Introduction
The consumption of chicken meat has been increased continuously over the past several decades thanks to the dramatic improvement in meat production through the intensive genetic selection for the growth rate and muscling, and the improvement in nutrition and management over the past 50 years (Aviagen, 2014). The improvements in the growth rate, breast muscle size, and yield in broilers, however, introduced serious abnormalities that influenced the quality of breast meat. Some of the abnormalities such as pale-soft-exudative (PSE) conditions and deep pectoral muscle (DPM) myopathy were known for decades, while other abnormalities such as spaghetti meat (SM), white striping (WS) and woody breast (WB) have not been reported until recently even though these conditions also have been known for some time (Maiorano, 2017; Petracci and Cavani, 2012). All the old and new abnormalities are associated with the dramatic improvement in growth rate and muscle size, which affected the structure, metabolism and repair mechanisms in poultry muscles (MacRae et al., 2006; Velleman, 2015; Velleman et al., 2014).
Although the incidence levels of the abnormalities vary widely depending upon researchers, Kuttappan et al. (2016) reported that the percent of broilers exhibiting WS/WB was as high as 90% and caused the U.S. poultry industry from $200 million to $1 billion economic losses per year. This review discusses the etiology, histological, physiological and chemical aspects of the abnormalities. The impacts of the anomalies on the functional, mechanical and sensory quality of the meat and their implications to the poultry industry are also discussed.
Pale-Soft-Exudative (PSE) Conditions
The incidence of PSE is closely related to the increased susceptibility of the birds to stress while other abnormal conditions are more related to the myopathies. A genetic mutation in the ryanodine receptor of sarcoplasmic reticulum that regulates calcium uptake and release is generally believed to be associated with the stress-susceptibility and the development of PSE conditions in pigs (Barbut et al., 2008). In poultry, however, it is not clear if the genetic mutation is the primary cause of PSE (Strasburg and Chiang, 2009). The PSE conditions in chickens are mainly attributed to a rapid post-mortem decline in pH due to pre-slaughter stresses and environmental factors (heat stress) (Petracci et al., 2015). Sandercock et al. (2009) reported that fast-growing birds exhibited a reduced thermoregulatory capacity, and thus those birds are more susceptible to heat stress during the pre-slaughter period. Heat stress causes various problems such as muscle damage, acid-base disturbances, and reduced meat quality. The broiler breast muscle is mainly composed of fast-twitching fibers that are mainly associated with the anaerobic glycolysis (Yost et al., 2002). Pre-slaughter stress conditions accelerate anaerobic glycolysis during the conversion of muscle to meat and lower muscle pH while the carcass temperature is still high. The low pH and high-temperature combination leads to protein denaturation and produces PSE conditions (Berri et al., 2005; Wilhelm et al., 2010). The PSE meat has an unattractive pale color, soft texture, and low water-holding capacity (WHC) and protein extractability (Barbut et al., 2008). Therefore, the processing ability of PSE meat is low, and the texture of the processed meat products is brittle and dry. The incidence level of PSE conditions is about 5% to 40%, and thus PSE conditions cause significant economic losses to the poultry industry (Owens et al., 2009).
Deep Pectoralis Muscle (DPM) Myopathy
DPM, also known as “green muscle disease,” was first described in turkeys and chicken breeders as “degenerative myopathy” (Dickinson et al., 1968). However, it has become common in all meat-type fast-growing birds (Bilgili and Hess, 2002; Grunder et al., 1984). DPM is defined as ischemic necrosis that develops in Pectoralis minor, and the genetics, physio-anatomical background and the management of birds are related to the incidence of DPM. The Pectoral major and the Pectoral minor are the two muscles involved in wing movements. When the birds are nervous, startled, flight and struggle because of feed or water outages, human activities, and excessive noises in and around chicken houses, they prone to have sudden movements of wings (Bilgili et al., 2000). In the turkeys and broilers, Pectoralis minor muscle that raises wings during the wing flapping expands its volume by about 20%–25% for the increased blood flow into the muscle. However, Pectoralis minor muscle is sandwiched between the sternum and inelastic fascia, and thus cannot fully expand during the activities. This generates pressure to the Pectoralis minor muscle and occludes the blood vessels, and causes oxygen deficit and develops ischemia (Jordan and Pattison, 1998; Sille, 1985). In lighter birds, this pressure quickly returns to the normal state. In heavy birds, however, the pressure can be maintained long enough to lead to irreversible necrotic changes in the Pectoralis minor muscle (Bilgili and Hess, 2002). In the early stage, DPM muscles show acute inflammation, edema, and hemorrhages. In the later stage, however, the conditions change to degenerative necrosis with muscle fiber atrophy, follicular, segmental and granular decomposition, macrophage infiltrations, connective tissue proliferation, and eosinophile and phagocyte infiltrations (Fig. 1). In the most advanced lesions, normal muscle fibers are replaced by fibrous and adipose tissue and the color changes from pink to green (Bianchi et al., 2006).
