Introduction
Lipid oxidation and proteolysis are factors that cause significant biochemical changes in the quality characteristics of dry-cured meat. Previous studies have well-established the relationship between quality characteristics and these factors (Toldrá, 1998). The majority of volatile compounds originate from the chemical or enzymatic oxidation of unsaturated fatty acids. These compounds can then further interact with proteins, peptides, and free amino acids. In the case of lipid oxidation, fatty acids generated through lipolytic enzymes in dry-cured meat generate volatiles through oxidation reactions (Gilles, 2009; Toldrá, 2006). Regarding proteolysis, amino acids released from meat proteins form volatile compounds due to Strecker degradation and Maillard reaction (Luo et al., 2021; Pérez-Santaescolástica et al., 2018; Toldrá, 1998). Volatile compounds generated by these two biochemical pathways are a key factor in aroma/flavor enhancement, and independent research on their effects on quality characteristics has been conducted by researchers. Górska et al. (2017) divided 21 dry-cured loins into three groups according to the characteristics of volatile compounds through principal component analysis (PCA) and evaluated the correlation between volatile compounds and sensory characteristics through sensory evaluation of each group. In addition, the relationship between volatile compounds and sensory properties as well as the relationship between physicochemical properties has been demonstrated (Marušić Radovčić et al., 2016; Petričević et al., 2018). Luo et al. (2021) mentioned that 3-methylbutanal is a compound produced by the interaction between leucine and some branched amino acids, and Villalobos-Delgado et al. (2014) reported that benzene acetaldehyde is produced through Strecker degradation from phenylalanine. In addition, it has been reported that furan and non-heterocyclic sulfur compounds are produced (Flores, 2018). Consequently, amino acids produced by proteolysis not only act as flavor precursors themselves but also affect various flavor/aroma-related compounds through additional biochemical reactions. Therefore, a better understanding of lipid oxidation and proteolysis during manufacturing may contribute to generating the volatile compounds in final products.
Many previous studies have reported the effects of lipid oxidation or proteolysis levels on changes in the quality characteristics of dry-cured meat. Pérez-Santaescolástica et al. (2018) compared quality characteristics in three dry-cured ham groups (low, proteolysis index<32%; medium, 32%≤proteolysis index≤36%; high, proteolysis index>36%) according to the proteolysis level, and the authors reported that an increase in proteolysis led to a rise in texture profile analysis (TPA) adhesiveness and a decrease in the quantity and quality of volatile compounds. They argued that the processing conditions conducive to lipid oxidation, such as increased salt content, typically, restrict the function of proteolytic enzymes. On the other hand, Marušić Radovčić et al. (2021) identified that during the manufacturing period, the biceps femoris, where proteolysis was high, exhibited a greater increase in volatile compounds than the semimembranosus. In addition, the authors mentioned that a moderate degree of lipid oxidation could have a positive effect on the flavor enhancement of dry-cured ham and that a high degree of lipid oxidation caused damage to the nutritional, functional, and sensory properties. Previous studies have shown that not only volatile substances but also other quality characteristics (texture properties, color, fatty acids, amino acids, etc.) could be changed by lipid oxidation or proteolysis.
Although the effect of lipid oxidation or proteolysis on quality characteristics is also important, understanding the relationship between them is essential for a better understanding of the quality characteristics. However, research related to elucidating the relationship between lipid oxidation and proteolysis is very limited. A discussion on the relationship between lipid oxidation and proteolysis can only be found in one paper. Harkouss et al. (2015) reported a quantitative correlation between lipid oxidation and proteolysis and established a linear regression model with a high coefficient of determination, regardless of muscle type, from 0 to 21 weeks during the entire manufacturing period of dry-cured ham (r2=0.92). The scarcity of this information is likely due to the inability in the manufacturing process to selectively initiate chemical reactions associated solely with protein or lipid oxidation or degradation. To overcome this limitation, in this study, the opposite of the experimental group was confirmed through a statistical procedure for the study group based on the 2-thiobarbituric acid reactive substances (TBARS) and proteolysis index (PI) of the generally prepared dry-cured loin. Furthermore, this study has the advantage of considering not only proteolysis but also lipid oxidation at the same time.
Lipid oxidation and proteolysis-related changes are biochemical reactions that occur simultaneously. Therefore, the relationship between the two reactions needs to be clearly established. Our hypothesis suggests that proteolysis may substantially impact quality characteristics considering the approximate composition of raw meat. Additionally, the effect of lipid oxidation may be greater when it is a limited volatile compound. The purpose of this study was to compare the quality characteristics of dry-cured loins by grouping them according to the levels of lipid oxidation and proteolysis, and to discuss the relationship between lipid oxidation and proteolysis on quality characteristics.
