Introduction
Enterococcus faecalis is a gram-positive bacteria that can be isolated from normal intestinal flora and optionally used as probiotics with E. faecium (Baccouri et al., 2019; Zommiti et al., 2018). However, E. faecalis is also known as an opportunistic pathogen that can induce peritonitis, urinary tract infections, and bacteremia (Lee et al., 2017). E. faecalis is reportedly able to make biofilms on food-processing plants, which could be a major microbial contaminant of foods (Liu et al., 2013; Pesavento et al., 2014). Therefore, E. faecalis is considered a foodborne pathogen, which can induce various infectious diseases accompanied by inflammatory responses (Giraffa, 2002).
A bacterial biofilm is a complex structure embedded in a matrix of exopolysaccharides, membrane vesicles, and bacterial DNA. This polymeric structure protects the bacterial cells from environmental stresses such as nutritional limitation, pH alteration, and osmolarity (Fux et al., 2005). In addition, this polymeric structure prevents the action of antibiotics, which results in the production of antibiotic-resistant bacterial populations. This causes severe problems for curing inflammatory responses in chronic wounds (Engemann et al., 2003; Hoyle and Costerton, 1991; Ngo et al., 2012; Wolcott et al., 2008). Therefore, demand for new agents that can more effectively control biofilm formation and biofilm-derived inflammatory responses continues to grow.
Honey is an edible natural agent that is produced by bees from the nectar of flowering plants. Honey mainly consists of primarily sugars, including oligosaccharides, and also contains minerals, vitamins, and plant-derived phenolic compounds (Bogdanov et al., 2008; da Silva et al., 2016; Qiu et al., 1999). Honey has been recognized as a medicinal food because of its anti-inflammatory, anti-oxidative, and anti-bacterial activities (Ranneh et al., 2021; Sherlock et al., 2010; Stagos et al., 2018). Since the components of honey vary with weather condition, geographic location, and floral source, monofloral honeys, which are produced at different locations, show a range of biological activities (Allen et al., 1991; AL-Waili et al., 2013).
The Hovenia (Hovenia dulcis) tree is a potential candidate for a honey plant in Korea because Hovenia flowers produce higher amounts of nectar than Acacia flowers, and one of their characteristics is that they prefer to grow in a sunny position. Therefore, in Korea, areas where Hovenia trees are being cultivated are gradually increasing. Physiochemical analysis, antioxidant, and anti-bacterial activities of Hovenia monofloral honeys (HMHs) were estimated in our previous study (Park et al., 2020). However, although HMH showed strong anti-bacterial activity against gram-positive (Listeria monocytogenes and Staphylococcus aureus) and gram-negative (Salmonella Typhimurium and E. coli O157:H7) strains, the effects of HMHs on E. faecalis-mediated biofilm formation and inflammation has not yet been elucidated. Therefore, in this study, the anti-biofilm and anti-inflammatory effects of HMH were evaluated and their underlying molecular mechanisms were also investigated.
Materials and Methods
HMHs were produced two times on different days (HMH-1, HMH-2) using a net house system. The purity of the HMHs was estimated by pollen analysis according to Park et al.’s method (Park et al., 2020), and the HMHs were determined to have more than 95% Hovenia pollen. The HMHs were stored at 4°C in a refrigerator until analysis.
Rabbit anti-TLR-2 antibody was obtained from Invitrogen (Waltham, MA, USA). Rabbit anti-Myeloid differentiation primary response 88 (MyD88) (4283S), Rabbit anti-phospho-ERK (9102S), rabbit anti-ERK (9101S), rabbit anti-phospho-p38 (9212S), rabbit anti-p38 (9211S), rabbit anti-phospho-JNK (9251S), rabbit anti-JNK (9252S), and rabbit anti-GAPDH (5174S) were purchased from Cell Signaling (Danver, MA, USA).
