Introduction
Memory refers to the brain’s ability to store and retrieve information from past experiences. Memory is largely divided into short-term memory and long-term memory. Short-term memory is the ability to store information for a short time, while long-term memory is the ability to remember information for a long period of time. Cognition is the ability of the brain to process and understand information through perception, cognition, reasoning, and memory. Cognitive ability is largely composed of information processing speed, information storage and retrieval ability, and reasoning ability. Cognition is related to the overall function of the brain and is affected by various factors, such as brain structure, neural circuits, and brain waves. When brain activity is performed smoothly, cognitive ability increases; on the contrary, cognitive ability decreases when brain activity decreases through neuron apoptosis. This loss of cognition and memory is a key early symptom of neurodegenerative disease (Bishop et al., 2010; Skvarc et al., 2018).
Inflammation is a biological response usually caused by injury, infection, or irritation and, at the appropriate level, plays an important role in the protective and repair processes. The main function of inflammation is to detect, contain, and eliminate the cause of injury and damaged tissues in the body and activate the immune system to augment the infiltration of inflammatory molecules and immune cells, such as antibodies and lymphocytes, to initiate tissue repair. The important proteins used in this process are cytokines. Cytokines are proteins secreted by immune cells that mediate various physiological actions related to inflammation. Some cytokines promote the production of prostaglandins and leukotrienes, chemicals associated with inflammation, and some promote the activation of the inflammatory mediator, nuclear factor kappa-light-chain-enhancer of the activated B cells. These actions trigger an immune response that reduces further tissue damage and mitigates cellular damage.
However, excessive cytokine secretion is associated with excessive activation of the inflammatory response, which is implicated in immune system diseases and inflammatory diseases (Dik et al., 2005). Among cytokines, pro-inflammatory cytokines, such as interleukin (IL)-1, IL-6, and tumor necrosis factor-alpha (TNF-α), directly affect the brain and can promote inflammatory responses inside the brain (Allison and Ditor, 2014). In the brain, these pro-inflammatory cytokines are also implicated in brain diseases, such as senile dementia, Parkinson’s disease, Alzheimer’s disease, and depression (Konieczna et al., 2012). Thus, suppressing inflammatory responses by inhibiting cytokine secretion is important for protecting brain health and function.
Studies have shown that injection of lipopolysaccharide (LPS) in mice can induce neuroinflammation and cause cognitive impairment (Kim et al., 2022). LPS is one of the cell wall constituents of Gram-negative bacteria. It is of large molecular size and a potent stimulant of the immune response. LPS activates Toll-like receptors on immune cells (Lee et al., 2008). Downstream, pro-inflammatory cytokines, such as IL-1β and TNF-α, are secreted, and caspase 1 is activated. This excessive inflammatory response causes cell death (Zhao et al., 2019). Neuronal cell death is associated with cognitive deficits and memory loss.
Excessive inflammatory response and oxidative stress are considered as leading causes of cell death. Oxidative stress is a condition that results from the production of free radicals due to oxidative metabolism. Cells are equipped with antioxidant mechanisms to respond to oxidative stress, but if oxidative stress persists, antioxidant mechanisms can no longer adapt and trigger death-inducing signals within the cell (Solis et al., 2002). Apoptosis is one of the cell death processes caused by oxidative stress. Apoptosis is programmed cell death caused by regulatory mechanisms inside the cell (Guan et al., 2015).
Mitochondrial oxidative stress accelerates mitochondrial free radical production and causes oxidative overload. In this state, cellular components, such as protein, DNA, and mitochondria, are damaged, inducing apoptosis. Apoptosis is tightly regulated to ensure the safe elimination of damaged cells while sparing nearby healthy cells (Ishihara et al., 2015). However, excessive oxidative stress can lead to excessive apoptosis. Many pathological conditions (e.g., neurodegenerative diseases) feature excessive apoptosis (Leszek et al., 2016). Therefore, it is important to prevent oxidative stress by consuming dietary antioxidants and to prevent cell damage and death (Czekus et al., 2022).
According to recent studies, the intake of specific probiotics can improve various diseases, such as sepsis, cancer, and neurodegeneration, through antioxidant and anti-inflammatory mechanisms (Liang et al., 2015; Rumman et al., 2021; Wang et al., 2020a; Wang et al., 2020b; Wang et al., 2021). Contrary to past predictions that probiotics would not affect the brain, the emergence of the gut brain axis hypothesis (Foster et al., 2017) that changes in intestinal microbes affect the brain implies the potential of probiotics as a therapeutic agent for cranial nerve diseases.
Lactic acid bacteria (LAB) and Bifidobacterium are the most well-known probiotic genera and have been extensively studied for their potential health benefits. Mixed cultures of LAB and Bifidobacterium strains have been shown to have a synergistic effect on the host’s health, leading to increased production of short-chain fatty acids and modulation of the immune system. LAB and Bifidobacterium used in this study will play a major role in the development of dairy food products in dairy second products and various applications.
