Introduction
Ricotta represents a typical soft Italian cheese, mainly obtained by ewes or goat milk, and less frequently by exploiting cow and buffalo milk (Mucchetti et al., 2002). Ricotta is specifically obtained by using the cheese whey deriving from breakage of the curd during cheese-making. The production process involves the direct acidification of the whey which increases the protein coagulation, the product that emerge on the surface is collected in plastic baskets characterized by small openings that allow the drainage of the liquid phase. The demand of consumers of traditional, unprocessed, and high nutritional dairy products has recently been increasing and Ricotta responds well to consumer requests because it is a fresh product, characterized by a low percentage of fat and by a high proteins content. Dietary, genetic, milking and technological factors influence the milk composition and, consequently, the quality of cheese. Although Ricotta is a very widespread whey cheese, the literature on this product is scarce and outdated.
Vinery by-products play an important role in the small ruminant diets (Alba et al., 2019; Correddu et al., 2020). Grape pomace (GP) is the main solid by-product of the wine industry consisting of peel, pulp, and seeds. Its storage and its disposal generate a lot of environmental and economic problems.
Red GP is a matrix rich in compounds with high biological value especially polyphenols (2%–6.5%), as simple flavonoids, phenolic acids, tannins and proanthocyanidins (Yu and Ahmedna, 2013). However, the high levels of lignified fibre, tannins and anthocyanins represent a limitation for use of GP in the diet of the ruminant, since these compounds could negatively the digestive nutrient utilization (McSweeney et al., 2001; Min et al., 2002). In contrast, Moate et al. (2014) have reported that tannins, due to low rumen biodegradability, can induce an increase in the small intestine protein intake, improving rumen metabolism and decreasing methanogenesis.
Furthermore, a lot of studies have shown that the presence in winery waste of a high content of linoleic and oleic acids and phenolic compounds can have beneficial effects on animal health and consequently on the quality of products of animal origin. It has been reported that antioxidant compounds in GP, as flavonoids, can be directly transferred to the milk or after metabolic transformation by rumen microbes (Correddu et al., 2015), causing an enrichment of the milk with substances that have health benefits for its consumers.
In the present study, the hypothesis to improve the nutraceutical and quality characteristics of milk-derived products has been assayed, supplementing the diet of lactating ewes with a GP supplementation. The presence of phenolic compounds and their effects on chemical-nutritional composition of whey and Ricotta cheeses were investigated.
Materials and Methods
Fourty-six Assaf ewes, homogeneous for days in lactation and age, were involved in the study and randomly assigned into two groups of twenty-three ewes each: A control group (CTRL) and experimental group (GP+) whose diet was enriched with 10% of red GP. Overall, the trial lasted 60 d and during this time interval all animals received isoenergetic and isoproteic diets that were prepared by taking into account the nutritional needs of lactating sheeps.
At the end of the trial, 80 L of milk were collected for each group of ewes. The whey obtained from the curd breakage during cheese-making was recovered and used to make Ricotta following the procedure described by Innosa et al. (2020). For both groups, 6 Ricotta cheeses of about 450–500 g were produced for each group, with a yield production equal to 8%–9% and. For each group, samples of whey before (WBR) and post (WPR) Ricotta cheese-making were collected. Three Ricotta cheeses were sampled and aliquoted after 1 d (T1) from production, while the remaining three were left at +4°C for 5 d (T5) and then subjected to the sampling. All the collected samples were packed under vaacum and stored at −20°C until the analysis.
The moisture of T1 and T5 Ricotta cheeses was determined according to the AOAC methods (2000). Evaluation of total lipids in Ricotta, WBR, and WPR was performed by following the procedure reported by Innosa et al. (2020), and the amount of total fat was expressed as mean percentage on a dry matter (DM) basis.
A CR-5 colorimeter (Minolta, New York, NY, USA) was used to evaluate the color of Ricotta cheese and whey, by calculating the chromatic coordinates L* (lightness), a* (redness) and b* (yellowness). The optical system exploited an aperture size that was adjusted to 3 mm, and each measurement was performed in reflectance by placing the sample into a glass petri dish (33 mm). On the contrary, WBR and WPR measurements were performed in transmittance, the samples were placed into a glass rectangular cell with optical path of 2 mm. From the already measured parameters were calculated: The total difference of color (ΔE*ab) and the Yellow Index (YI) by using the formulas listed below:
Final ΔE*ab values were compared with arrange by Nedomová et al. (2017).