Materials and Methods
Sixty pork loins at 2 d postmortem from the right side of the carcass were employed for this study. Pork loin was purchased six times at two-month intervals from a local butcher block in Jinju, Korea. At the time of each purchasing, the pork loins were assigned a sample code (random 4-digit code). The coded pork loins were left in a cooler until 3 d postmortem before manufacturing dry-cured loins. The fresh pork loin was salted after all connective tissues were removed. For salting, purified sodium chloride was used and 4% of the pork loin weight was rubbed on the surface. Then, it was vacuum-packed and stored in a refrigerator at 4°C for 7 d. The surface of the cured loin was washed in tap water and the water on the surface was removed with a paper towel. The cured loins were placed in a permeable fibrous casing and dried at 9°C (50%–55% RH) for 14 d, then the temperature was raised by 1°C every 3 d, and finally aged at 18°C (65%–70% RH). The total production period was 55 d. The dry-cured loins were removed from the casing and stored at –80°C until analysis. To assign samples based on lipid oxidation and proteolysis, the dry-cured loins were measured for lipid oxidation using TBARS and for proteolysis using PI. After dividing the samples into four groups based on TBARS and PI, detailed information is presented in Table 1.
Sample classification | PI range | TBARS range | n |
---|---|---|---|
HH | X≥10.6 | X≥0.88 | 16 |
HL | X≥10.6 | X<0.88 | 9 |
LH | X<10.6 | X≥0.88 | 18 |
LL | X<10.6 | X<0.88 | 17 |
The TBARS of dry-cured loin was determined with Bozkurt and Erkmen (2004) with some modifications. A 3 g of sample was homogenized with 27 mL of 3.86% perchloric acid using homogenizer (T25 Basic, IKA, Wilmington, NC, USA). The homogenate stood in a cold room for an hour. After standing, the homogenate was centrifuged at 2,000×g for 10 min and filtered with Whatman No. 1 filter paper. The 2 mL of filtrate was mixed with 2 mL of 20 mM TBA solution and left for 16 hours at room temperature with dark conditions. For the blank, 2 mL of deionized water was used to replace for filtrate. The TBARS was measured at 531 nm using Cary 60 spectrometer (Agilent Technologies, Santa Clara, CA, USA). A standard curve was calculated using 1,1,3,3‐tetraethoxypropane. The total nitrogen content (TN) was measured with 0.5 g of sample using the nitrogen analyzer (TruMac CNS analyzer, Leco, MI, USA). The non-protein nitrogen content was determined according to Pérez-Santaescolástica et al. (2018) with some modifications. A 3 g minced sample was homogenized with 25 mL of deionized water, and 12 mL of 20% trichloroacetic acid (TCA) were introduced into the homogenate. The mixture was thoroughly agitated and then left to stabilize for an hour at room temperature. Subsequently, it was centrifuged again at 2,000×g for 10 min. Post centrifugation, the supernatant was filtered. A 15 mL of the filtrate was then employed to determine the nitrogen content, using the same procedure as was used for determining TN content by Kjeldahl method. Finally, the PI was calculated using the formula (non-protein nitrogen / total nitrogen) × 100, as described by Pérez-Santaescolástica et al. (2018).
Moisture was performed by drying method the samples to a constant weight at 103°C (method 934.01, AOAC, 2005). The TN content was determined as described previously in the materials and methods section. The protein content was calculated by multiplying the TN content by 6.25. The fat content was conducted with Soxhlet extraction method with diethyl ether (method 920.39, AOAC, 2005).
The dry-cured loins were cut to the same size [8 cm (W)×5 cm (L)×3 cm (H)], and three pieces were used for instrumental color. Each piece was measured at different locations five times. The instrumental color was analyzed by colorimeter (CR-400, Konica Minolta, Tokyo, Japan) with an 8 mm aperture using an illuminant with D65 and 2° standard observer after calibrating with a calibration plate (Y=81.2; x=0.3191; y=0.3263). The CIE L*, CIE a*, and CIE b* were used as color parameters. The mean value was read 5 readings for each piece and took the average of 3 pieces.
The 3 g of minced sample was homogenized with 27 mL of deionized water using homogenizer (T25 Basic, IKA). The pH of the sample was measured with a pH meter (S20 SevenEasyTM, Geifensee, Switzerland) after calibrating with 7.00, 4.01, and 9.21 using pH buffer.