E. faecalis (KCTC 3511), which was obtained from the Korean Collection for Type Culture (KCTC), was used in this study. E. faecalis was incubated in a brain heart infusion (BHI; BD Biosciences, Franklin Lakes, NJ, USA) medium at 37°C. To assess the effects of HMH on the biofilm formation of E. faecalis, various concentrations of HMH (0%–32%, w/v) were co-cultured with E. faecalis (1×107 /well) in a black polystyrene 96-well microtiter plate with a clear bottom (Greiner Bio-one, Kremsmunster, Austria) at 37°C in an incubator for 24 h. After washing with phosphate buffered saline (PBS) three times, the HMHs were stained with SYTO9 (25 μM) solution at room temperature for 30 min. After washing with PBS once, biofilm formation was examined visually using a confocal laser scanning microscope (Nickon, Tokyo, Japan).
To estimate the effects of HMH on the established E. faecalis biofilms, E. faecalis (1×107 /well) was first added to a 96-well microtiter plate and incubated at 37°C in an incubator for 24 h to form biofilms. After washing with PBS three times, HMH (0%–32%) was added and further incubated at 37°C in an incubator for 24 h. The biofilm was stained with a SYTO9 solution, as described as above, and examined visually using a confocal laser scanning microscope (Nickon). Biofilm mass were quantified by using the ImageJ program.
E. faecalis (2.5×104) with and without HMH was cultured in BHI broth at 37°C in an incubator for 2, 4, and 6 h. The growth of E. faecalis and cell viability were calculated by estimating the optical density at 595 nm and 450 nm respectively by using a microplate reader (iMark Microplate Reader, Bio-Rad Laboratories, Contra Costa County, CA, USA). The effects of HMH on E. faecalis growth was further estimated using a WST-1(2-[4-Iodophenyl]-3-[4-nitrophenyl]-5-[2,4-disulfophenyl]-2H-tetrazolium) cell viability assay kit according to the manufacturer’s protocol. Briefly, E. faecalis (2.5×104) was cultured in 96-well microtiter plate with and without HMH in a 37°C incubator for 24 h. Then WST-1 solution was added, and the samples were incubated at room temperature for an additional 30 min.
Estimating the amount of extracellular polymeric substances (EPSs) was conducted according to Yoon and Kang’s method (Yoon and Kang, 2020) with minor modifications. Briefly, HMH (0%, 16%, and 32%) was co-cultured with E. faecalis for 16 h and then the bacterial pellet was diluted in a PBS to reach a turbidity of 1.0 at OD600. Then, the E. faecalis suspension (1 mL) was mixed with 1 mL of Congo red solution (80 μg/mL) and further incubated at 37°C in an incubator for 2 h. After centrifuging at 4,000 relative centrifugal force (RCF) for 30 min, the optical density of the supernatant was estimated at OD492 using a UV/VIS spectrophotometer (Optizen POP, Mecasys, Daejeon, Korea) and the amount of Congo red was calculated by comparing the optical density of the standard curve of Congo red.
The HT-29 colorectal adenocarcinoma cell line, which was obtained from the American Type Culture Collection (ATCC, Manassa, VA, USA) and cultured in Dulbecco’s Modified Eagle’s Medium (DMEM, Welgen, Seoul, Korea), was mixed with 10% fetal bovine serum (Corning, Corning, NY, USA), 100 unit/mL of penicillin, and 100 μg/mL of streptomycin. To estimate the cytotoxicity of the HMH, HT-29 cells (1×104) were cultured on a 96-well cell culture plate at 37°C in a CO2 incubator for 24 h. Then, the culture media was changed to serum-free DMEM containing HMH (0–2 mg/mL) and further incubated at 37°C in a CO2 incubator for 24 h. Subsequently, 10 μL of WST-1 solution was added to each well and incubated for an additional 30 min in a CO2 incubator. The number of viable cells was calculated by estimating the absorbance at 450 nm using a microplate reader (iMark Microplate Reader, Bio-Rad Laboratories).