Total phenolic compounds (TPCs) of WBR, WPR and Ricotta T1 and T5 were spectrophotometrically evaluated at 765 nm by using the method described by Singleton and Rossi (1965). Fifteen milliliters of a solution composed by methanol and water (70:30, v/v) was added to 5 mL of whey and/or to 1 g of Ricotta. The mixture was shaked for 30 s, incubated at room temperature and in the dark for 40 min and centrifuged (15 min, 4,000×g), then the supernatant was removed for analysis. The calibration curve was prepared by using the gallic acid (1–100 μg/mL, r2=0.994) and results were expressed as μg equivalent of gallic acid (GAE) per mL of whey and μg equivalent of GAE per g of Ricotta.
With regard to the antioxidant potential, such parameter was evaluated by the 2,2'-azino-bis (3-ethylbenzothiazoline-6-sulfonic acid) (ABTS) assay according to Brahmi et al. (2012). The calibration curve was built using Trolox (1–32 μmol/mL, r2=0.9991) and the antioxidant capacity of each sample was reported as μmol equivalent of Trolox (TEAC, Trolox Equivalent Antioxidant Capacity) per mL of whey and μmol equivalent of Trolox per g of Ricotta.
Seventy milligrams of the WBR and Ricotta T1 lipid extracted as reported in paragraph (Chemical analysis and color measurement of Ricotta and whey) were recovered in hexane in which were added 500 μL of sodium methoxide in methanol (1:1, v/v) in order to induce the fatty acids trans-methylation. Fatty acid methyl esters (FAME) were then separated according to Bennato et al. (2019). Peaks areas of each FAME identified were analyzed by using the ChromeCard Software and the values associated to each compound were expressed as relative percentage of total FA. The value of each FA was used to calculate the sum of monounsaturated fatty acids (MUFA), polyunsaturated fatty acids (PUFA), saturated fatty acids (SFA), short chain fatty acids (SCFA), medium chain fatty acids (MCFA) and long chain fatty acids (LCFA). Furthermore, Atherogenic (AI), Thrombogenic (TI) and Desaturation indices (DI) for C14:0, C16:0, C18:0, and CLA were calculated by using the formulas reported by Innosa et al. (2020).
Protein profile in WBR and Ricotta T1 and T5 was evaluated via SDS-PAGE, using the procedure reported by Laemmli (1970). For protein extraction, 10 g of Ricotta sample were dissolved in 10 mL of H2O and heated at 37°C for 15 min. Then, 1 mL of 5% (v/v) acetic acid and after 10 mins 1 mL of 1 N sodium acetate were added. The samples were filtered and to 1 mL of filtered 200 μL of 100% (w/v) trichloroacetic acid (TCA) were added. The samples were cooled at –20°C for 20 min and centrifuged at 4°C for 20 min at 12,000×g. Supernatant was removed, and the pellet was washed thrice with 1 mL of cold acetone at 12,000×g for 10 min. For whey protein extraction the same procedure used for Ricotta was followed even if 1 mL of whey was treated directly with 200 μL of 100% (w/v) TCA.
The proteins extracted were then quantified using Bradford method (Bradford, 1976) and separated on 12% SDS-PAGE gel as reported by Bennato et al. (2020).
Densitometric analysis of the visualized bands was then performed by exploiting the ImageJ software, and the content of proteins was expressed as relative percentage of the total protein.
The identification of volatile compounds (VOCs) in T1 and T5 Ricotta samples was achieved by making reference to the protocol previously described by Bennato et al. (2020) and based on a solid-phase microextraction (SPME) followed by a gas chromatography-mass spectrometry (GC-MS) analysis performed with a gas chromatograph (Perkin Elmer, Waltham, MA, USA) coupled with a mass spectrometer (SQ8S; Perkin Elmer, USA). Briefly, 3 g of Ricotta were transferred in vials in which there was the addition of 10 mL of a NaCl solution (360 g/L) and 10 μL of an internal standard (4-methyl-2-heptanone). The VOCs adsorption was performed with a divinybenzene–carboxen–polydimethylsiloxane SPME fiber (Supelco, Bellefonte, PA, USA) exposed for 1 h and at 60°C in the headspace. The extracted VOCs were thermally desorbed into the GC/MS and identified using Kovats retention index. The data concerning each compound were expressed as relative abundance on the sum of the total identified VOCs.