The TPA was performed using EZ-SX (Shimadzu, Kyoto, Japan) with a flat bottom (5 mm diameter) probe. The 3 pieces of the sample was used after measuring the instrumental color. A double compression test was carried out with 50% compression of the original sample height. Force-time curves were expressed with a 500 N load cell applied at a 100 mm/min crosshead speed. The texture parameters were calculated according to Bourne (1982). The mean value was taken from two random points in each sample piece.
Free amino acid composition was extracted as described by Aro et al. (2010) with some modifications. Briefly, a 3 g of sample and 27 mL of deionized water were homogenized for 30 s and 10 mL of 10% TCA solution was added and shaken at 250 rpm for 1 h at room temperature. After an hour, centrifugation was performed at 10,000×g for 10 min and the supernatant was filtered using Whatman No.1. Finally, it was filtered with a 0.2 μm syringe filter, transferred to a 1.5 mL vial, and used for analysis. A 20 μL of sample was injected into amino acid analyzer (Biochrom 30, Biochrom, Cambridge, UK). Amino acids were derivatizated with 6-aminoquinolyl-Nhydroxysuccinimidyl carbamate (Waters AccQ-Fluor reagent kit) and used the standard with 40 amino acids (Physiology standard, Biochrom). The results were expressed as mg of free amino acid/100g of sample.
Lipid extraction was carried out according to Folch et al. (1957). Saponification, methylation, and gas chromatography (GC) learning conditions were employed by Seo et al. (2021). The fatty acid methyl etsers (FAME) were separated with GC (7890B, Agilent Technologies) equipped with a Supelco SP®-2560 capillary GC column (100 m×0.25 mm×0.20 μm). The individual FAMEs were identified based on a standard mixture of Supelco 37 Component FAME Mix (Sigma-Aldrich, St. Louis, MO, USA). The amount of individual fatty acids and fatty acid groups identified was calculated with internal standard (C11:0, undecanoic acid). The fatty acid was expressed as g/100 g tissue.
The solid phase micro-extraction (SPME) fiber was used after pre-conditioning at 270°C for 30 min according to the manufacturer’s manual before use. Briefly, 2.5 g of the minced sample was placed in a 20 mL screw-capped vial and sealed with a screw cap fitted with polytetrafluroethylene (PTFE) septa. The adsorption of volatiles was carried out for 55 min in an incubator at 45°C using 50/30 μm DVB/CAR/PDMS SPME fiber (57328-U, Supelco, Bellefonte, PA, USA). After adsorption, the SPME fiber was immediately injected into the GC inlet and desorbed for 3 min in pressure splitless mode (30 psi). At this time, the GC inlet temperature was 240°C. Before adsorbing the next sample, pre-conditioning was performed for 8 min while flowing carrier gas (Helium, 99.999%) at 240°C at 100 mL/min for overall SPME fiber cleaning.
The GC machine was 7890B (Agilent Technologies). Helium was used as the carrier gas and a constant flow rate of 1.0 mL/min was used. The GC column was a Supelcowax 10 capillary column (60 m×0.32 mm×0.25 μm, Supelco). The oven temperature program is described in Domínguez et al. (2019) and analyzed for a total of 49.5 min. A mass spectrometer of 5975C (Agilent Technologies) was used, and the temperatures of the mass transfer line, ion source, and quadrupole were 260°C, 230°C, and 150°C, respectively. Mass spectra were obtained at 70 eV electric shock and eletron voltage multiplier (EMV) of 1,080, and data were collected in the m/z range of 40–450 at a scan speed of 3.4 times per second. Compounds were identified by comparing their mass spectra with those contained in the NIST 20/Wiley 12th library. The results are expressed as area units (AU)×105/g of sample.
All quality characteristics were taken an average of three replication on each measurement for further statistical analysis. The distribution of sample groups was expressed as a score plot using PCA using data from physicochemical variables by MetaboAnalyst (version 5.0; https://www.metaboanalyst.ca/). Mixed model was used to confirm the difference in physicochemical properties in the sample groups according to the TBARS value and PI. The mixed model was performed using the mixed procedure of SAS 9.4. The statistical models were used to evaluate the quality characteristics (moisture, instrumental color, pH, TPA, fatty acid content, free amino acid content, and volatile compounds) of the dry-cured loin. These models included a fixed effect for the sample group and a random terms for processing batch and sample replication. Least squares means for all traits of interest were separated (F test, p<0.05) by using least significant differences analyzed by the protected difference option in SAS.