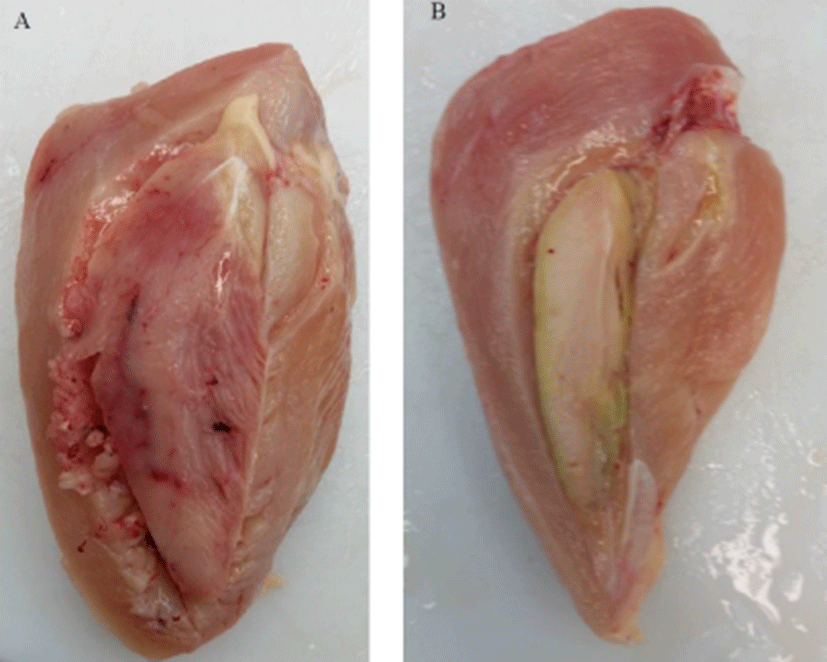
The part with deep pectoral myopathy in breast muscle is trimmed off, which downgrades the breast and causes an economic loss for the industry (Kijowski et al., 2014). Under commercial rearing conditions, broilers and turkeys are relatively inactive, and the Pectoralis minor muscle is not used much. Therefore, the elasticity of the muscle compartment is reduced, and it is difficult for those birds to accommodate the swollen muscle after wing flapping. The reported incidence level of DPM varies widely depending upon the age of birds and genotype: Bianchi et al. (2006) reported that the incidence of carcasses affected by DPM was 0.84%, but the range of total DPM varied from 0% to 16.7% range. Considerable variations were also observed for early (range: 0% to 12.0%) and old (range: 0% to 5.6%) developing stages of DPM. Ross 308 exhibited a higher incidence of DPM than Cobb 500 (1.27% vs 0.35%). Lien et al. (2011) reported that the incidence of spontaneous DPM cases ranged from 3% to 17% and was greater in broiler chickens with a higher growth dynamic than those with normal growth rate. Badger (2011) identified 4.4% DPM cases in chickens at slaughter and cutting up the carcass. Kijowski et al. (2014) reported that the incidence of DPM cases in commercial slaughter chickens was between 0.02% and 1.9% in Poland.