SigmaPlot 12.0 Software (Systat software, Chicago, IL, USA) was used for the statistical analysis of the obtained data. Student’s t-test was applied in order to identify significant differences between the two groups of data; p-values lower than 0.05 were considered statistically significant. Results were reported as mean±SD.
Results
As shown in Table 1, the diet did not affect WBR and WPR lipid content. Significant differences were observed in color parameters. The colorimetric analysis carried out on WBR showed a lower lightness (p<0.01) and a significant decrease (p<0.01) of CIE a* parameter (green-red) towards to a light green nuance. On the contrary, compared with the CTRL-WBR, the GP+–WBR showed higher (p<0.01) CIE b* parameter (blue-yellow) and YI (p<0.01). An opposite trend was observed in WPR. In agreement with the criteria of Nedomová et al. (2017), between GP+ and CTRL was observed a middle color difference (ΔE*ab) both for WBR and WPR.
Ricotta T1 made from whey obtained from the rennet breakage of GP+ cheese exhibited a higher DM percentage compared to CTRL (p<0.01) but not significant differences between the two groups were observed in lipid content (Table 2). The same trend was observed in Ricotta T5. With regard to the chromatic coordinates, lightness (CIE L*) and redness (CIE a*) were not affected by the diets, while yellowness (CIE b*) and YI were lower in Ricotta T1 deriving from the milk obtained from the GP+ (p<0.01). After 5 d of ripening, GP+ Ricotta samples showed higher values for lightness (p<0.05) and a* (p<0.05) compared to the CTRL samples.
Very light differences were observed both for Ricotta T1 and T5 between GP+ and CTRL.
The TPC amount in WBR obtained from GP+ was significantly lower (p<0.05) compared to the CTRL. On the contrary, no significant variations were observed for WPR in the same samples (Table 1). GP+ Ricotta T1 had a higher content of TPCs (p<0.01), after 5 d of ripening no significant differences were evidenced. Furthermore, no significant variations were observed for the antioxidant capacity in WBR, WPR and T1 and T5 Ricotta samples (Table 2).
The fatty acid profile of WBR and Ricotta T1 is reported in Table 3. No significant variations of SFA, MUFA, PUFA, SCFA, MCFA, and LCFA were observed in WBR and Ricotta T1. However, in whey samples lower levels of odd-chain fatty acids, as pentadecylic (C15:0, p<0.001) and margaric (C17:0; p<0.05) acids, stearic acid (C18:0, p<0.05), myristoleic acid (C14:1, p<0.001) and linolenic acid (C18:3, p<0.05), on the contrary higher levels of myristic acid (C14:0, p<0.05) and vaccenic acid (C18:1, t11; p<0.05) were observed. In Ricotta, lower levels were observed in C15:0 (p<0.01), C17:0 (p<0.05), C14:1 (p<0.001); on the contrary, higher level of C18:1, t11 (p<0.05) were observed in GP+ Ricotta samples. Furthermore, lower DI DIC14:0 and DICLA and higher desaturation index DIC18:0 was observed both in whey and Ricotta of GP+ group.
ns, not significant, CLA, conjugated linoleic acids, SFA, saturated fatty acid, MUFA, monounsaturated fatty acid, PUFA, polyunsaturated fatty acid, SCFA, short chain fatty acids (C4:0-C6:0), MCFA, medium chain fatty acids (C8:0-C15:0), LCFA, long chain fatty acids (C16:0-C18:3); DI, desaturation index; AI, atherogenic index, TI, thrombogenic index.
SDS-PAGE analysis was exploited in order to characterize the protein profile of whey and Ricotta (Fig. 1). WBR SDS-PAGE analysis showed the separation of the main whey proteins fraction (lactoferrin, serum albumin, β-lactoglobulin and α-lactalbumin) and less intensive bands corresponding to caseins residues. No significant differences in bands intensity were evidenced between the two groups (Table 4).