Results and Discussion
Table 1 and Fig. 1 show the sample descriptions used in this study. PI and TBARS have been widely used as indicators of proteolysis and lipid oxidation in many previous studies, respectively (Marušić Radovčić et al., 2021). The sample groups used in this study showed significant differences in proteolysis and lipid oxidation levels (p<0.05). Sample grouping was also performed using PCA (Fig. 2). As a result of the PCA, PC1 and 2 from sample groups were explained 73% variance, which may consider a clear distribution between the groups. Therefore, the grouping of samples based on PI and TBARS appears to be highly appropriate.
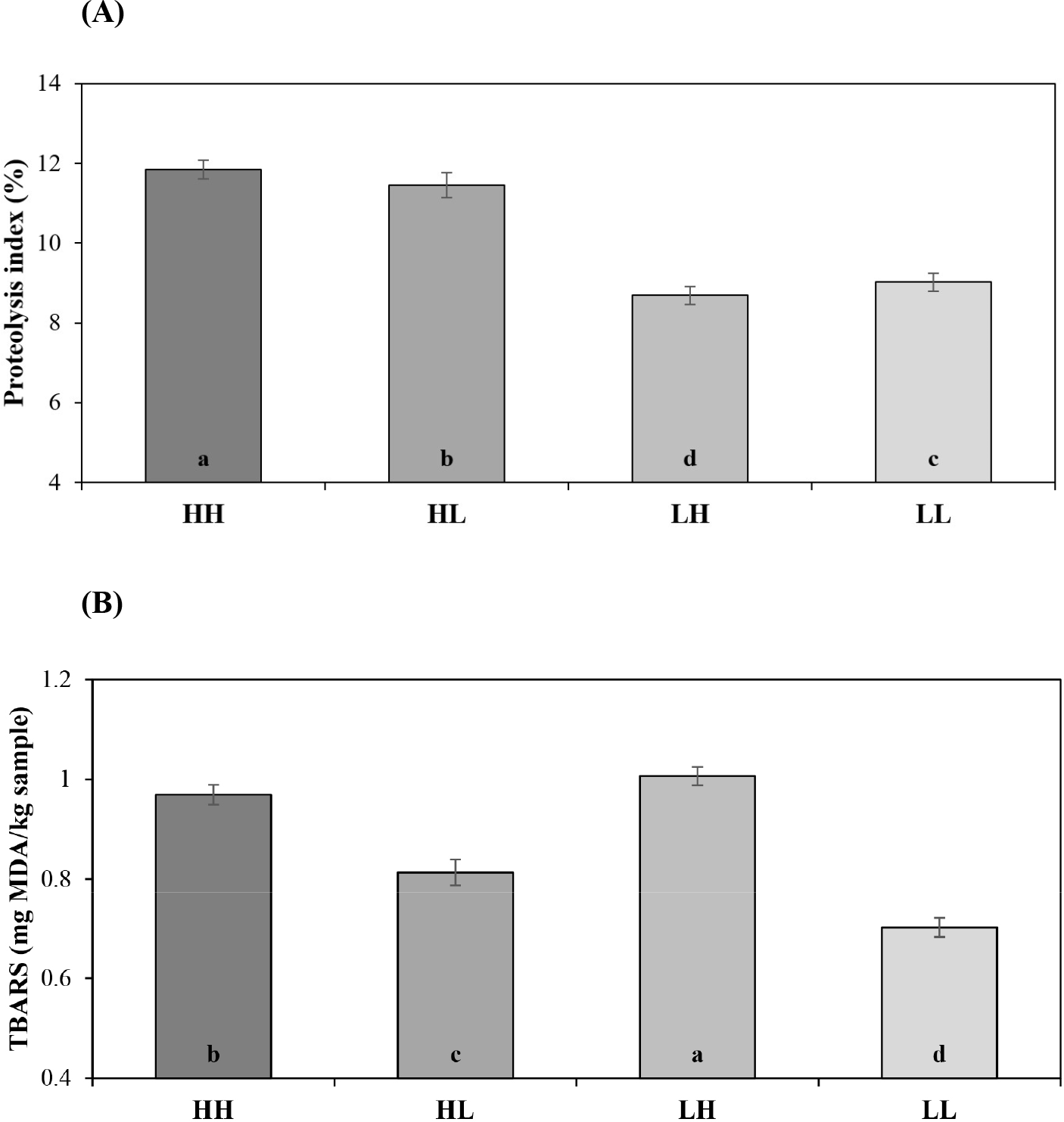
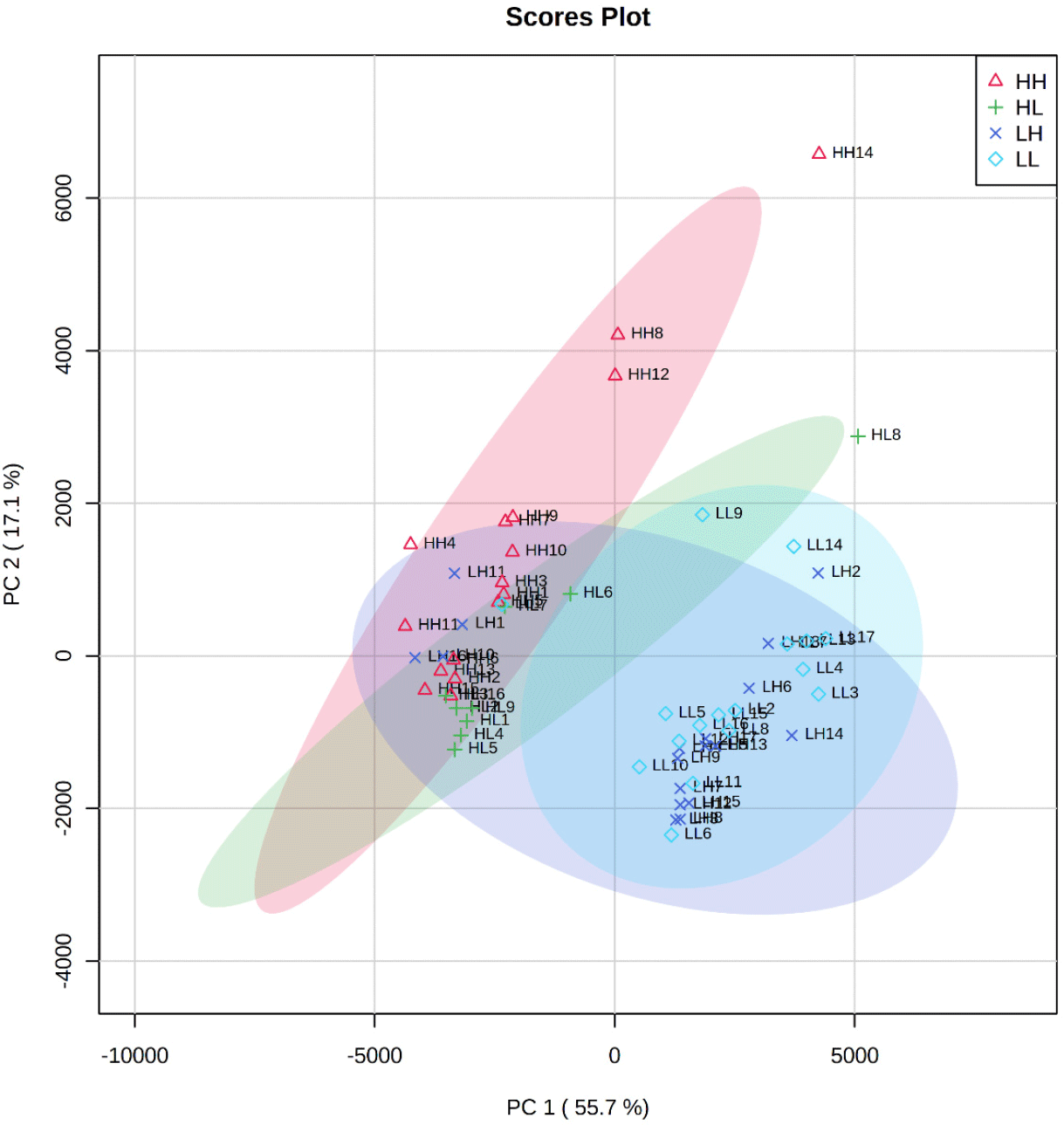
In dry-cured ham, the PI reflecting excellent quality was reported in previous studies as 33%–36% (Spanish style) and 22%–30% (Italian style; Careri et al., 1993; Pérez-Santaescolástica et al., 2018). However, in products using pork loin, there is no mention of the PI range for good quality yet. The PI values in the current study ranged from 7% to 14%, consistent with data reported by other authors (Abellán et al., 2016; Stadnik and Dolatowski, 2013). This difference between dry-cured ham and dry-cured loin is due to manufacturing conditions such as raw material, salting period, and drying-ripening period. Our TBARS (0.6–1.1 mg MDA/kg sample) results are consistent with those reported by Muriel et al. (2007) and Soto et al. (2008). In addition, Hoz et al. (2007) reported that the overall acceptability of dry-cured loins with 0.87 to 1.24 mg of TBARS was higher than that of dry-cured loins with higher TBARS. The results suggest that the dry-cured loin utilized in this study can be characterized as exhibiting normal quality. Therefore, despite the absence of a definitive statement regarding the specific ranges of PI and TBARS, dividing these values into four groups based on the median value from the present study may be a somewhat compelling approach.