White Striping (WS)
WS is characterized by white striations parallel to muscle fibers due to fiber degenerations with fat infiltration (lipidosis) and connective tissues (fibrosis) (Fig. 2). The intensity and the thickness of the white stripes can vary from bird to bird. Kuttappan et al. (2012a) developed a classification system for WS based on visual appearance: normal, moderate and severe. However, more extensive groupings can also be used when necessary. The major histopathological changes associated with WS include necrosis of fibers, degenerating and regenerating fibers of variable size, loss of cross striations, mineralization of nuclei, hyalinization, replacement of muscle fibers with fibrous connective tissues (fibrosis), accumulation of adipose tissues (lipidosis), and lymphocytes and macrophages infiltration (Ferreira et al., 2014; Russo et al., 2015; Sihvo et al., 2014). Kuttappan et al. (2013b) reported that the WS was not associated with any systemic infections, inflammatory, or stress conditions, but had increased levels of serum creatine kinase (CK), alanine aminotransferase (ALT), aspartate aminotransferase (AST), and lactate dehydrogenase (LDH). When the damages are acute or continuous, and the attempts to repair the damages fail, satellite cells of the muscle tissues become fibroblasts or adipocytes and lead to fibrosis and lipidosis.
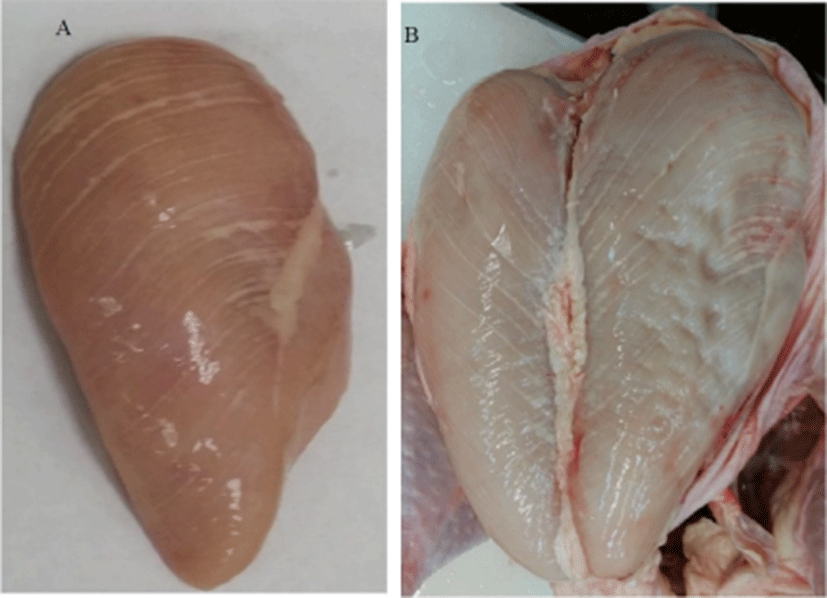
Kuttappan et al. (2009) and Kuttappan et al. (2013a) found that more than 50% (50.7%–55.8%) of broilers had WS conditions at 59–63 days of age. Trocino et al. (2015) found 74.5% of the fillets examined were WS at the gross examination, but 97% of the breast muscles examined showed histologically damaged muscle fibers. Many studies reported that the incidence of severe WS increased dramatically from 1.4%–8.7% (average 5%) in 2012 (Kuttappan et al., 2012a; Petracci et al., 2013) to 25.7%–32.3% (average 29%) in 2015 (Russo et al., 2015; Tijare et al., 2016), and to 75.5% in 2017 (Gratta et al., 2017), indicating very rapid increase of the incidence levels. Also, the occurrence of severe WS was higher in Ross 308 chickens than in Cobb 500 chickens (25.9% vs 7.41%), and up to 40% of medium and heavy broiler chickens raised under commercial conditions had WS conditions (Lorenzi et al., 2014).
Feeding a high-energy diet produced birds with higher body weight and accomplished lower feed conversion ratios but increased the incidence of WS breast fillets. Higher levels of lipids and lower amounts of protein in diets also increased the occurrence of WS in broilers (Kuttappan et al., 2009). Bauermeister et al. (2009) reported a higher incidence in broilers processed at an older age (8 wks old) than a younger age (6 wks old) because of the higher body weight at older ages. More than half of the broilers are processed at older ages currently (>8 wks), and the meats are deboned and used for portioning and further processing (Owens, 2018). So, WS in broiler breast fillets could affect the poultry meat market significantly and result in higher economic losses.