HT-29 cells (5×105 /wells) were seeded in six-well plates and cultured for 24 h. After incubation with serum-free DMEM for 16 h, E. faecalis (2×106 /well) was administered to HT-29 cells with and without HMH. After further incubation in a CO2 incubator for 16 h, it was lysed with protein extraction buffer (Lee et al., 2010) on ice for 1 h. After centrifuging (12,000×g) at 4°C for 15 min, the supernatants were separated and used for estimating the expression of each protein. Each protein sample was separated in a sodium dodecyl sulfate polyacrylamide gel electrophoresis (SDS-PAGE) and the gels were separated and transferred to nitrocellulose membranes. After being incubated with a blocking buffer (5% nonfat milk in Tris-buffered saline with 1% Tween 20), each antibody was first reacted with the membranes (1:1,500) for 12 h at 4°C. The membranes were washed with a Tris-buffered saline with Tween 20 buffer (TBST), and reacted with a horseradish peroxidase conjugated rabbit secondary antibody (1:3,000) (Abcam, Cambridge, UK) at room temperature for 2 h. Each band was examined visually using an enhanced chemiluminescence (ECL) detection reagent (Bio-Rad, Hercules, CA, USA).
HT-29 cells (5×105 /wells) were seeded in six-well plates and cultured for 24 h. After incubation with serum-free DMEM for 16 h, E. faecalis (2×106 /well), 10 μM of a control inhibitor (NBP2-29334, Novus, Centennial, CO, USA), and MyD88 inhibitor (NBP2-31226, Novus) were administered to HT-29 cells with and without HMH. After further incubation in a CO2 incubator for 16 h, the total RNA of the HT-29 cells, which were treated as above, was extracted using a TRIzol® Reagent (Invitrogen). Complementary DNA (cDNA), which was synthesized with 1 μg of the total RNA and 10 pM of oligo dT primer, was mixed with SYBR® Green Realtime PCR Master Mix (Toyobo, Tokyo, Japan) and qRT-PCR was performed to compare the transcriptional expression of the interleukin-8 (IL-8) gene in a RT-PCR detection system (CFX ConnectTM, Bio-Rad). A comparative ct method was used for evaluating the relative expression of the IL-8 gene and normalized to the expression of glyceraldehyde 3-phosphate dehydrogenase (GAPDH). The sequences of the IL-8 primers were 5’-TGTCCCATGCCACTCAGAGA-3’ (forward) and 5’-5’-AGCAGGTGCTCCGGTTGTAT-3’ (reverse); and those of the glyceraldehyde 3-phosphate dehydrogenase primers were 5’-ACGGGAAGCTCACTGGCA-3’ (forward) and 5’-TCCAGGC GGCATGTCAGA-3’ (reverse).
Results
To investigate whether or not HMH can inhibit the formation of E. faecalis biofilms, HMHs (HMH-1 and HMH-2) were first incubated with E. faecalis for 24 h. Then, the E. faecalis biofilms were examined visually by SYTO9 staining (Invitrogen) and quantified under a confocal laser scanning microscope. As shown in Fig. 1, the SYTO9 positive area, which is considered as the E. faecalis biofilm, was significantly (p<0.05) decreased when 16% and 32% (w/v) of HMHs was co-incubated with E. faecalis. In addition, to examine whether HMH could destruct the E. faecalis biofilms, various concentrations (0%–32%) of HMHs were administered to pre-formed E. faecalis and were examined visually by SYTO9 staining. As shown in Fig. 2, administration of 16% and 32% of HMHs to pre-formed E. faecalis biofilm significantly (p<0.05) decreased the SYTO9 positive area when compared with that of the control (non-treated). These results suggest that HMH could attenuate the formation of E. faecalis biofilm and could effectively eradicate the pre-formed biofilm of E. faecalis.