Table 2 presents the results of physicochemical properties for the four dry-cured loin groups based on the levels of proteolysis and lipid oxidation. Moisture content exhibited a significant difference between groups (p<0.05), with the PI and TBARS of high levels (HH) group showing the highest moisture content at around 51%, and the PI and TBARS of low levels (LL) group exhibiting the lowest at around 47%. In protein content, on the other hand, high proteolysis groups [HH and PI of high level and TBARS of low level (HL)] were significantly lower than low proteolysis groups (p<0.05). Also, the fat content was observed same significant result as for protein content (p<0.05). CIE a* showed a significant difference among the sample groups, with HH and HL exhibiting higher values, while PI of low level and TBARS of high level (LH) and LL showed relatively lower values (p<0.05). TPA hardness and cohesiveness showed significant differences across groups (p<0.05). HH and HL exhibited lower TPA hardness than LH and LL, while TPA cohesiveness was higher. Thus, the level of proteolysis and lipid oxidation did not significantly affect the CIE L*, CIE b*, and pH (p>0.05), but they notably influenced the other variables, with proteolysis seen to giving a more substantial effect than lipid oxidation.
The current results of moisture, protein and lipid content in dry-cured loin were agree with previous studies (Bermúdez et al., 2015; Ortiz et al., 2020; Seong et al., 2015). Moisture content is an important factor in determining the quality of dry-cured meat products, as the level of moisture dehydration directly affects tissue properties and various enzyme activities (Harkouss et al., 2015). In addition, moisture is one of the technological traits that determine not only dryness but also storage stability of dry-cured meat (Seong et al., 2015). HH exhibited a higher moisture content than HL, indicating weaker dehydration in HH than in HL. Accordingly, lipid oxidation and proteolysis were more active in HH than in HL. Moreover, the difference in moisture content between HH and HL was only about 1%, whereas HH and LH showed a difference of about 3%. Therefore, proteolysis may have a more significant influence than lipid oxidation on moisture, protein and fat content in dry-cured loin. Additionally, both the protein and fat contents changed due to variations in moisture content brought about by dehydration. In particular, the protein content increased, reaching levels similar to that of the water content. Such changes can significantly impact subsequent biochemical reactions, potentially altering the reaction products.
Our findings align with Pérez‐Alvarez et al. (1999) report of high CIE a* in dry-cured ham muscles with high moisture content, citing nitrosomyoglobin formation as the cause. The CIE a* may be influenced by the denaturation of nitrosomyoglobin due to the production of lactic acid during ripening (Lorenzo et al., 2013). Therefore, it can be hypothesized that proteolysis affected nitrosomyoglobin and production of lactic acid. However, this explanation alone does not sufficiently clarify why the sample group with higher proteolysis exhibited stronger CIE a*. Thus, additional research is needed on the relationship between proteolysis and color in dry-cured loin.
The observed hardness and cohesiveness cannot be entirely explained by moisture content alone, as proteolysis and lipid oxidation effects must also be considered. In HH and HL, the dry matter’s interference in TPA measurement is reduced due to high moisture content, while LH and LL, with low moisture content, increase the interference of dry matter, resulting in the current hardness result. Dry-cured loin shrinks gradually during manufacturing, which may result in increased cohesiveness. LH and LL, which were highly dehydrated, contracted more strongly, and cohesiveness increased as the dehydrated empty space naturally decreased. Seo et al. (2021) also reported similar findings to ours.