The occurrence of WS in broilers was reduced by controlling the growth trajectory through feed reduction during the rearing period (Meloche et al., 2018b). Restricting feed from 13 to 21 d of age was effective in controlling muscle fiber degeneration, but too early feed restriction (first two-week post-hatching) had adverse effects on the growth of broilers (Kuttappan et al., 2012a). During the re-alimentation period, however, previously restricted birds showed a compensatory growth and the effects of feed restriction disappeared (Trocino et al., 2015). The broiler line with high ultimate pH had a higher incidence of moderate and severe WS than the low ultimate pH lines regardless of the sex (Alnahhas et al., 2016; Muldahl et al., 2015; Petracci et al., 2013).
WS meat exhibited a higher percentage of moisture, intramuscular fat and collagen, and lower contents of myofibrillar and sarcoplasmic proteins and ash (Kuttappan et al., 2013a; Petracci et al., 2013). Because of the decreased total protein content but increased collagen and fat contents in WS breast muscles, their WHC, protein solubility and marinade uptake are lower than the normal muscles (Sihvo et al., 2014). The digestibility and the quality of amino acids in collagen are inferior to myofibrillar proteins. Therefore, the nutritional quality of WS meat is lower than that of the normal meat (Mudalal et al., 2014). The solubility of myofibrillar proteins is highly correlated with the WHC (Warner et al., 1997) and the functionality of the meat (Bowker and Zhuang, 2013). Bowker and Zhuang (2016) reported that WS fillets had higher ultimate pH and Allo-Kramer (AK)-shear force but had lower a*- and b*- color values than the normal fillets (p<0.001). Lightness (L*) was generally higher in the WS than the normal breast fillets but was not always consistent. Some researchers reported that WS had a significant impact on the visual appearance of breast fillets and lowered the consumer acceptance of the meat (Kuttappan et al., 2012c; Mudalal et al., 2014). However, Soglia et al. (2018a) indicated that WS conditions did not have significant effects on meat quality.
There are a few other known causes of myodegeneration such as vitamin E and selenium deficiency, exertional or exercise-induced myopathy, toxins, and tissue hypoxia in poultry. Among those myopathies, nutritional muscular dystrophy (NMD) is characterized with white-colored bands on the breast and leg muscles in the direction of the muscle fibers, which is very similar to that of the WS muscles. The NMD is associated with the degeneration of muscle fascicules (Netke et al., 1969) and its microscopic characteristics are also similar to those of the WS (Kuttappan et al., 2009). The NMD is caused mainly by the vitamin E and Se deficiency and can be prevented by the dietary supplementation of vitamin E and Se (Walsh et al., 1993). However, dietary vitamin E level had little effect on the incidence of WS (Guetchom et al., 2012; Kuttappan et al., 2012b).
Spaghetti Meat (SM)
WS defect is also associated with another muscle abnormality termed SM (Sirri et al., 2016). While WS fillets exhibited necrosis and lysis of fibers, fibrosis, lipidosis, inflammation, loss of cross striation, and vacuolar and hyaline degeneration, SM is characterized by poor muscle cohesiveness due to the immature intramuscular connective tissues (Bowker and Zhuang, 2016; Radaelli et al., 2017; Sihvo et al., 2017). Collagen maintains the structural integrity of skeletal muscle (McCormick, 2009; Purslow, 2005): intramuscular connective tissues are composed of three layers called endomysium, perimysium, and epimysium, which surrounds individual muscle fibers, the bundles of muscle fibers, and the whole muscle, respectively. In SM, the density of connective tissues in endomysium and perimysium progressively decreases, and thus the muscle fiber bundles become easily disintegrated or mushy (look like spaghetti) (Puolanne and Voutila, 2009).
The histopathological features of the SM abnormality share similar morphological changes to other myopathies (e.g., WS, WB): Swatland (1990) observed abnormal conditions similar to the SM in turkey breast muscles and attributed that to the outgrowth of muscle fibers to their connective tissues. Ahn et al. (2010) observed that the perimysial septa of breast muscles in fast-growing birds were thinner than the slow-growing counterparts. Muscles with both WS/SM conditions showed the most severe histological lesions with increased adipose tissue infiltration in the endo- and perimysial spaces and high intra- and extra-myofibrillar water content (Radaelli et al., 2017). Using NMR, Baldi et al. (2018) found a movement of water from intra-myofibrillar to extra-myofibrillar area in SM. A higher proportion of extra myofibrillar water in the superficial section of SM led to a reduction of WHC of the meat. Both WS and SM abnormalities influenced meat quality, but SM had more pronounced adverse effects on meat quality than the WS (Baldi et al., 2018).