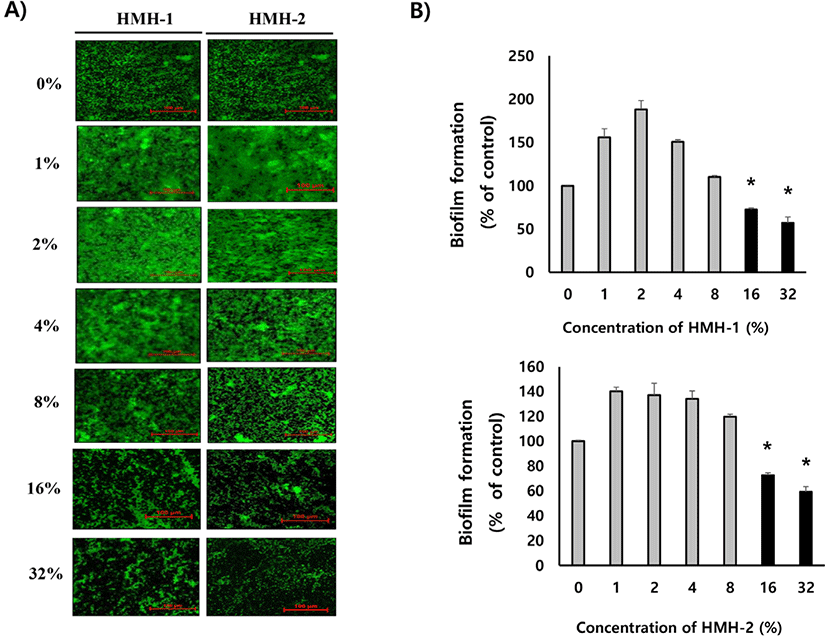
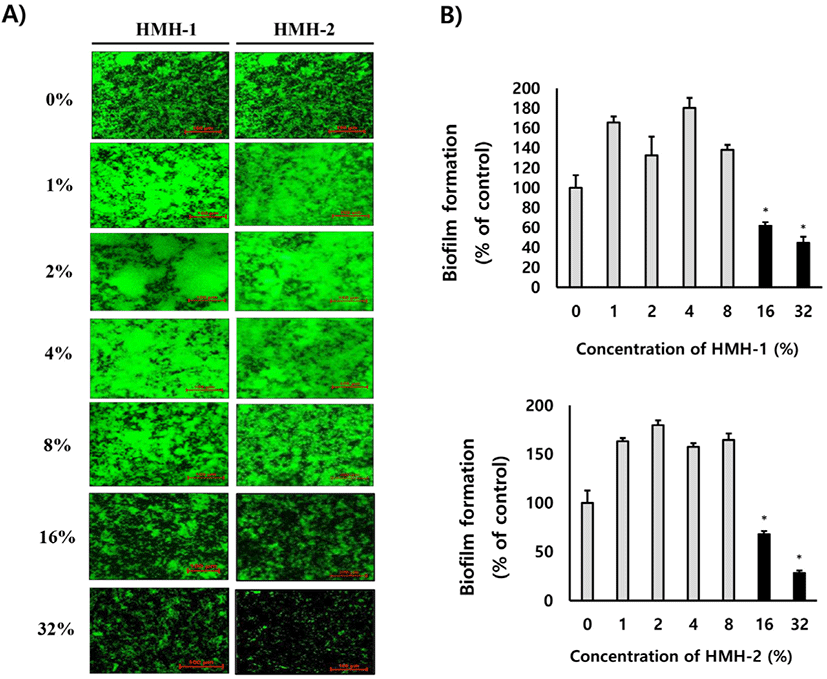
Since the HMH showed inhibitory activity on E. faecalis biofilm formation and also exhibited stimulatory properties on elimination of the E. faecalis biofilm (Fig. 2), we then examined the effects of HMH on the viability and EPSs production of E. faecalis. As shown in Fig. 3, administration of HMH on the planktonic growth of E. faecalis (which was estimated by measuring the optical density at 2, 4, and 6 h after inoculation) dose-dependently attenuated the growth of E. faecalis. The inhibitory activity of HMH was further examined using a WST-1 cell viability assay kit at 24 h after inoculation. About 60% and 80% of the E. faecalis population was decreased when 16% and 32% of HMH was co-incubated with E. faecalis, respectively. However, the production of EPSs, which is considered an important factor in the formation of E. faecalis-mediated biofilm, was not affected by HMH treatment. These data indicate that the HMHs’ attenuation of the biofilm formation of E. faecalis was a result of the growth-inhibitory effects of the HMH but not that of EPS production suppression effect of HMH.