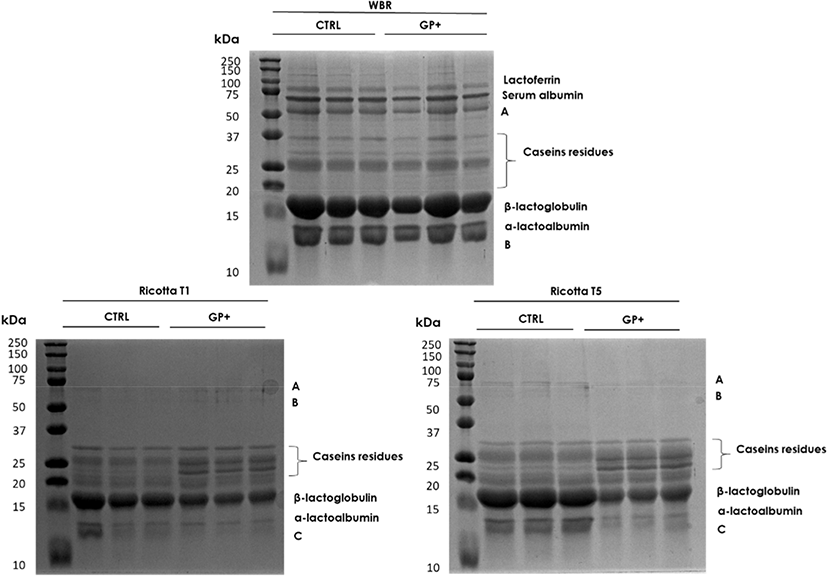
In GP+ Ricotta T1 and T5 samples, a higher intensity of caseins residues was highlighted, β-lactoglobulin band intensity although decrease during the ripening in both groups, a lower (p<0.05) band intensity degradation was observed in GP+ Ricotta T5 samples compared with CTRL. No significant variations were observed between the two groups in α-lactalbumin band intensity (Table 5).
Nine VOCs were identified both in T1 and T5 Ricotta samples, five aldhehydes, two ketones and two carboxylic acids (Table 6). In Ricotta T1, nonanal and octanoic acid were affected by the diet, resulting respectively lower (p<0.05) and higher (p<0.05) in GP+ samples in comparison to CTRL Ricotta. A lower (p<0.05) hexanal amount was highlighted in GP+ Ricotta after 5 d of ripening.
Discussion
The dietary supplementation with red GP did not influence the lipid content of whey and Ricotta, however the DM content resulted to be higher in GP+ Ricotta. Differences between the two groups in moisture content of Ricotta could be correlated to a different protein concentration. As highlighted by Salvatore et al. (2014), with a low protein content in the cheese whey, some challenges appear in gel formation, conversely, with increasing protein concentrations, the number of linkages increases during heating, resulting in a more compact protein gel characterized by an improved water-holding capacity.
Whey and Ricotta color could be influenced by several factors, as the diet used for animal feeding, technological applications as heating and acidification and chemical composition of whey, such as fat, protein, Ca, and P. In the present study variations of CIE L*, CIE a*, and CIE b* components were detected in both GP+–WBR and WPR. Generally, the whey has a straw yellow color due to presence of riboflavin (vitamin B2), however, a lot of pigments contained in animal feeding could influenced a* and b* parameters (Nozière et al., 2006; Schreiner and Windisch, 2006; Solah et al., 2007). Higher CIE b* values and lower CIE a* coordinate and consequently a higher YI in GP+ WBR could be correlate to a different pigment composition compared with CTRL. Most of pigments contained in animal feeding, belonging to polyphenols family have a different affinity for protein and lipids and a different susceptibility to increased temperature like those used for the Ricotta production (Nozière et al., 2006) and this could explain the different trend in CIE b* values and YI observed in GP+ samples of Ricotta compared with WBR. However, despite the variations in color parameters observed in whey and Ricotta samples according to the table of color difference reported by Nedomová et al. (2017), it is possible to state that the difference between the two groups was very light.
Regarding the phenolic compounds, it was possible to observe a lower amount in GP+ WBR and an opposite trend in Ricotta T1. As previously stated for the different color trend between whey and Ricotta, this apparent contradiction could be explained by a different polyphenols composition that transfer differently from whey to Ricotta. The different content of TPCs was not correlated to changes in the antioxidant capacity of WBR and Ricotta T1 evaluated by ABTS assay. Recently, it has been reported that the structure as well as the position and the number of OH groups affects the antioxidant activity of phenolic compounds, producing different results of the two assays (Platzer et al., 2021).
