after hydrolysis. This is possibly due to enzyme activity destroying the metal-binding sites.
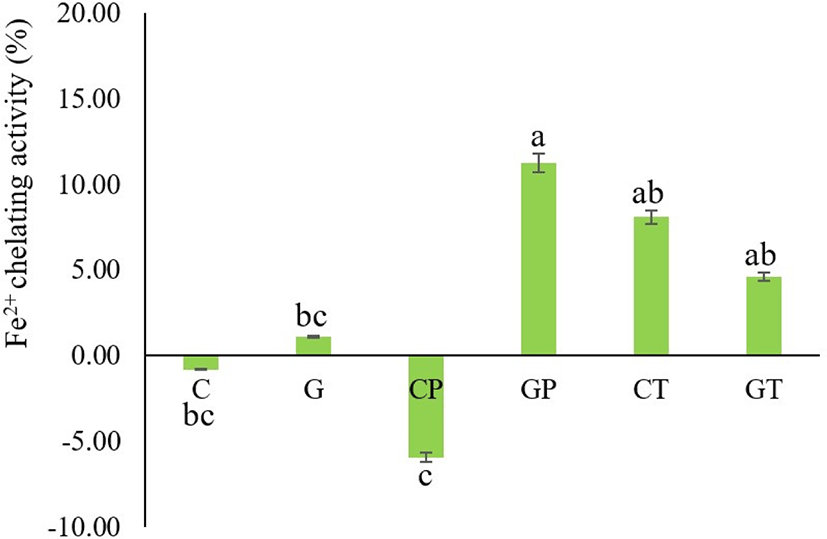
In hydroperoxide breakdown processes, Cu is a more potent catalyst than Fe (Halliwell and Gutteridge, 1990). The copper chelating activities of all tested samples were excellent, except for the non-hydrolyzed cattle plasma (Fig. 6). Among six treatments, G and GT had the strongest copper chelating power (95.83±0.51% and 96.76±0.51%) respectively. Dong et al. (2008) indicated that the chelation ability of hydrolysates increased with longer hydrolysis time, which agreed with the result of GT (longer hydrolysis time). The copper chelating activity of alcalase hydrolyzed porcine blood plasma protein increases significantly with an increasing degree of hydrolysis (Liu et al., 2010). In this investigation, both hydrolysates strongly suppressed lipid oxidation by copper chelating activity at a concentration of 20 mg/mL. It is thought that peptide cleavage in this investigation led to enhanced copper ion binding, thus removing prooxidative free metal ions from the hydroxyl radical system. Therefore, intact goat plasma and all hydrolysates from the current study can be used as copper-chelating agents.
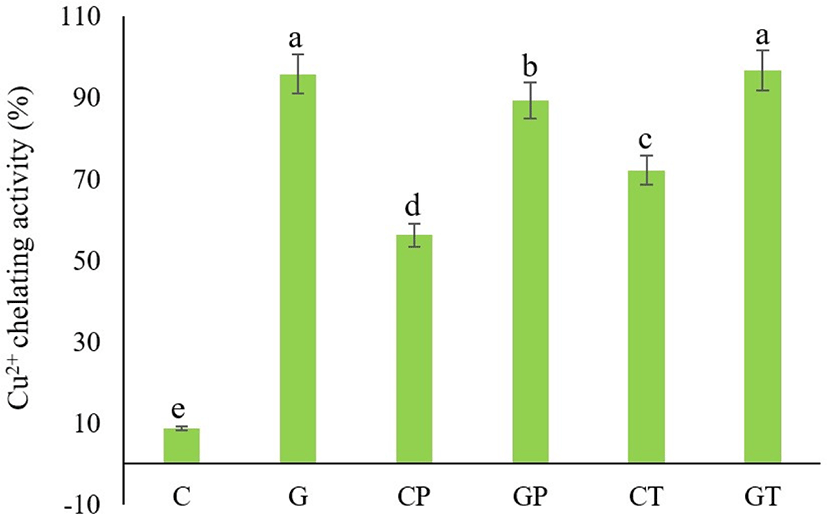
Regarding metal chelating activities, non-hydrolyzed goat blood plasma protein had stronger chelating power than non-hydrolyzed Hanwoo cattle blood plasma. This is assumed to be due to the specific biological functions of black goat plasma proteins. Most biological systems contain proteins, which have the biological function of binding, storing, or transporting catalytically inactive metals (e.g., Transferrin: Iron activity is controlled by binding iron in its less active ferric form and sterically preventing metal-peroxide interactions; Elias et al., 2008).