The aim of this study was to investigate the potential health benefits of mixed cultures of probiotics that can improve cognition and memory through their antioxidant and anti-inflammatory properties. To achieve this, we administered LPS to BALB/c mice and assessed the impact of probiotics intake on cognition. Additionally, we measured the levels of IL-1 and nitric oxide (NO) in the blood and analyzed antioxidant indicators through cell experiments to compare and evaluate the efficacy of these probiotics.
Materials and Methods
Probiotic strains containing Bifidobacterium animalis KL101 (KL101), Levilactobacillus brevis KL251 (KL251), and Limosilactobacillus fermentum KL271 (KL271) were obtained from Kolab, Inc. (Gwangju, Korea). KL101 was grown in BL (blood and liver) broth using an anaerobic system (MACS 1000, Don Whitley Scientific, Shipley, UK) at 37°C for 48 h. The strains of KL251 and KL271 were grown in de Man Rogosa Sharpe (MRS) broth (BD Biosciences, Sparks, MD, USA) at 37°C for 24 h. The bacterial cultures were washed with sterile phosphate-buffered saline (PBS) and centrifuged at 3,000×g for 15 min at 4°C. The bacterial pellets were resuspended in sterile PBS to a concentration of ca. 1×109 CFU/mL and heated at 90°C for 20 min to heat-kill the samples.
For the animal experiments, we used BALB/c 8-week-old female mice with an average body weight of 19 g. During the 1 wk adaptation, sufficient water and feed were consumed autonomously. The animals were housed in individual cages in a room with a temperature of 23±2°C and 55±15% RH, under a 12 h light/12 h dark cycle, light 200 300 lux 12 h/day, and noise maintained at 40 dB or less. All experiments were conducted following the policy of the Laboratory Animal Ethics Committee and regulations related to animal testing (approval number: CNU IACUC-YB-2016-07). After the adaptation period, mice in the treatment group were administered each sample of Probiotics orally at the same time once a day for 15 d with a final concentration of 1×109 CFU, and mice in the control group were administered orally deionized water. All mice were orally administered probiotics with a final concentration of 1×109 CFU on day 7, and short-term memory was measured on day 7 after learning the Morris water maze (MWM) for 6 d. Before the short-term memory measurement, 1 mg/kg of LPS was administered intraperitoneal (i.p.) injection to mice excluding the control group. The body weight, feed intake, water intake, and excretion every 24 h of the experimental animals were recorded. In order to compare physical changes according to the probiotics administered, physical weight was regularly measured at the same time and in the same order using a scale. All measurements in the experimental animals were conducted in a quiet environment to prevent exposure to stress.
During oral administration, all mice groups repeatedly learned the platform location of the MWM, three times a day for 6 d, and on day 7, LPS was i.p. to the negative control (NC) group and the experimental group, excluding the positive control group, to cause intentional cognitive decline, and finally, short-term memory was measured. The platform was removed, and the animals were allowed to swim for 1 min to evaluate retention memory. To perform the MWM test, cephalopod ink was used to make the water opaque, and the water temperature was 24±2°C. If a mouse did not find the platform, the animal was placed on the platform and left for about 20 s. The final MWM test was conducted to measure how accurately the mouse remembered the location of the platform after removing the platform.
Mouse blood collection was carried out on day 16, twenty-four hours after 1 mg/kg LPS i.p., and after all oral administration and MWM experiments. Blood was collected from the jugular vein and in isolation from all other mice to minimize stress in experimental animals. Before blood collection, the blood collection area was sterilized with 70% alcohol to remove contamination on the skin surface. After blood collection, the blood collection site was disinfected with 70% alcohol to prevent infection of mice and pressed for more than 30 s to prevent hematoma formation. Blood was collected using 3.8% sodium citrate buffer, an anticoagulant, to prevent blood clotting. Immediately, the blood was centrifuged at 1,200×g for 10 min at 4°C to separate the plasma for subsequent analysis.
The levels of IL-1β in plasma were measured by ELISA using antibodies and the IL-1β Mouse ELISA Kit (Abcam, Cambridge, UK) according to the manufacturer’s instructions. The kit was stored at 20°C before use. For the assay, 100 μL of diluted plasma and standard were put into separate 96 wells and incubated at room temperature for 2.5 h with gentle shaking. Following incubation, wash buffer was added to remove unbound proteins, and 100 μL of 1X biotinylated-IL-1β was added to each well and incubated for 1 h. After incubation, wash buffer was added to remove the unbound antibody, and then 100 μL of HRP-streptavidin solution was added to each well and incubated at room temperature with gentle shaking for 45 min. Subsequent to the washing process, the 3,3′,5,5′‐tetramethylbenzidine (TMB) one-step substrate agent was put into each well and incubated at room temperature with gentle shaking for 30 min, after which stop solution was added, and the absorbance was immediately measured at 450 nm. The color change developed in proportion to the amount of IL-1β. Quantification was performed by interpolation of the standard curve.