It has been widely demonstrated that different classes of polyphenols are able to interact with proteins, however, several factors could influence the strength of binding and affinity, such as pH, ionic strength, as well as the protein and polyphenols structures. Furthermore, changes in the temperature, may induce modifications in protein structures and ligand solubility, thus affecting the protein-phenol interactions. Several polyphenolic compounds were demonstrated to bind proteins by specific interactions with proline residues; therefore caseins, characterized by a high proline content, could represent a target of election for polyphenolic substances (Yildirim-Elikoglu and Erdem, 2017). The higher presence of caseins residues in GP+ Ricotta might explain the higher amount of TPCs in GP+ Ricotta. SDS-PAGE analysis did not show significant differences in the main protein fractions, α-lactoalbumin and β-lactoglobulin. This finding disagrees with a study of Chedea et al. (2017) that showed an increase of β-lactoglobulin but no effect for α-lactalbumin, albumin and caseins concentration in the milk of dairy cow fed a diet supplemented with 15% GP. However, the effect of polyphenol compounds on protein synthesis depends on several factors including type of compound, its concentration and the eventual presence other compounds with which competition can be generated.
Red GP supplementation modified fatty acid profile of WBR and Ricotta. To our knowledge, no research has characterized the fatty acid profile of whey obtained from the processing of cheeses produced with milk deriving from ewes fed GP. However, previous studies have highlighted an increase of linoleic acid (C18:2) and C18:1, t11 in the milk of lactating ruminants fed a diet supplementation with grape processing products, as GP and grape seed (Correddu et al., 2015; Ianni et al., 2019a). Conversely to the previously mentioned studies, Manso et al. (2016) observed an increase of C18:2 concentration but no modifications for C18:1, t11 and in the relative percentages of SFA, MUFA, and PUFA, by administering to ewes diets containing linseed oil and GP with different concentrations, 5 and 10 g/100 g of TMR. In our study, no differences between the two groups were observed in C18:2 but a significant increase was found in C18:1, t11 both in GP+ whey and Ricotta samples. The differences observed in whey and Ricotta fatty acid profile compared to milk could be due to the cheese manufacturing process.
GP+ whey and Ricotta samples were characterized by a lower content of odd-chain fatty acids, C15:0 and C17:0. These acids can be produced by rumen microbial fermentation and microbial de-novo lipogenesis. Rumen microbial population produce odd-chain fatty acids by a pathway which utilizes the removal of the α-carbon, through the conversion of C16:0 or C18:0 to a hydroxyl fatty acid followed by decarboxylation to produce either C15:0 or C17:0, respectively. These odd-chain fatty acids are then produced in the rumen and absorbed by the mammary gland for milk fat production (Vlaeminck et al., 2006). The reduction in C15:0 and C17:0 could be a consequence of the intake of the polyphenols deriving from GP, because of the ability of these compounds to influence the rumen microbiota (Vasta et al., 2010).
In addition to this, the diet administered to the GP+ group conferred a decrease of C14:1 and an increase of C14:0 respect to the CTRL both in whey and Ricotta, this result might be mainly related to an inhibitory effect on the myristic acid desaturation by stearoyl coenzyme A desaturase, a result also supported by the decrease of C14:1/C14:0 ratio, that was considered by Mele et al. (2007) a reliable index of Δ9-desaturation in the mammary gland. No significant variations in total amount of SFA, MUFA, and PUFA and consequently in AI and TI were highlighted.
The volatile profile of dairy products could be affected by the diet administered to lactating ruminants (Bennato et al., 2019; Bennato et al., 2020; Ianni and Martino, 2020; Ianni et al., 2019b). The analysis of VOCs has allowed to identify nine compounds, belonging to aldhehydes, ketones and carboxylic acids families, resulting by secondary lipid oxidation. In both groups the most abundant was hexanal, whose concentration was lower in GP+ samples after 5 d of ripening compared with CTRL. Furthermore, nonanal and octanoic acid were affected by the diet, resulting respectively lower and higher in GP+ samples compared with CTRL Ricotta.
Since hexanal and nonanal are typical secondary oxidation products of unsaturated fatty acids, their reduction suggest that GP supplementation may contribute to induce an antioxidant effect; at the same time this may negatively influence the aromatic parameters of the Ricotta, since the presence of hexanal in dairy products is strictly related to green, lemon, slightly fruity and herbal notes.
Conclusion
The present study suggests that compounds present in GP may interfere with animal biological function and could be transferred in milk even following a metabolic transformation by rumen microbes. After the cheese-making, these compounds could directly affect the chemical-nutritional characteristic of dairy products. The results obtained in this study suggest that the supplementation of GP in the ewe’s diet did not induce a worsening of the quality parameters of dairy by-products and a possible improvement of the oxidative stability, however further investigations are needed in order to improve the characterization of the biological mechanisms responsible for these findings, without neglecting sensorial evaluations useful to evaluate the acceptability of the product by the consumer.