Table 3 shows the fatty acid content in relation to the levels of proteolysis and lipid oxidation. No significant difference was observed in saturated fatty acids (SFA) between the sample groups (p>0.05). However, monounsaturated fatty acids (MUFA) and polyunsaturated fatty acids (PUFA) exhibited significant differences between the groups with strong significance levels of p<0.05 and p<0.001, respectively. Concerning individual fatty acids, significant differences were found between sample groups for SFA at C10:0, C12:0, and C20:0 (p<0.05). For MUFA and PUFA, there were no significant differences between sample groups in C18:1 n-7 and C20:4, respectively, but significant differences were observed in other fatty acids (p<0.05). In particular, there was a significant difference for C16:1, C18:2 n-3, C18:3 n-6, C20:1, C20:2 and C20:3 (p<0.05). The amount of MUFA was significantly lower in HH than other sample groups (p<0.05). On the other hand, there was no significant difference between LH and LL in MUFA (p>0.05). Therefore, MUFA was affected by lipid oxidation in the high proteolytic sample group but not affected by lipid oxidation in the opposite case. In terms of the PUFA, HH and HL were significantly lower than LH and LL (p<0.05). These results indicated that higher proteolytic levels were associated with lower amount of PUFA, regardless of the degree of lipid oxidation. Conversely, lower proteolytic levels were linked to higher amount of PUFA, regardless of the degree of lipid oxidation. Additionally, there does not appear to be a noticeable effect of lipid oxidation on changes in PUFA. These results were reflected in the total fatty acid content, and HH and HL had a significantly lower total fatty acid content than LH and LL (p<0.05). Therefore, it may be suggested that proteolysis contributes more to changes in fatty acid content than lipid oxidation.
PI, proteolytic index; TBARS, 2-thiobarbituric acid reactive substances; HH, PI and TBARS of high levels; HL, PI of high level and TBARS of low level; LH, PI of low level and TBARS of high level; LL, PI and TBARS of low levels; ns, no significance; SFA, saturated fatty acid; MUFA, monounsaturated fatty acid; PUFA, polyunsaturated fatty acid.
Previous studies have reported that the content of SFA, MUFA, and PUFA decreases in the final product compared to the initial stage (Andres et al., 2005; Gilles, 2009; Salazar et al., 2016). During this time, mainly neutral lipids and phospholipids decrease. In the present study, proteolysis induced more changes in fatty acid content than lipid oxidation, and these changes are mainly attributable to PUFAs. The most probable hypothesis that can be inferred is the interaction between protein and lipid oxidation. Oxidation reactions may easily lead from fat and protein, causing various changes in food. Among these changes, the loss of enzyme activity, which can directly affect the quality of dry-cured meat products, is a representative example (Viljanen et al., 2004). Considering that our dry-cured loin had a significantly higher protein content than fat content on a dry matter basis (Table 2) and that the oxidation reaction is a chain reaction, the following conclusions can be drawn. Fat-initiated oxidation can induce a cascade of oxidation reactions and promote the oxidation of proteins. Carbonylated proteins may be more susceptible to enzyme-induced proteolysis (Nyström, 2005). Gan et al. (2019) also mentioned the relationship between proteolysis and protein oxidation, arguing that protein oxidation can cause changes to protein structure, leading to unfolded proteins that are more reactive forms for proteases. Consequently, the final products such as hydroperoxide from protein-lipid-derived oxidation may have induced changes in fatty acids (Lund et al., 2011; Viljanen et al., 2004). Among fatty acids, PUFA is known to undergo the most severe changes due to oxidation, with n-6 type fatty acids being the most severely reduced as oxidation proceeds (Sampaio et al., 2012). Therefore, in the group with high proteolysis, the content of PUFAs may have decreased, which may have affected the volatile compounds.
Table 4 presents the content of free amino acids. Except for taurine and anserine, there was a significant difference between groups overall (p<0.001). HH had the highest content at approximately 2,309 mg and LL had the lowest content at 1,799 mg (p<0.05). Furthermore, there was no significant difference between HL and LH, with both having a content between 1,880 and 1,895 mg (p<0.05). Aspartic acid, threonine, alanine, valine, and isoleucine showed the same results among sample groups, with HH having the highest content and LL having the lowest content (p<0.05). Similarly, serine, asparagine, glutamic acid, glycine, isoleucine, leucine, tyrosine, and phenylalanine showed the same trend, with the highest content in HH and the lowest content in LL (p<0.05). Additionally, there was no significant difference between HL and LH (p<0.05). These findings were consistent with those of Cittadini et al. (2020), who reported that free amino acid content was high in the group with high TBARS. In summary, proteolysis or lipid oxidation could influence the generation of free amino acids, with high levels of either leading to an increase in the content of free amino acids. Furthermore, high levels of both could lead to the highest content of free amino acids.