Nitrite (NO2-), a stable end product of NO degradation, was assayed using the Griess colorimetric reaction. The method is based on a chemical reaction using sulfanilamide and N-1-naphthyl ethylenediamine dihydrochloride (NED) under acidic (phosphoric acid) conditions and has been used to detect NO2- in various biological and experimental samples, such as plasma, serum, urine, and tissue culture media. Briefly, plasma was added to the well in the same amount as the nitrite standard (0 100 μM), which was assayed simultaneously. After adding 50 μL of sulfanilamide solution into all wells, the plate was incubated at room temperature for 10 min while avoiding light. Next, 50 μL of NED solution was added to each well and incubated at room temperature for 10 min while avoiding light. The absorbance of the azo chromophore developed by the reaction of NO2- with sulfanilamide was measured at 540 nm. All the standard and reagent solutions were stored at 4°C in a refrigerator while not in use.
Results
Figs. 1A and B indicate to confirm the results of co-treatment with probiotics on the oxidative stress induced by LPS with the relative expression levels of Gclm (glutamate-cysteine ligase modifier subunit), Gclc (glutamate-cysteine ligase catalytic subunit), and Gpx1 (glutathione peroxidase 1), Cat (catalase), Sod1 (superoxide dismutase 1), and Sod2 (superoxide dismutase 2) were confirmed. Fig. 1A shows that KL251 treatment results and Fig. 1B shows that KL271 treatment results.