Wooden (or Woody) Breast (WB)
WB is macroscopically characterized by bulged, hard and rigid muscles with a surface hemorrhage (Fig. 3) and the presence of a light-yellow viscous exudate on the muscle surface (Mazzoni et al., 2015; Mudalal et al., 2015). Wooden breast muscle has a larger cross-sectional area (Dalle Zotte et al., 2014), and higher intramuscular collagen and ultimate postmortem pH (Chatterjee et al., 2016; Clark and Velleman, 2016; Petracci et al., 2015; Soglia et al., 2016) than the normal muscle. Histopathologically, WB is characterized with increased degenerative and atrophic fibers, variability in fiber size, floccular/vacuolar degeneration and lysis of fibers, hyalinization, lipidosis, extensive fibrillar collagen deposition (fibrosis), and macrophage infiltration (Sihvo et al., 2014; Soglia et al., 2016; Velleman and Clark, 2015). The WB lesions begin focally and spread as a diffuse form as the age of the bird increased. Some birds showed markedly hardened breast muscle with no apparent fibrosis histologically, indicating that fibrosis is not the sole factor behind the hardness of the WB meat and the lesion is not restricted to the Pectoralis major muscles only.
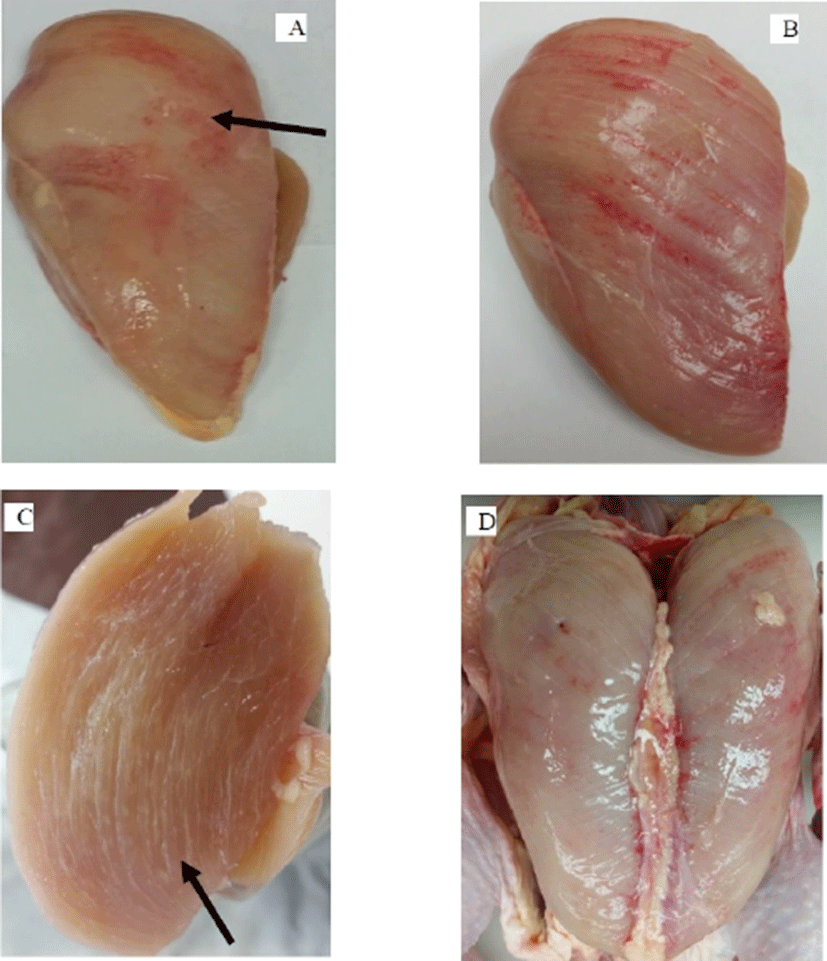
The birds with high growth rate, feed efficiency, and breast muscle yield are more likely to develop myopathies including WB (Griffin et al., 2018) because the muscle tissues outgrow the supporting systems such as connective tissues and capillaries and change the structure and metabolism of the muscle (Petracci and Cavani, 2012; Sandercock et al., 2009). Muscle fiber formation is virtually complete at hatch. Hyperplasia occurs during the embryonic development and hypertrophy after hatch. Hypertrophy can increase the muscle fiber size (cross-sectional area) 5–6 folds (Dransfield and Sosnicki, 1999). The post-hatch muscle growth, development, and maintenance are accomplished through the activities of satellite cells. Satellite cells are multipotential stem cells that are heterogeneous depending upon the fiber type of the muscle they are derived from and the expression of cell surface markers. They are located between the basement membrane and plasmalemma of muscle fibers (Mauro, 1961). The satellite cells proliferate, differentiate and fuse with adjacent fibers but remain quiescent after hatch (Moscatello et al., 1998). The muscle fiber enlargement is accomplished through the fusion of satellite cells into the existing muscle fibers that adds nuclei to the muscle fibers and increases protein synthesis (Allen et al., 1979; Velleman, 2015).