ACE converts angiotensin I into the angiotensin II active form in the blood and deactivates bradykinin to extend the blood vessels (Li et al., 2004), which contributes to blood pressure elevation. Therefore, ACE inhibitors need to repress the catalytic action of ACE to lower blood pressure and achieve an antihypertensive effect (Arihara and Ohata, 2008). The ACE-inhibitory activity of porcine blood plasma hydrolyzed with the single enzyme trypsin was mild, but the ACE-inhibitory activity of porcine blood plasma hydrolyzed with multiple enzymes was maximum (Wei and Chiang, 2008). Our results indicated that the non-hydrolyzed goat plasma (G) and its hydrolysates produced from thermolysin (GT) possessed the highest ACE-inhibitory activity (9.86±3.7% and 21.77±3.74%) respectively (Fig. 7). The original cattle blood plasma (C), its hydrolysates (CP and CT), and goat blood plasma treated with Bacillus protease (GP) did not show the ACE inhibitory activity. Among the different ACE inhibitory peptides that have structure-activity correlations, C-terminal tripeptides strongly influence binding to the ACE (Li et al., 2004). In addition, peptides possessing hydrophobic (branched-side chain and aromatic) amino acid residues at each of the three C-terminal positions have substantial ACE inhibitory action, which is characteristic of ACE inhibitory peptides (Hanafi et al., 2018). If the hydrophilic characteristic is high, the inhibitory activity is generally low or no activity is observed because the peptide is rendered inaccessible to the active site of ACE (Li et al., 2004). Therefore, peptides should contain hydrophobic amino acids for optimal inhibitory activity. The hydrophobicity also varies in each hydrolysate, depending on the hydrolysis conditions. Luo et al. (2014) reported that hydrolysates prepared under different hydrolysis conditions exhibited different hydrophobicities. In our results, G and GT showed ACE-inhibitory activity but cattle blood plasma (C), its hydrolysates (CP and CT), and goat blood plasma treated with Bacillus protease (GP) showed no inhibitory activity. It is assumed that a suitable hydrolysis process is required to yield optimal ACE inhibitory activity.
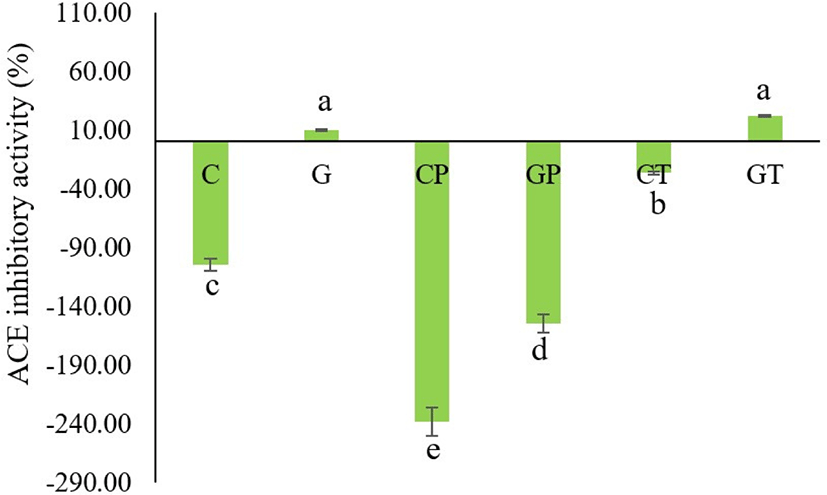
Conclusion
Under the test conditions, the plasma proteins of Hanwoo cattle and black goats were almost completely hydrolyzed using protease from B. licheniformis and thermolysin. The black goat blood plasma and its hydrolysates are good sources to produce bioactive peptides with functional properties. In particular, even goat plasma that has not been hydrolyzed exhibits good functional characteristics like antioxidant activity, metal chelating activity, and ACE inhibitory activity. Therefore, it can be used to improve antioxidant activity in food processing and reduce hypertensive people’s blood pressure in the future. However, Hanwoo cattle blood plasma and its hydrolysates have relatively poorer functional characteristics. Some hydrolysis conditions could negatively affect the functional qualities of these hydrolysates. The best functional features of blood plasma proteins can only be obtained through further investigation using an appropriate hydrolysis procedure, enzymes, and the enzyme-substrate ratio. It is essential to analyze the various hydrolysate concentrations and identify the functional properties based on the identification of peptides.