Introduction
Nitrate (NO3) and nitrite (NO2) found naturally in the environment are also often utilized in several food products (Moorcroft et al., 2001). These incidentally discovered ions have been used to cure meats for centuries (Pierson et al., 1983). Before the discovery of the curing process, salt was used to preserve meat. However, improved preservation and reddish color development was noticed with a certain type of salt that contained an impurity known as saltpeter, which is a common name for nitrates (Binkerd and Kolari, 1975). Apart from cured meat color, nitrite, which is formed by reduction of nitrate, is also well known for improving aroma, flavor, antioxidant effect, and antimicrobial activity, particularly against pathogen Clostridium botulinum (Shahidi and Pegg, 1992; Xi et al., 2012). Despite these advantages, the use of nitrites in meat has been associated with carcinogenic nitrosamines (Sebranek et al., 2012; Sindelar and Milkowski, 2011). Concerns are further raised by occasional reports that link addition of nitrite into meat with cancer. Due to the lack of equivalent substitute for conventional sodium nitrites and nitrates (Gray et al., 1981), natural plant materials are being studied. Among various plants, vegetables such as lettuce, beets, radishes, spinach, and celery are known to contain high concentrations of nitrates as precursors of nitrite (Sindelar and Milkowski, 2012). Meat industry has exploited such rich natural sources of nitrate to produce cured meats.
To reduce nitrate to nitrite, starter cultures are included to result in the characteristic cured meat color and flavor (Møller et al., 2003; Sebranek et al., 2012). However, the reduction of nitrate to nitrite by microbes is both time-consuming and cost intensive. Moreover, traditionally cured meats were produced with age-old method using naturally present nitrate reducing bacteria to convert nitrate to nitrite (Cassens, 1990). For several years, celery (Apium graveolens var. dulce) due to its high nitrate and nitrite contents that has been utilized as a curing and flavoring agent in meat products (Bacus, 1984). Processors usually make use of celery powders (CPs) as a natural source of nitrate and nitrite in meat products since celery also has low vegetable pigment content along with mild flavor (Horsch, 2014; Sebranek and Bacus, 2007). Furthermore, other constituents of celery such as proteins, fibers, and carbohydrates can positively or negatively influence the formation of nitric acid (Djeri, 2010). Nitric acid in turn can affect the growth of pathogens such as Clostridium botulinum and Listeria monocytogenes (Horsch, 2014). Celery is also known to contain phenolic acids (such as ellagic acid and pyrogallol) and flavonoids (such as quercetin and hesperitin) that contribute to its antioxidant activities (Sorour et al., 2015). Therefore, this plant with its various constituents can serve many purposes in food applications. Another major consideration is the high demand for natural and organic products because they are perceived to be healthy (Djeri and Williams, 2014).
Extraction of bioactive compounds, nitrites and nitrates is commonly achieved via physical and chemical methods that increase production costs. Furthermore, chemical methods such as solvent extraction have demerits that include low extractability and toxic chemical remnants (Huang et al., 2006). Planetary ball-mill treatment is an environment friendly milling technique wherein materials are placed in a container along with balls (Khadka et al., 2014; Ramachandraiah and Chin, 2016). While various sizes and types of container and balls are available, stainless steel and ceramic are commonly used (Khadka et al., 2014). This low cost milling method is known to reduce the particle size of dietary fibers, thereby influencing its physicochemical properties (Liu et al., 2016). Particle size reduction also has several advantages that include improved extractability, solubility and bioavailability of important constituents (Khadka et al., 2014). Particle size reduction also affects other properties such as flowability, water holding capacity, and absorptivity, thus decreasing the amount of ingredients needed (Khadka et al., 2014; Merkus and Meesters, 2014). Although most studies involve the use of concentrate, extracts, or juices, no study has focused on the effect of particle size of CPs on physicochemical properties or antioxidant activities of meat products. Therefore, the objectives of this study were: i) to produce CPs of different particle size distributions by routine grinding and ball-milling, and ii) to evaluate the effect of particle size on physicochemical properties, curing efficiency, antimicrobial activity, and antioxidant activity.