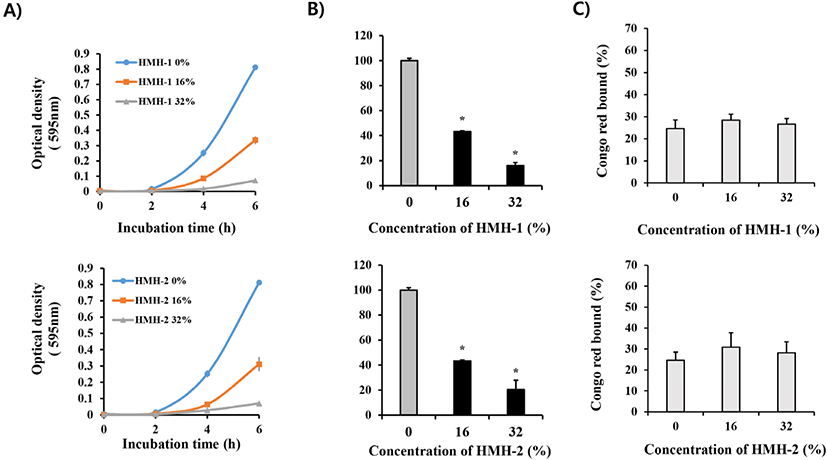
To investigate the effects of the HMHs on the E. faecalis-mediated inflammation in gastrointestinal epithelial cells, the inhibitory properties of the HMH on the E. faecalis-mediated induction of a proinflammatory cytokine, IL-8, was estimated by using HT-29 cells, which have been used as an in-vitro model of intestinal epithelial cells (Lee et al., 2010). At first, the cytotoxicity of the HMH in the HT-29 cells was evaluated. As shown in Fig. 4A, treatment of HMHs did not show cytotoxicity in HT-29 cells up to 2 mg/mL concentrations. Based on these data, non-toxicological levels of HMHs (0.1–0.2 mg/mL) were used for estimating the anti-inflammatory properties against E. faecalis in HT-29 cells. We then investigated whether or not HMHs can ameliorate the E. faecalis-mediated expression of the proinflammatory cytokine IL-8 in HT-29 cells. As shown in Fig. 4B and 4C, administration of E. faecalis to HT-29 cells stimulated IL-8 expression when compared with that of the control (non-treated). However, pretreatment of HMHs (HMH-1 and HMH-2) abolished the E. faecalis-induced IL-8 expression in HT-29 cells, suggesting that HMHs can prevent E. faecalis-mediated inflammation through attenuating the E. faecalis-induced expression of the proinflammatory cytokine IL-8.
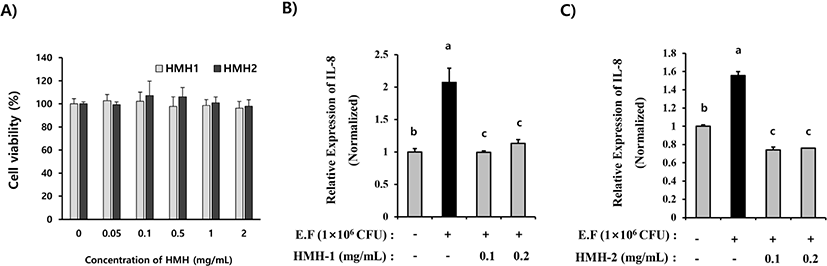
To further elucidate the action mechanisms of the HMH-mediated anti-inflammatory responses on the gastrointestinal track, the expression of Toll-like receptor-2 (TLR-2), which plays an important role in transmitting the E. faecalis-mediated inflammatory signal, and a TLR-2 adaptor protein, MyD88, were investigated. As shown in Fig. 5, administration of E. faecalis to HT-29 cells significantly (p<0.05) increased the expression of TLR-2 as well as MyD88 when compared with that of the control (non-treated). Interestingly, those up-regulations disappeared in HT-29 cells treated with HMHs, suggesting that HMH could be used for attenuating the E. faecalis-mediated activation of TLR-2 related inflammatory signal transduction in HT-29 cells. Moreover, we found that administration of E. faecalis to HT-29 cells stimulated the phosphorylation of mitogen-activated protein kinases (MAPKs; ERK, p38, and JNK), which is a downstream signaling pathway of TLR-2, and it was significantly (p<0.05) attenuated by HMH administration (0.2 mg/mL; Fig. 5A and 5D). These results indicate that HMH could inhibit the E. faecalis-induced intracellular over-activation/phosphorylation of MAPKs, which is a critical signaling pathway that transduces the TLR-2 mediated inflammatory signals. Taken together, our data suggest that HMH could effectively ameliorate E. faecalis-mediated inflammatory responses through regulating the TLR-2/MyD88/MAPKs signaling pathways in HT-29 cells.