The extreme hypertrophy, however, results in reduced endomysial and perimysial connective tissue spacing (Velleman et al., 2014). Therefore, birds selected for hypertrophy have limited space for capillaries in the perimysial connective tissues area, which results in the decreased size and density of the capillary network (Sosnicki and Wilson, 1991). Both large muscle fibers and inadequate vascularization induce metabolic stress because the greater diffusion distances for oxygen, metabolites and waste products make it difficult to supply oxygen as well as remove the metabolic wastes and initiate muscle damages (Alnahhas et al., 2015; Alnahhas et al., 2016; MacRae et al., 2006). The low oxygen and nutrients from blood during the ischemic period lead to inflammation and oxidative stress rather than restoring the tissues to normal function. The re-supply of blood flow and oxygen to the ischemic cells lead to increased damage to cellular proteins and plasma membranes due to the production of superoxide by the xanthine-xanthine oxidase system and the deficiency of scavenger enzymes to remove the superoxide and other reactive oxygen species (ROS) (Halliwell and Gutteridge, 1990). An excess of ROS within the muscle tissue is involved in initiating the inflammatory mechanisms associated with the WS and WB muscle abnormalities (Mutryn et al., 2015).
The main inflammatory cells found around the degenerative fiber are macrophages (Sihvo et al., 2013). The activation of macrophages in stress conditions creates a source of additional free radicals. Macrophages use superoxide to destroy pathogens as their first line of defense. However, superoxide (O . –) is toxic to the cells and needs to be converted to a less toxic form quickly by superoxide dismutase. If not combated by the animals’ natural line of defense, however, the free radicals may lead to more cellular destruction due to lipid peroxidation of the biological membranes and compromise their structure and functions (Zhao et al., 1998). Trace minerals such as Se, Zn, Mn, and Cu are important for the activity of antioxidant enzymes, and play important roles in growth, immunity and gut health of animals (Cemin et al., 2018; York et al., 2016). Dietary supplementation of those minerals in combination with antioxidants enhanced the bird's natural defense system in the tissues, which are essential to combat free radical production (Kidd, 2004; Midorikawa et al., 2001).
Zambonelli et al. (2016) reported that WB muscle showed different genetic expression for glycolysis, oxidation, calcium signaling pathways, and proteoglycan and polysaccharide synthesis from normal muscles. The RNA-seq analysis and the microscopic and biochemical studies indicated that the localized hypoxia, increased muscle degradation, reduced glucose utilization, increased intracellular calcium and muscle fiber-type switching are the critical features of myopathic muscles (Abasht et al., 2016; Mutryn et al., 2015; Petracci et al., 2015). After the initial degeneration, the damages to the sarcoplasmic reticulum surrounding muscle fibers increase calcium influx and activate calcium-dependent protease (calpain) and initiate necrosis. WB exhibited a significantly higher amount of free calcium. The increase of sodium and calcium content changes the intracellular ion homeostasis, and the increase of glycolytic activity lowers the amount of glycogen reserve in the muscle, which leads to an increase of ultimate pH in abnormal muscles. Fiber-type switching, from type IIb fast-twitching fibers towards slow-twitching type I fibers, decreases the enzymes related to the glycolytic pathway and glycolysis (Soglia et al., 2016).