As discussed earlier, free amino acids are considered as representative indicators of proteolysis. Therefore, the changes in free amino acid levels according to various conditions have been investigated in several previous studies. Garrido et al. (2012) reported that the total amino acid content decreased with an increase in salting time, as higher salt concentrations can inhibit proteolytic activity. Protein degradation through cathepsin B, L, and calpain activity promotes the production of small peptides and free amino acids. In particular, myosin heavy chain, C protein, and desmin are degraded by proteolytic enzymes, which can contribute to the generation of free amino acids (Zhou et al., 2017).
Lipid oxidation is a significant chemical reaction that occurs in meat and meat products. It is a breakdown of lipid components and is closely related to lipolytic activity, which releases free fatty acids (Muriel et al., 2007). Reactive oxygen species typically initiate lipid oxidation by reacting with fatty acids (Domínguez et al., 2019). Lipid oxidation products such as hydrogen peroxide, lipid-free radicals, and volatile secondary oxidation products can react with proteins to generate protein-centric free radicals (Hematyar et al., 2019). Furthermore, carbonylation of proteins is promoted by lipid oxidation, and carbonylated proteins are more sensitive to proteolytic enzymes (Nyström, 2005). Moreover, the folded structure of natural proteins forms a rigid structure that makes it difficult for proteolytic enzymes to act as a substrate (Fontana et al., 2004). However, oxidation-induced changes in the secondary and tertiary structures of proteins result in an unfolded state, leading to an increase in the site of action of proteases and protein degradation (Grune et al., 2004). This ultimately leads to an increase in the production of free amino acids released by proteolysis.
The volatile compounds were analyzed by the sample group and shown in Table 5. A total of 27 volatile compounds were detected, consisting of 11 alcohols, 4 alkanes, 6 ketones, and 6 aldehydes. Of these, 7 alcohols, 3 alkanes, 3 ketones, and 4 aldehydes showed significant differences between groups (p<0.05). For ∑alcohol, ∑alkane, ∑ketone, and ∑total, HH and HL were high, while LH and LL were low (p<0.05). However, ∑aldehyde showed the opposite result (p<0.05). Therefore, the sample group with high protein degradation (HH and HL) produced a large amount of total volatile compounds. Interestingly, there was no difference according to lipid oxidation in the sample group with high protein degradation, but there was a significant difference according to lipid oxidation in the opposite case (p<0.05).
An additional discussion was not conducted because the properties of individual volatile compounds are widely known in dry-cured meat.
The formation of volatile compounds in dry-cured meat products is primarily considered a result of lipid oxidation. Due to the lipolytic activity (enzymatic and oxidative) of dry-cured meat, unsaturated fatty acids are converted into various compounds, mainly aliphatic compounds such as alcohols, aldehydes, and ketones. In dry-cured meat, volatiles are mainly produced by lipid oxidation in the initial processing step, whereas in the ripening step, both lipid and amino acid degradation occur (Gilles, 2009). In fact, among the results of our study, the amount of the PUFA and volatile compounds showed the same manner between groups, which is likely due to the effect of proteolysis. Therefore, the change in PUFA was caused by proteolysis, and it could be inferred that the current volatile compound result is also due to this. Earlier, it was explained that oxidation was carried out by proteolysis and had an effect on PUFA. A previous study reported that lipid oxidation is closely related to volatile compounds (Domínguez et al., 2014). Additionally, lipid oxidation usually occurs in PUFA, as the double bond site has a radical reactive site, making it more sensitive with more double bonds (Domínguez et al., 2019). Therefore, during the dry-aging process of dry-cured loin, PUFA are converted to volatile compounds due to lipid oxidation, generating alcohols, aldehydes, and ketones. Moreover, free amino acids are known to act as precursors of volatile compounds and are mainly produced by Strecker degradation and Maillard reaction, with heterocyclic compounds containing sulfur or nitrogen and aliphatic compounds being produced (Jurado et al., 2007; Toldrá, 1998).
Conclusion
The study investigated how proteolysis and lipid oxidation affect key quality parameters during the manufacturing of dry-cured loin. The results of this study demonstrated that varying levels of proteolysis and lipid oxidation can influence the physicochemical properties, total fatty acid content, total free amino acids content, and amount of volatile compounds in dry-cured loin. While lipid oxidation did not affect the fatty acid content, it did influence the free amino acid content between sample groups. Proteolysis, however, impacted both. Intriguingly, volatile compounds were influenced by the level of lipid oxidation when proteolysis was high but remained unaffected by lipid oxidation levels when proteolysis was low. These results implicate that lipid oxidation and proteolysis can directly cause changes in quality characteristics when manufacturing dry-cured loin. It also suggests that not only lipid oxidation but also proteolysis may play an important role in the formation of volatile compounds in dry-cured loin.