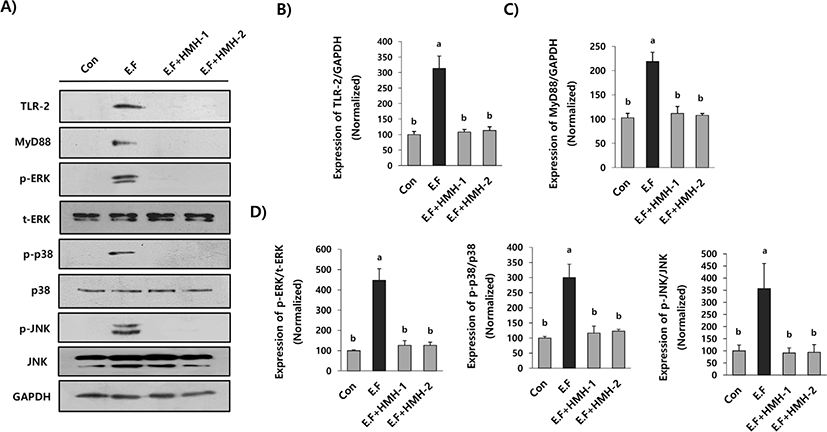
Since TLR-2 is known as a key regulatory receptor protein that regulates pathogen-mediated inflammatory responses, TLR-2 binding molecules have been targeted for developing anti-inflammatory agents. MyD88 is an adaptor molecule that binds TLRs, thereby regulating the inflammatory responses. Therefore, finally, we compared the anti-inflammatory efficacy of HMH with that of the MyD88 inhibitor in HT-29 cells. As shown in Fig. 6, although the expression of IL-8 did not increase when HMH (0.2 mg/mL), a control inhibitor (5 μM), or the MyD88 inhibitor (5 μM) were administered to the HT-29 cell, it was dramatically increased if E. faecalis was administered to HT-29 cells. Interestingly, E. faecalis-mediated IL-8 expression was significantly (p<0.05) attenuated by pretreatment of the MyD88 inhibitor as well as HMHs. Furthermore, we found that 5 μM of the MyD88 inhibitor and 0.2 mg/mL of HMH has an equal level of efficacy in suppressing E. faecalis-induced IL-8 expression in HT-29 cells. These results suggest that MyD88 plays a critical role in E. faecalis-induced inflammatory responses in HT-29 cells, and support the anti-inflammatory effects of HMH whereby E. faecalis-mediated inflammatory responses could be effectively attenuated by HMH through controlling the expression of MyD88 in HT-29 cells.