Materials and Methods
Fresh celery (A. graveolens L. var. dulce) stalks were obtained from a local market, Gwangju, Korea. Cut celery stalks were cleaned and placed in a hot air oven at 100°C (LDO-250F, Labtech, Namyangju, Korea). Drying temperature of 100°C was decided based on our earlier studies (Ramachandraiah and Chin, 2017), which yielded higher antioxidant activities at this temperature compared to lower temperatures (50°C and 75°C).
Celery stalks dried at 100°C were pulverized using a 650 W kitchen (dry) grinder, passed through a sieve of 500 mesh to obtain coarse powder (C), and then passed through a sieve of 300 mesh to obtain micro-powders (M). These micro-powders were then subjected to ball-mill (planetary) treatment (Pulversette No. 6; Fritsch, Darmstadt, Germany) by placing powders with ZrO2 balls (6-mm diameter) and milling at 400 rpm for 24 h to form superfine powders (S). The weight of the balls was four time the weight of the sample. Intermittent milling was undertaken to avoid any overheating of the sample in the container. Particle size distributions of persimmon by-product powders were determined using a Malvern Mastersizer 2000 Particle Size Analyzer (Malvern Instrument, Malvern, UK) based on laser diffraction. These powders were dispersed in ethanol prior to size distribution measurement.
Pork sausages were manufactured using CPs (1%) with different granularity. Table 1 shows the formulation used in this study for the preparation of pork sausage batter. Pork hams were ground with a blender (K55, Crypto Peerless, Halifax, UK) for thirty seconds. Reference sausages were prepared by mincing the meat with sodium nitrite (NaNO2), salt (NaCl), and ice water. Similar to conventional sausages, a synthetic antioxidant, butylated hydroxytoluene (BHT), was also added to the reference sausages. Likewise, control sausages were prepared by homogenizing meat without nitrite and treatment sausages were added with CPs. CPs with different particle size added to the formulation were: coarse (T1), micro (T2), and superfine powder (T3). 1% of CPs was used as preliminary studies showed that increasing the amount led to unfavorable results. These homogenized meat batters were then packed under vacuum and held at 4°C for 2 h for proper equilibration of batters (Sebranek et al., 2012). Later, these batters were stuffed in casing (polyvinylidene chloride). Sausages were heated to have an internal temperature of 72°C using a water bath (WB-22, Daihan Scientific, Wonju, Korea). Cooked sausages were immediately chilled for 30 min. These sausages were then held at 4°C for 6 h before storing at 20°C. Quality analysis of vacuum-packed sausages stored at 20°C was performed every 3 days from 0 to 15 days.
A pH-meter (MP-120, Mettler-Toledo, Schwarzenbach, Switzerland) was used to measure pH values of meat samples. Sausage samples (10 g) were added to double distilled water (90 mL), blended 30 s and pH measurement was undertaken. Colorimetric assessment of the surface of each sausage was done using a model CR-10 color reader (Minolta, Tokyo, Japan). To determine the color values of the sausages, each sample was cut into 2 cm sections. Color determination was undertaken by placing the colorimeter perpendicular to the cut surface. Parameters analyzed were L* (lightness), a* (redness), and b* (yellowness) values. At least six measurements of each sausage were done for these parameters.
Quantification of residual nitrite was done by using the AOAC method (AOAC, 1990). Approximately 5 g of comminuted sample was initially added with some hot (80°C) distilled water. Lumps were broken up and the final volume was made to 300 mL. These samples were then placed in a hot (100°C) water bath for 1 h, filtered with Whatman No. 2 paper, and added with water to reach a final volume of 500 mL. Then 25 mL of filtrate was added to 2.5 mL of sulfanilamide and incubated for 5 min. After the incubation, 2.5 mL of N-(1-naphthyl) ethylene diamine (NED) was added and incubated for 15 min. These steps were repeated for nitrite standard solutions. A 0.5 g of sulfanilamide was dissolved in 150 mL of acetic acid (15% v/v). 0.2 g of N-(1-naphthyl)-ethylenediamine·2HCl was dissolved in 150 mL of acetic acid solution (15% v/v). The concentration of nitrite was measured using the standard curve.