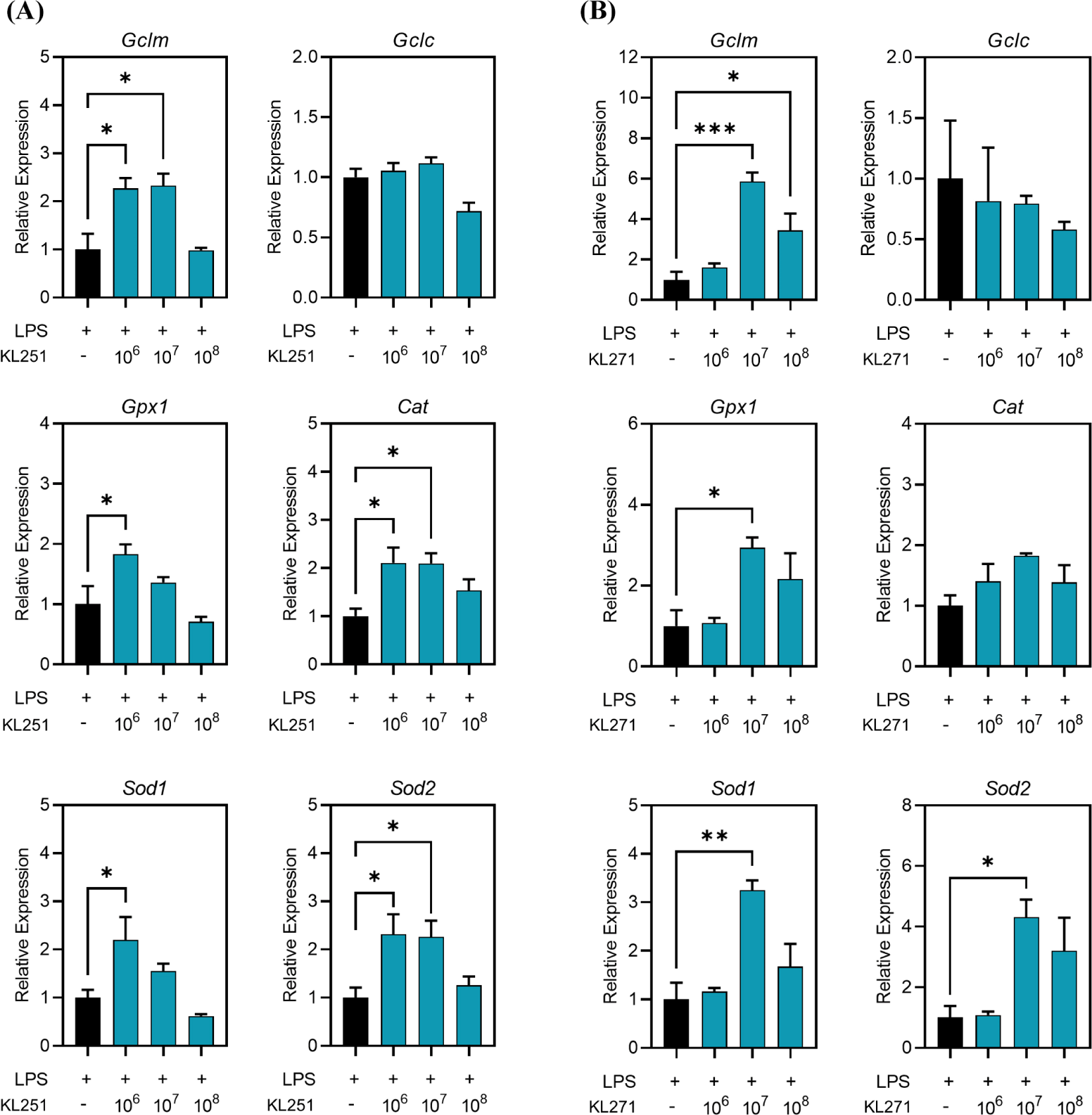
Fig. 2A represents a schematic diagram of the overall experiment schedule and defined groups. To compare the physical changes according to oral administration of probiotic samples, all mice were weighed at the same time every day during the experiment period (Figs. 2B, C, D, and E). Additionally, food, water intake and excretion were recorded every 24 h. As a result of weighing mice for a total of 17 days, there was no difference between all groups before LPS treatment. After i.p. administration of LPS on day 15, it was confirmed that all LPS-treated mice (average change in body weight=–2.5±0.19 g) except for the control group (average change in body weight=0.3±0.33 g) lost weight. By contrast, the group administrated with KL101 had less weight loss (–1.75±0.48 g) than the other groups. These results suggest that intake of KL101 can prevent weight loss caused by the LPS-induced inflammatory response.
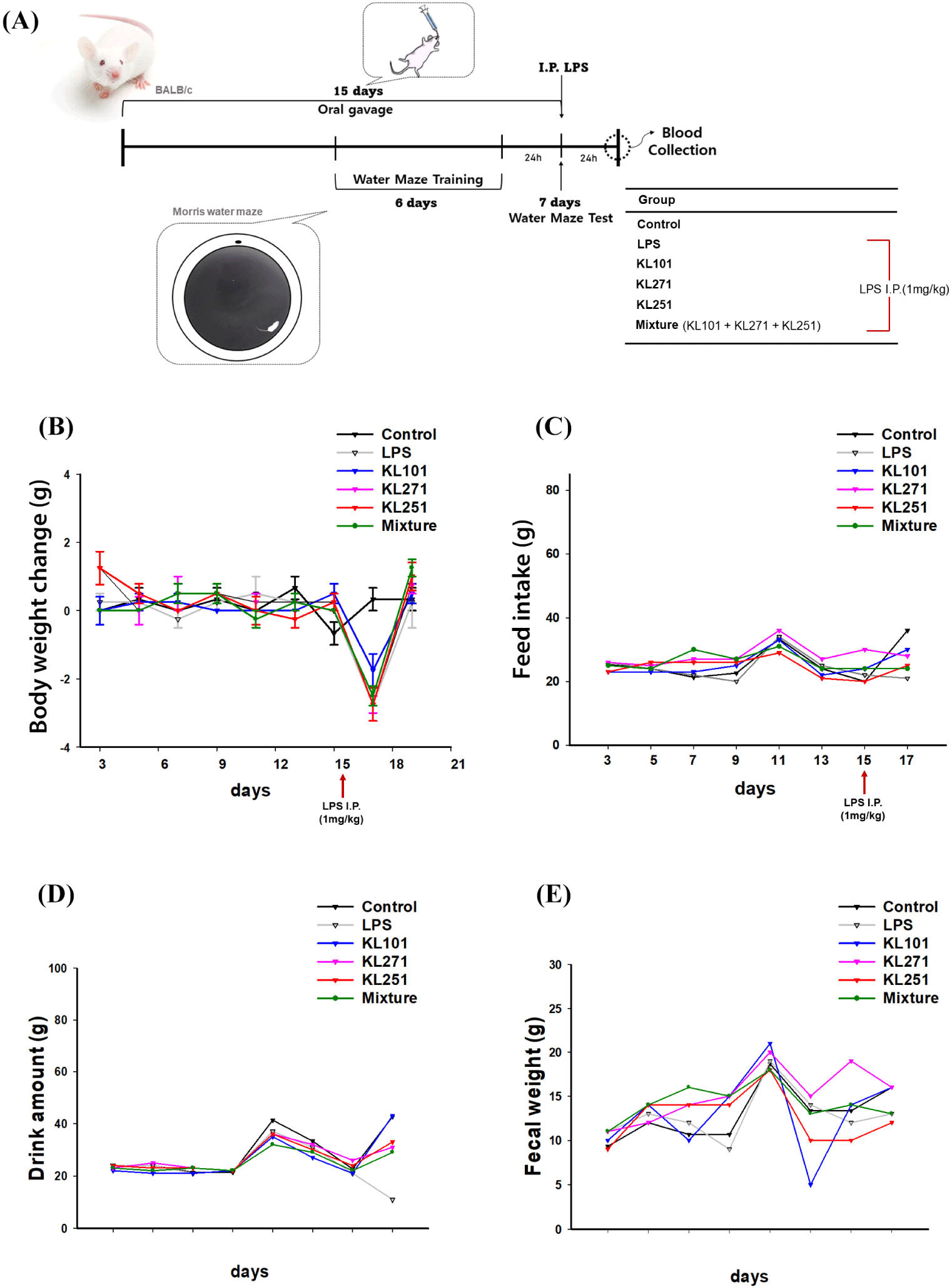