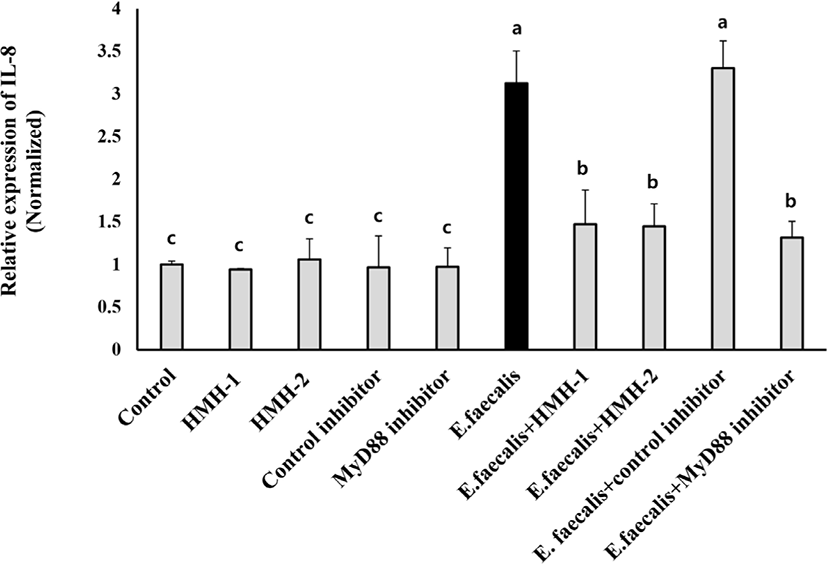
Discussion
Foodborne disease is often induced by bacterial contamination from bacterial biofilms that are located in food processing plants (Liu et al., 2013; Pesavento et al., 2014). In addition, chronic wounds, which induce severe inflammatory responses, contain bacterial populations as a form of biofilms (Fux et al., 2005). The reason why chronic inflammation has problems in treating with antibiotics is because bacterial biofilms are more resistant to antibiotics that planktonic bacteria (Ngo et al., 2012). Thus, developing effective ways to eliminate existing biofilms and suppress newly formed biofilms may be an important means of attenuating foodborne diseases and chronic inflammatory responses. Therefore, in this study, we investigated whether or not HMH could effectively attenuate E. faecalis-mediated biofilm formation. In addition, the regulatory roles of HMH on E. faecalis-induced inflammatory responses in HT-29 cells were firstly evaluated.
Honey is a potential nutraceutical food and has also been recognized as a traditional medicine for curing chronic wounds and burns (Majtan, 2014). Since antimicrobial activity is a representative biological property of honey, anti-bacterial and anti-biofilm activities of several monofloral honeys have been investigated (Kim and Kang, 2020; Lu et al., 2014; Lu et al., 2019). Each monofloral honey is made by honeybees using distinct floral sources; therefore, the content and types of phenolic acids are different, and specific ingredients such as methylglyoxalate (MGO), which has a strong anti-bacterial effect, could be included in specific monofloral honeys, thereby showing distinct anti-bacterial activities among monofloral honeys (Lu et al., 2019).
Since honeybees can access honey plants that are far away to produce honey, many types of pollen are often detected even in monofloral honey. Therefore, in general, honey can be recognized as monofloral honey if the content of major pollen is more than 45% and, if the content of major pollen is increased, the characteristics of the monofloral honey could be clearer (Alvarez-Suarez et al., 2010). The high-purity HMHs that were used in this study were produced by using a net house system; they contained more than 95% single pollen derived from the Hovenia tree (Park et al., 2020). We therefore think the HMHs used in this study are a good source for evaluating the honey potential of HMH. The antioxidant and anti-bacterial properties of HMH were first reported in our previous study (Park et al., 2020) and this study is the second paper to report the functionality of HMH. We found that HMHs can attenuate E. faecalis-mediated biofilm formation and stimulate the destruction of E. faecalis biofilms, indicating that HMH could be used for inhibiting E. faecalis-induced foodborne disease and chronic inflammation. We also found that the planktonic growth of E. faecalis was dramatically attenuated by HMH administration, but the EPS formation of E. faecalis was not affected by HMH. Thus, these results suggest that HMH-mediated attenuation or stimulation of E. faecalis biofilm formation and elimination could come from the killing effects of HMH on E. faecalis.