Pigment contents of cured sausages were analyzed as described by Pietrasik et al. (2016). To approximately 2.0 g meat sample, 9.0 mL of acetone solvent (acetone diluted to 92.5% using distilled water) was added and mixed for a minute using a glass rod. Tubes containing the samples were incubated at room temperature for 10 min. Later, samples were filtered using Whatman paper No. 42 and the filtrate was analyzed at wavelength of 540 nm. A mixture of acetone and water (80:20) was used as blank. Quantification of nitrosyl hemochrome concentration was based on the obtained absorbance (A540×290) and recorded in parts per million (ppm).
Similar to pigment test for cured samples, 9.0 mL of acidified acetone solvent was added and mixed with 2.0 g meat sample. Acidified acetone solvent was prepared by mixing diluted acetone (92.5% in water) and concentrated HCl at a ratio of 2 to 1. Tubes containing samples were set aside at room temperature for 90 min. Following filtration with Whatman No. 42 paper, absorbance of the filtrate was measured at 640 nm. The blank was prepared the same as for pigment analysis of cured samples. Total pigment concentration was calculated as A640×680 and recorded in ppm.
TBARS determination was performed as described by Shinnhuber and Yu (1977). To tubes containing 2 g of test materials, 3 mL of thiobarbituric acid solution (1 g/100 mL) and 17 mL of trichloroacetic acid (2.5 g/100 mL) were added and mixed. Tubes containing the mixture were then heated to 100°C for 30 min. Subsequently, 5 mL aliquot was carefully added to each tube containing 5 mL chloroform, mixed, and centrifuged at 200 g for 5 min. Three mL of the solution from each tube was then transferred to another tube containing 3 mL of petroleum ether. After mixing, the mixture was centrifuged at 200 g for 10 min. Malondialdehyde as the reactive substance was quantified at 532 nm using a spectrophotometer (UV-1601; Shimadzu, Kyoto, Japan) and expressed as mg malondialdehyde kg−1.
Total bacterial counts were assessed using total plate count (TPC) agar medium and those of Enterobacteriaceae were assessed using violet red bile (VRB) agar medium as described by Chin et al. (2006).
TPA was performed for sausages using a Universal testing machine 3344 (Instron, Canton, USA). Sausages were cut to dimensions of 1.3 cm (height) and 1.25 cm (diameter) with a puncturing apparatus. Textural parameters such as hardness (gf) and springiness (cm) were analyzed along with machine derived data of gumminess (hardness×cohesiveness), chewiness (hardness×cohesiveness×springiness), and cohesiveness (ratio of active work done under the second compression curve to that done under the first compression curve). The analysis was undertaken using published procedures (Bourne, 1978).
In this study, three replications were performed and quantification of all parameters were made in duplicate. Data are presented as mean±standard derivations. SPSS 20.0 for Windows (SPSS, Chicago, IL, USA) was used for all data analyses. Statistical comparisons were carried out with two-way analysis of variance (treatment and storage time), and p-values <0.05 were considered significant.
Results and Discussion
Average particle sizes of CPs evaluated by laser diffraction instrument were 265 μm for coarse powder, 68 μm for micro powder, and 7 μm for ball-milled or superfine powder. Effects of CPs on pH and L* values are shown in Table 2. As main factors, treatments and storage time had significant effects (p<0.05). However, no interaction was found between the two factors (p>0.05). The pH values of samples with all treatments were lower than those of reference sausages (p<0.05). The pH values of sausages added with CP of higher particle size (T1 and T2) were lower than those of T3 (p<0.05). Concentration of various constituents including carbohydrates, proteins and polyphenols observed in celery concentrates could possibly influence pH and nitrite reactions (Djeri, 2010; Horsch et al., 2014). It is likely that the amount of phytocomponents in the powders with different particle sizes (T1, T2, and T3) could have affected the pH values.