The MWM test was performed to investigate the effect of intake of probiotic samples on spatial learning ability and spatial memory. In the MWM test, the animal is required to locate a hidden platform in order to escape from swimming in a pool of water. All mice were taught the platform position three times a day for 6 days. In the final test, the test was conducted 30 min after i.p. of LPS. The platform was removed, and how accurately the mouse memorized the position of the platform was measured to evaluate spatial memory and cognitive ability between groups. Fig. 3A shows the location of each zone in the MWM. It was expected that the better the mouse memorized the location of the platform, the more time it would spend in the SW area searching for the removed platform and the more times it would pass through the removed platform position (Figs. 3C and D). Additionally, the path to reach the platform for the first time (latency map) and overall movement (activity map) were compared. As a result, compared to the control group not treated with LPS, the time spent in the SW area searching for the removed platform decreased, but in the KL251 and mixture group (probiotic mix: KL101+KL271+ KL251), similar paths and movement lines to those of the control group were confirmed (Figs. 4A, B, and C). As a result of the MWM test, it was confirmed that there was a significant difference in the time, ratio, and the number of times (target crossing) of staying on the platform in the probiotic mixture intake mouse group compared to other groups treated with LPS (p<0.05). This result suggests that intake of the probiotic mixture has an effect of suppressing the decrease in memory and cognition induced by LPS treatment. Detailed results of the MWM test are shown in Table 1.
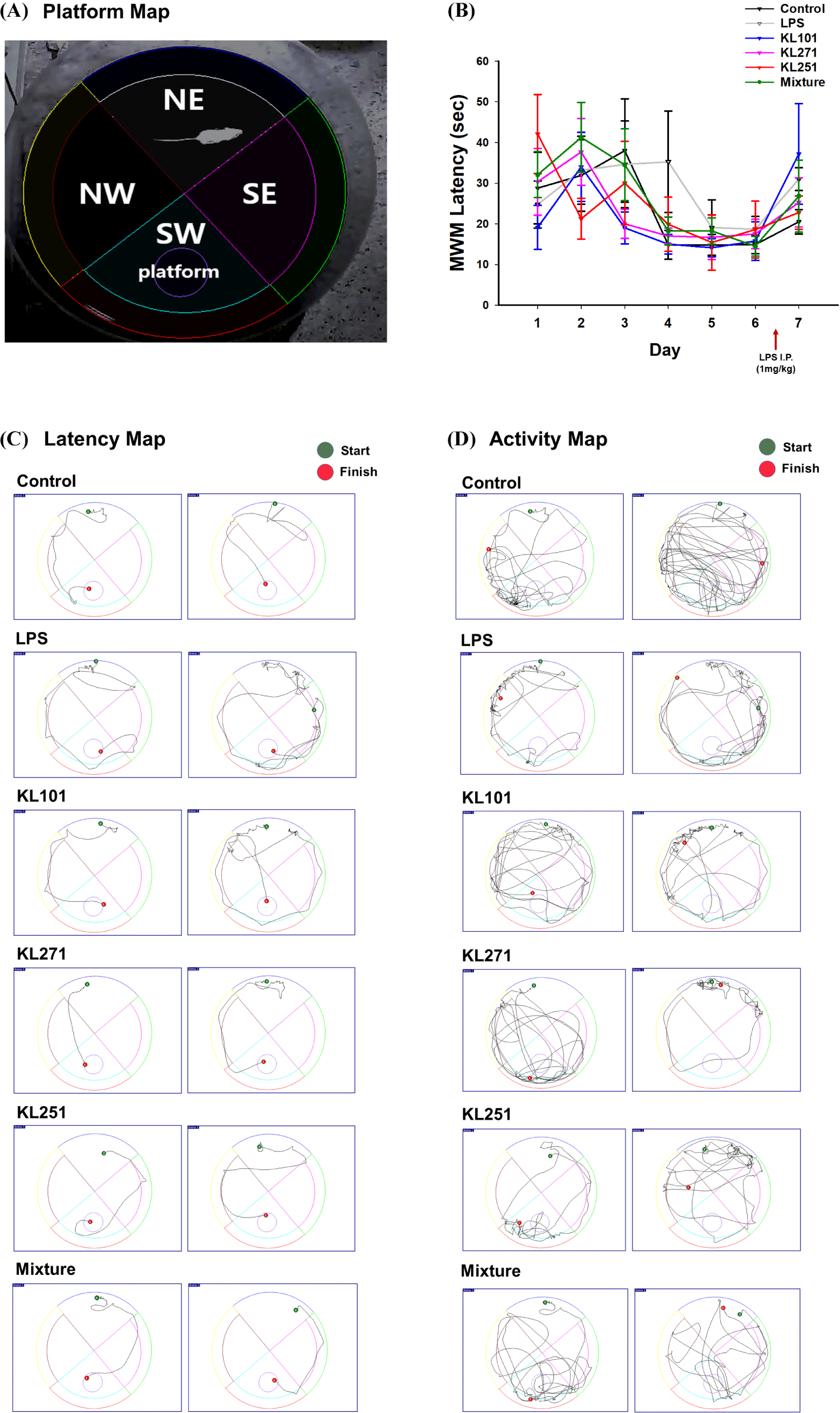
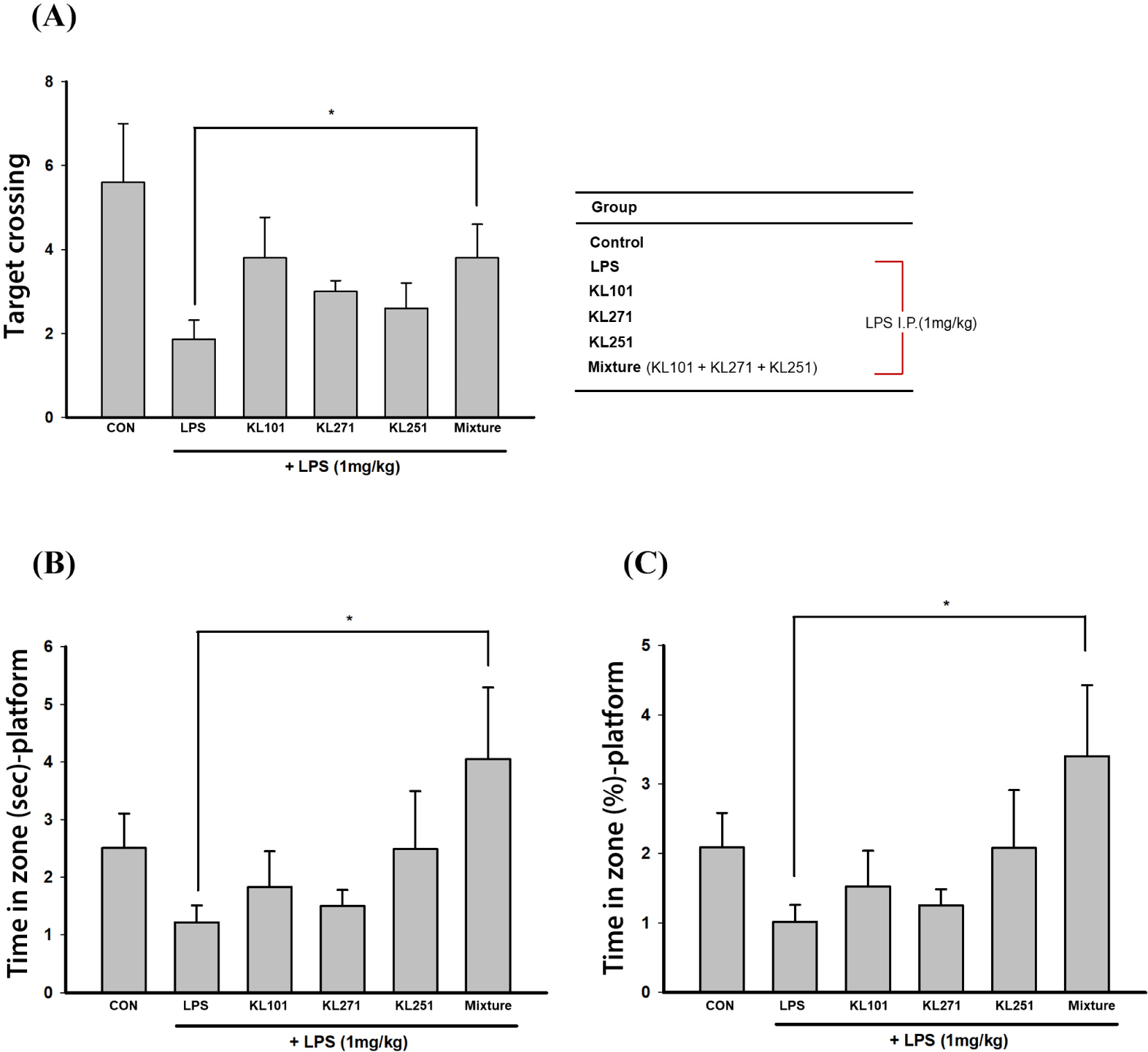
NO is an important physiological messenger in many biological systems, including immune, neural, and cardiovascular tissues. Because it is involved in these diverse systems, NO levels in biological tissues and body fluids can be used as a versatile biomarker. On day 15 of the experiment, all mice except the control group were i.p. injected with LPS. Twenty-four hours later, on day 16 of the experiment, blood was collected from all mice, including the control group. Plasma was separated from the collected blood (Fig. 5A). As a result of measuring the amount of NO induced by LPS injection, the NO concentration detected in the LPS treatment groups differed from that detected in the control group. Furthermore, a significantly lower concentration of NO was detected in the KL101 group (43.06±1.98 μM, p<0.05) compared to the NC group (52.54±3.75 μM). The lower concentration of NO detected in the KL101 group suggests that intake of KL101 can suppress the inflammatory response induced by LPS.