Although E. faecalis is a common bacteria in the gastrointestinal track, it has been known to induce pathological disorders such as endocarditis and root canal infection (Ch'ng et al., 2019). In addition, food-contaminated E. faecalis may enter the gastrointestinal track and cause an inflammatory reaction. It has also been reported that E. faecalis is able to stimulate reactive oxygen species (ROS) production in gastrointestinal epithelial cells as well as colonic epithelia cells, which can induce epithelial cell damage (Rokutan et al., 2006; Strickertsson et al., 2013; Wang et al., 2008). In addition, E. faecalis infection was reported to be associated with Crohn’s disease, a type of severe inflammatory disease (Zhou et al., 2016). Moreover, administration of E. faecalis to MKN74 gastric adenocarcinoma cells up-regulated proinflammatory cytokines such as tumor necrosis factor-α and IL-8. These previous reports suggest that E. faecalis could directly induce inflammatory responses in gastrointestinal epithelial cells. In addition, the detailed molecular mechanisms of how E. faecalis transduces inflammatory signals to produce proinflammatory cytokines in gastrointestinal epithelial cells still need to be elucidated.
In our study, we demonstrated that administration of E. faecalis to HT-29 cells stimulated the expression of a proinflammtory cytokine, IL-8, and it was controlled by TLR-2 mediated signaling. The expression of TLR-2 and its adaptor protein MyD88 was increased when E. faecalis was administered to HT-29 cells. In addition, activation of MAPKs, a downstream signaling pathway of TLR-2, was shown when E. faecalis was administered to HT-29 cells. Furthermore, we found that E. faecalis-mediated expression of IL-8 was abolished by administration of a MyD88 specific inhibitor. These results strongly suggest that E. faecalis could induce the inflammatory response in HT-29 cells through activation of a TLR-2 mediated signaling pathway. Moreover, our results demonstrate that this E. faecalis-mediated TLR-2 mediated inflammatory signaling could be attenuated by HMH treatments.
Various studies have suggested the anti-inflammatory activity of honey. Indeed, it was reported that clinical use of honey on wounds helps to inhibit infection (Armon, 1980; Phuapradit and Saropala, 1992) and rapid rates of healing were seen on wounds dressed with honey (Bergman et al., 1983; Hejase et al., 1996). In addition, many studies have reported that honey can inhibit inflammation through inhibition of ROS production and cyclooxygenase-2 (COX-2) expression (Hussein et al., 2012; van den Berg et al., 2008). Although the effects of HMHs on the expression of ROS and COX-2 in HT-29 cells was not examined in this study, anti-inflammatory properties of HMHs against E. faecalis in HT-29 cells was clearly demonstrated. Administration of HMHs to HT-29 cells effectively suppressed the adhesion of E. faecalis to HT-29 cells and thereby attenuated the E. faecalis-mediated TLR-2 inflammatory signaling. Furthermore, our comparative study showed that 0.2 mg/mL of HMH and 5 μM of the MyD88 inhibitor had equal efficacy in inhibiting IL-8 production by E. faecalis treatment. MyD88 is well-known as an intracellular adaptor protein of TLRs that plays a critical role in connecting TLRs and intracellular proteins to transmit signals from TLRs. Since TLRs-mediated signaling is closely involved in the progression of various diseases, the MyD88 protein has been targeted as a therapeutic target and, actually, several MyD88 inhibitors have been developed to treat inflammation-related diseases such as atherosclerosis, cancer, and leukaemia (Chen et al., 2019; Liu et al., 2020; Shiratori et al., 2017). Therefore, the results of our comparative study strongly support that HMS could effectively inhibit E. faecalis-mediated inflammatory responses in HT-29 cells.
Conclusion
Collectively, the effects of HMH on E. faecalis-mediated biofilm formation and the inflammatory response in HT-29 cells were firstly evaluated in this study. We found that HMH effectively attenuated E. faecalis-mediated biofilm formation by attenuating the growth of E. faecalis. In addition, we found that HMH could effectively inhibit the E. faecalis-mediated inflammatory responses in HT-29 cells through controlling the TLR-2/MyD88/MAPKs pathway. Taken together, these data suggest that HMH could be used to develop an antimicrobial agent for preventing E. faecalis-mediated inflammatory diseases.