Among color parameters, L* values was affected by treatment and storage (p<0.05). No interaction was found between these factors (p>0.05). Sausages added with CP (all particle sizes) had lower L* values compared to other sausages (p<0.05). The lowest L* value was found for sausages added with ball-milled CP (T3). This could be attributed to the increased surface area and in turn to well-dispersed powder. This could be attributed to the increased surface area of powder that can cause particles to be well dispersed in the sausage. Furthermore, it is possible that ball-milling could have enhanced the extraction of pigments from celery stalk. In T3, owing to its lower mean particle size of 7 μm, celery particles were not visible compared to the other two treatments. Decreased L* values with addition of plant materials have also been reported in other studies (Naveena et al., 2008). CIE redness (a*) and yellowness (b*) are shown in Table 3. The two factors, treatment (particle size of CP), storage days (time), and their interaction (treatment and storage days) had significant effects (p<0.05). Sausages added with CPs had lower redness values but higher yellowness values compared to reference sausages (Fig. 1). This might be due to pigments present in CPs, in agreement with results of Horsch et al. (2014). They suggested that the color differences between natural and conventional nitrite hams was due to plant pigments present in celery concentrates. However, among samples added with CPs at different particle sizes, redness values decreased with decreasing particle size (granularity). Increasing fineness of powder caused lower redness but higher yellowness (b*). Interestingly, the redness and yellowness remained stable throughout the storage time for sausages added with sodium nitrite while redness increased and yellowness decreased with storage time for sausages loaded with CP. A relatively large increase in the redness of sausages was noticed for the sample added with CP at the largest particle size (265 μm) after 3 days of storage. This indicated that powders with relatively larger particle sizes retained higher nitrite content, which was released gradually during storage.
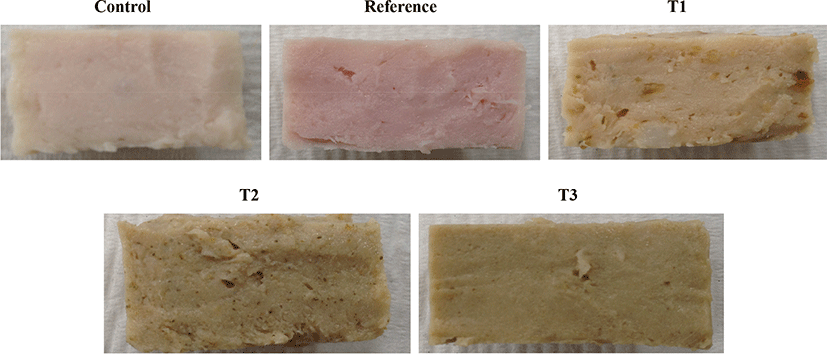
Results for the effect of CP on microbial growth in sausages are presented in Table 2. Similar to pH and L* values, interaction between treatment and storage time was not significant. Addition of CP decreased total bacterial count of sausage compared to control sausage, indicating that CP might have antimicrobial activity. Enterobacteriaceae counts for all treatments were also lower than those of control sausages. Increasing storage time resulted in increased microbial growth. Previous studies have shown that nitrite can retard the growth of microbes such as L. monocytogenes without completely stopping their growth even at concentrations used in conventional curing (Xi et al., 2012). Therefore, microbial counts increased with increasing storage time. Moreover, the effect of CP as an antimicrobial agent is dependent on the concentration of ingoing nitrite (Xi et al., 2012). Variability in antimicrobial effect of CP at different sizes could also be partially due to difference in concentration of nitrite present in CPs with various particle sizes.
Results for the ability of CP to retard lipid oxidation are summarized in Table 2. Although celery-loaded sausages had lower TBARS values than control sausages, their antioxidant activities were not comparable to those of reference sausages. Higher antioxidant activity observed in reference sausages is mainly due to sodium nitrite and BHT, which are commonly added to meat products. However, in this study, CP with the smallest particle size (T3) had higher tendency to decrease TBARS than CP with the other two particle sizes. Some studies have shown that CP is comparable to sodium nitrite for limiting lipid oxidation over time (Sindelar et al., 2007). Variations in nitrite and polyphenolic contents can often lead to different antioxidant and curing effects. It is important to note that antioxidant properties of CP can greatly affect its applicability in food. Nevertheless, CP with different particle sizes had lower TBARS values than control sausages, indicating its antioxidant capacity.
For residual nitrite concentrations, significant (p<0.05) interaction between treatment and storage time was found (Table 3). During the initial stage of storage, residual nitrite levels for reference sausages were the highest among all samples. However, with increasing storage time, residual nitrite level decreased as reported in other studies on nitrite added meat products (Xi et al., 2012). CP loaded sausages had progressively higher residual content than reference sausages. Residual nitrite content in celery added ham has been reported to be higher than conventional nitrite added ham (Horsch et al., 2014).