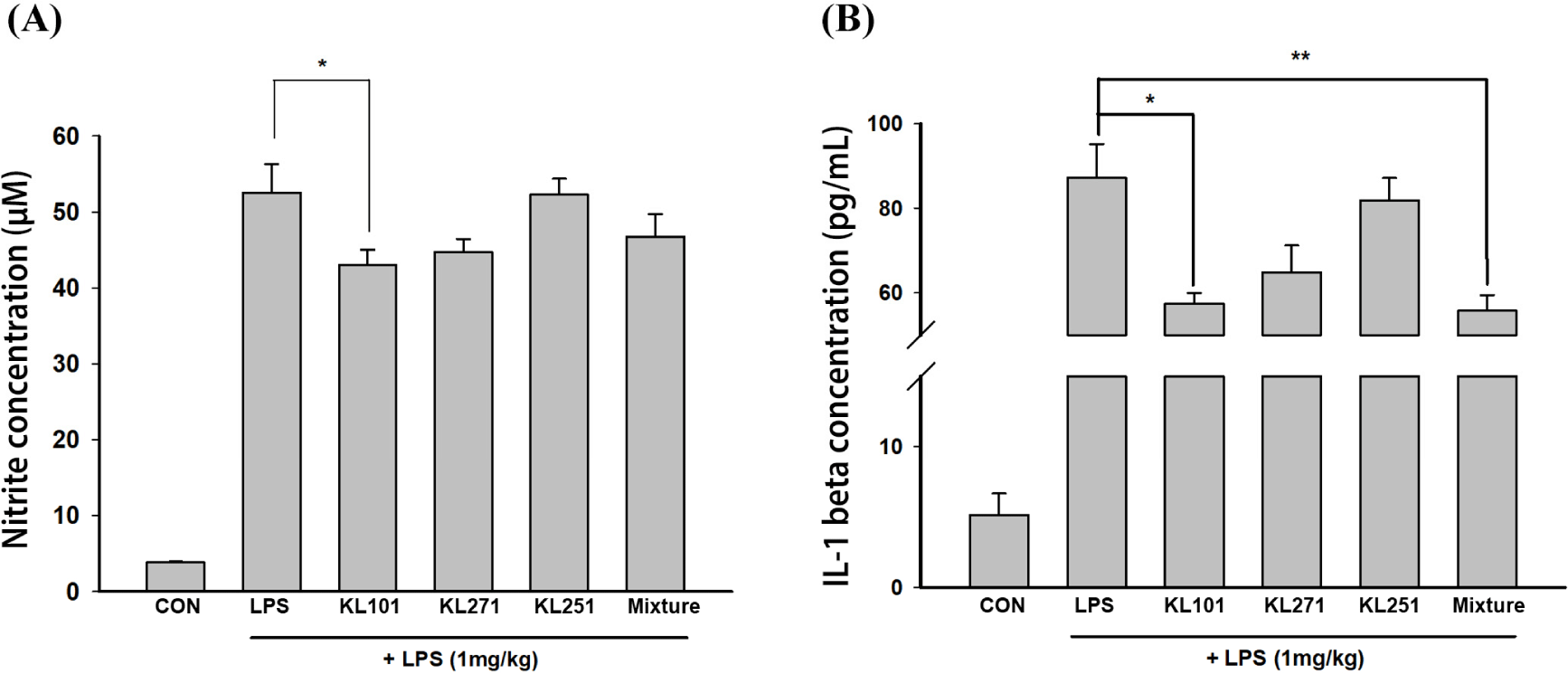
IL-1β is a potent proinflammatory cytokine important in defense responses to infection and injury. On day 15, LPS i.p. injection, excluding the control group, could have induced the expression of pro-inflammatory cytokines, such as TNFα, IL-6, and IL-1β. On day 16, 24 h after LPS injection, blood was collected from all mice, including the control group, and the plasma was separated for IL-1β ELISA (Fig. 5B). As a result of the IL-1β ELISA, a significant concentration of IL-1β was detected in the LPS-treated groups compared to the control group. Meanwhile, IL-1β levels in the KL101 group (57.38±2.59 pg/mL, p<0.05) and the mixture group (55.77±3.75 pg/mL, p<0.01) were detected at a significantly lower concentration than in the NC group (87.19±8.0 pg/mL). The decrease in the concentration of IL-1β, a pro-inflammatory cytokine, suggests that intake of KL101 and the probiotic mixture can suppress the inflammatory response induced by LPS treatment.