The instrumental texture of the cooked severe WB was harder than the normal breast fillets, and sensory analyses of the cooked WB showed more springiness and cohesiveness than the normal fillets (Chatterjee et al., 2016). However, the sensory texture attributes of WB were not uniform throughout the entire Pectoralis major muscle (Aguirre et al., 2018; Brambila et al., 2017). Tasoniero et al. (2017) reported that the hardness of WB is related to the extra water trapped in the myofibrillar matrix (myowater). Velleman and Clark (2015) suggested that the hardened area of wooden breast muscle is related to the very high levels of decorin expression and excessive collagen crosslinking. Decorin is a small leucine-rich proteoglycan that is involved in collagen crosslinking and other functions including cellular growth, collagen fibril structure, and extracellular matrix organization (Danielson et al., 1997). However, Radaelli et al. (2017) reported that the replacement of the degenerated muscle fibers with connective tissue (fibrosis) was not related to the muscle hardness. The activity of μ/m-calpain decreased significantly in WB during storage but the differences in the calpain activities during the postmortem period was not the primary factor for the increased hardness in WB (Soglia et al., 2018b). Marination decreased the toughness in WB fillets but did not eliminate tenderness differences between the normal and the WB fillets.
Compared with the normal breasts, WB meats showed higher drip and cooking losses and shear force for both raw and cooked meat (Aguirre et al., 2018; Chatterjee et al., 2016; Mudalal et al., 2014). Although, the objectionable texture characteristics of WB fillets can be overcome by grinding the meat, the compositional changes still can result in impaired functionality in further-processed products (Bowker and Zhuang, 2016; Mazzoni et al., 2015; Mudalal et al., 2015; Tijare et al., 2016). WB fillets had higher color L*-, a*-, and b*-values and pH than the normal breast fillets (Cai et al., 2018). Wold et al. (2017) developed a Near-infrared (NIR) spectroscopy for rapid and non-destructive detection and grading of WB in chicken breast fillets and found that the NIR was a practical and useful tool for detecting and grading WB syndrome in the processing line. They also used low-field NMR to measure WHC and found that WB muscles had a significantly higher share of loosely bound water due to muscle fiber degeneration.
WB conditions have been found in several countries around the world, including the United States, Finland, Italy, and Brazil. Although, the industry-wide incidence rates for the WB muscle are challenging to assess, approximately 5%–10% of commercially produced breast fillets display severe WB conditions. Owens (2014) reported that 30% to 50% of broilers at 56 days of age (BW>4.2 kg) showed severe WB conditions. Gratta et al. (2017) reported that 5.1% of commercial broilers were WB, while Cruz et al. (2017) estimated up to 85.9% at 35 d of age and up to 89.2% at 42 d of age. Tijare et al. (2016) observed up to 96.1% of WB incidence in broilers reared using commercial diets. WS often coexisted with WB. The first WS and WB cases were seen at 10–18 days of age, and the prevalence levels increased as the age of the birds increased (Sihvo et al., 2017; Wold et al., 2017). Myopathies are more directly related to the slope of the growth trajectory of birds and the reduced nutrient allocation at 90% density throughout the grow out period reduced the severity of WB. The quantitative control of nutrient intake, however, had a negative impact to the performance of the birds and processing characteristics (Meloche et al., 2018a; Meloche et al., 2018b; Trocino et al., 2015). Therefore, the concurrent manipulation of dietary amino acid and energy density is not a viable and practical solution for breast myopathies (Meloche et al., 2018a).
Woody Breast with White Striping
Mudalal et al. (2015) indicated that the measurements of height or compression force in the caudal part of fillets could be used as a tool to discriminate between WS and wooden breast abnormalities. Both WS and WB are observed in varying degrees of severity, and sometimes they appear together in one muscle. WS and WB alone or together negatively impacted several aspects of meat quality, including WHC and proximate composition (low protein coupled with high moisture, collagen, and fat contents) (Soglia et al., 2016; Tijare et al., 2016). The occurrence of both WS and WB is closely associated with up-regulation of protein metabolism and the regenerative process to repair the degenerative changes, and the down-regulation of carbohydrate metabolism (Kuttappan et al., 2017).