Sausages added with CP at particle size of 265 μm had the highest residual nitrite, followed by sausage added with CP at particle size of 68 μm and that at 7 μm. In addition, sausages added with CP at the largest particle size had higher residual nitrite levels in comparison with sausages added with CP at the other two particle sizes throughout the storage time. This is related to increased a* values of sausages added with these powders. Even with enhanced extraction of ball-milling, sausages added with superfine powders did not have high a* values. The relatively lower particle size might have exposed larger quantities of nitrite at the initial stage, thereby improving the curing efficiency at the earliest stage. On the other hand, relatively larger particles might have acted as reservoir for nitrates. Larger particles might have retained more nitrates within plant fibers and such nitrate might have been gradually released during storage. Similar variations have also been noticed in other studies (Sindelar et al., 2007). Furthermore, the process of curing through nitrite is a complex one. The addition of nitrite into meat product can result in the formation of an intermediate pigment, nitrosylmetmyoglobin (NO-MMb), which is unstable. It auto reduces with storage time due to the presence of endogenous or exogenous reductants in muscle tissue (Pegg and Shahidi, 2000). In the present study, residual nitrite level of reference sausage decreased with increasing storage time.
Effects of CPs on cured and total pigments are shown in Table 3. The concentration of cured pigment was higher for reference sausage than that for any other treatment. However, the concentration decreased with increasing storage time. At the initial stage of storage, the concentration of cured pigment was higher for lower particle size amongst CPs. With increasing storage time, the concentration of cured pigment increased. These results are contrary to other studies showing that naturally cured meat products have comparable cured pigments to conventionally cured products (Pietrasik et al., 2016). Such variation in results could be attributed to the lack of uniformity in sample composition (particles retaining varying amounts of nitrates). Similar variation has also been observed in previous studies (Sindelar et al., 2007).
The curing efficiency is the ratio between cured pigment and total pigment in percentage (AMSA, 2012). These results indicated that the efficiency was higher initially for reference sausage than any other sausage, although it decreased with storage time. The curing efficiency for CP with the lowest particle size (7 μm) was higher than that of other particle sizes until 3 days after storage. After 3 days of storage, curing efficiencies of samples added with CP at relatively larger particle sizes (T1 and T2) were higher than those added with CP at the lowest particle size (T3). At 6th day, the curing efficiency of sausage added with CP was higher than that of reference sausage. Such increase in curing efficiency for CP loaded sausages during storage might be due to gradual release of nitrite from celery particles. The presence of some endogenous bacteria might have also led to the gradual conversion of nitrate to nitrite.
Textural parameters of sausages prepared with CP of different particle sizes are shown in Table 4. Regardless of particle size, CP did not significantly affect texture parameters such as hardness, springiness, chewy, gumminess, or cohesiveness of sausages. Even with decreased water-holding capacity of ball-milled CPs (Ramachandraiah and Chin, 2016), textural properties of sausages were unaffected when compared to sausages added with coarse powders. Cell wall components of plant parts possess the ability to retain water during cooking. Previous studies have shown improved textural properties upon addition of some plant materials (Pietrasik et al., 2016). This is often attributed to cell wall polysaccharide such as pectin. In the current study, no such enhanced ability to retain moisture content was noticed. CP with larger particle size (T1) indicating higher particulates (fibers, carbohydrates, and minerals) did not retain moisture better than CP with lower particle size powders. This is different from a previous study showing that the springiness is improved and cohesiveness is lower for CP added ham (Pietrasik et al., 2016).
Conclusion
Sausages loaded with CP at the largest particle size had higher redness (a*) values but lower yellowness (b*) than those loaded with CP at lower particle sizes during storage. Sausages added with CP at the largest particle size also had higher residual nitrite content than those added with CP at smaller particle sizes. Textural properties of sausages were unaffected by particle size of CP. Thus, CPs with larger particle size would be preferable for improving physicochemical properties and antioxidant activities of meat products intended for longer storage. Although CPs had antimicrobial effect, they were not comparable to conventional nitrite. These results emphasize the need for further investigations on the efficiency of conversion from nitrate to nitrite for different particle sizes with the use of starter cultures. It could also be worthwhile to evaluate the effect of an additional natural antimicrobial to reach antimicrobial quality comparable to conventional sausages. Nevertheless, CP with different particle sizes could serve as an alternative for developing natural organic label friendly meat products.