Discussion
This paper investigates probiotics that can improve LPS-induced inflammatory cognitive impairment. The changes in IL-1 concentration and NO concentration in the blood of BALB/c mice according to the intake of probiotics were measured, and finally, whether the administration of probiotics had improved cognition was tested through the MWM. According to the results of this study, it means that consumption of probiotics has the effect of preventing cognitive decline induced by LPS i.p. injection.
Above all, improved results were measured in several indicators of the MWM experiment that measure memory. In latency, target platform crossing, and percentage located in the target quadrant, the mice that received probiotics showed more platform memory values than the mice that only received LPS. Looking closely at the traces of mice, which indicate the traces of memory for the platform location, it can be seen that the traces of mice that received probiotics arrived at the target through several direction changes. This can be considered as an improvement in the loss of memory. Additionally, evidence of remembering the location of a platform that has disappeared from more clearly is that you visit the vicinity more often. In the MWM memory test, it can be confirmed that the movement line of the mice that ingested probiotics stayed near the platform compared to those injected only with LPS.
It has been proven through several previous studies that memory and cognitive loss are induced by inflammation following LPS injection (Kim et al., 2022; Lee et al., 2008). In our experiment, we injected LPS into the peritoneal cavity, not the brain, which is an alternative and less invasive method to elicit a neuroinflammatory response in the brain using LPS. Multiple previous studies have directly confirmed this through experiments in which excessive inflammatory responses were observed as a result of the culture of the brains of mice injected with LPS into the stomach, and various inflammatory markers were detected by immunohistochemistry. Therefore, the gut brain axis hypothesis that the gut and the brain are connected and interact with each other is supported (Cryan et al., 2019). Studies have demonstrated the importance of maintaining the balance of the intestinal microbiome to prevent or combat various diseases (Foster et al., 2017). In particular, it was announced in 2000 that diet-induced obesity in mice was associated with changes in the gut microbiome, and the obesity phenotype could be alleviated by restoration of the gut microbiota. Since then, various studies investigating the importance of the gut microbiome in various diseases, such as autism, allergy, diabetes, rheumatoid arthritis, and Parkinson’s, have become active (Magistrelli et al., 2019).
Among them, a number of studies have shown that changes in the intestinal microflora through the intake of probiotics are effective for several brain diseases, such as depression, anxiety, and Parkinson’s disease (Castelli et al., 2020; Liu et al., 2019; Magistrelli et al., 2019). Even despite the continuous flow of research on Alzheimer’s disease (Leblhuber et al., 2018), many therapeutics targeting amyloid-beta have failed recently, which has encouraged research focused on probiotics as a treatment (Cummings et al., 2018; Cummings et al., 2022). Accordingly, we also revealed some probiotics with potential as a treatment for Alzheimer’s disease. The results of the current study do not mean that the probiotics we discovered can be used immediately as a treatment. In order to find lactobacilli as an effective treatment, it is necessary to observe changes in the intestinal microflora before and after consumption of the probiotics and to conduct more precise studies on changes in metabolites. However, our discovery is meaningful because these new drug candidates are currently in short supply.
In conclusion, it was confirmed that the levels of NO and IL-1 in the blood of LPS-injected mice were significantly decreased, and cognitive evaluation using the MWM showed significant values in the latency and target quadrant percentages in the group that received probiotics. Antioxidant-related markers were examined at the molecular level, confirming that KL251 and KL271 had a positive effect on antioxidant-related factors. But in this result, the KL101-related expression data were excluded. Future studies are expected to provide better results by verifying the KL101 with other factors. These results provide that intake of these probiotics improves cognitive impairment and memory loss through anti-inflammatory and antioxidant mechanisms. Furthermore, these results are considered to be able to add strength to the study of inflammatory Alzheimer’s disease through the intake of probiotics, which is a field that currently requires much research.