Both WS- and WB-lesioned areas showed myodegeneration with a variable amount of interstitial connective tissue accumulation (Sihvo et al., 2014), which changed the nutritional and technological properties of meat (Mazzoni et al., 2015; Mudalal et al., 2015), and negatively affected the consumer preference (Kuttappan et al., 2012c). Over 50% of consumers indicated that they would not buy WS and WB fillets (Kuttappan et al., 2012c).
The WB/WS myopathies have both macroscopic and microscopic similarities to other myopathic conditions like hereditary muscular dystrophy, nutritional myopathy, toxic myopathies, etc., but their etiologies are different (Kuttappan et al., 2016). Mutryn et al. (2015) identified a set of differentially expressed genes involved in myofiber reactions to oxidative stress in WS/WB muscles. Dridi and Kidd (2016) reported that WB meat is a result of a metabolic disorder that is associated with hypoxia and oxidative stress. Immunoglobulin superfamily was also overexpressed in abnormal muscles, indicating the presence of the tissue inflammation. The expression of interleukin-1-beta (IL1B-β), a cytokine protein, is vital for the angiogenic processes (Voronov et al., 2003). The low IL1B-β expression level is related to the lack of pain symptoms in chickens affected by WB/WS abnormalities because IL1B-β regulates the pain sensation during inflammation.
Both WS and WB abnormalities impair the appearance and the functional quality of breast meats, but WB had a more detrimental effect than WS, and the adverse effect was more severe when both WS and WB were present together (Mudalal et al., 2015; Tasoniero et al., 2016). There are no published studies on microbial shelf-life of WS and WB fillets, although both have higher ultimate pH than the normal meat (Mudalal et al., 2015; Petracci et al., 2013). The notable discolorations on the skin-side surface of severe WB fillets after cooking and the white striations on the ventral surface of raw WS fillets negatively impacted the purchasing decisions of consumers.
Although the selection for increased growth rate and breast yield played a role in the occurrence of the abnormalities in poultry breast muscle, the genetic basis for the incidence of these myopathies is still debatable: Lien et al. (2011) reported low heritabilities for DPM (0.02–0.1) and relatively low heritability for WS (0.19–0.34). Bailey et al. (2015) also reported that the development of myopathies has poor heritability and genetic correlations, and environmental factors such as nutrition and management contributed more than 90% and 65% for the incidence of WB and WS, respectively. However, Alnahhas et al. (2016) reported that WS had a high heritability (0.65) and also had medium to high correlations with body weight, breast meat yield, and intramuscular fat (0.33, 0.68, and 0.64, respectively). Significant genetic associations were also observed between WS and the ultimate pH of breast and thigh meat (0.21 and 0.31, respectively), and breast cook loss (0.30). Chabault et al. (2012) found that WS was highly correlated with the intramuscular fat content of the Pectoralis major muscle (heritability 0.83) and suggested to use it as an indirect criterion for the selection against WS.
Conclusions
The incidences of the newly emerging myopathies (SM, WS, and WB) as well as the previously known abnormalities (PSE and DPM) are much higher in the heavy birds selected for the fast growth rate and high breast size and yield than the slow-growing birds. However, the heritability and correlations of the genetic parameters for growth rate, breast yield and breast size to the abnormalities are lower than those of the environmental factors that include nutritional density of the diet, management, and age of the birds. Although, all the abnormalities (myopathies) are different macroscopically they share many similarities in histopathological and biochemical characteristics. Also, all the abnormalities share the common initiating mechanisms – muscle fiber growth outpaces the development of connective tissues and vascularization. Many researchers have studied the initiating mechanisms and the histopathological and chemical changes in the abnormal meat. However, early detection and prevention methods for the abnormalities are not known yet. Developing non-invasive biomarkers based on mass spectroscopy for diagnostic purposes in live birds and a rapid, automatic detection and grading system in slaughtering and processing lines can be of help, but they cannot be the fundamental method to solve the issues related to the muscle abnormalities.
The abnormalities in breast meat raise concerns over the quality as well as safety and lower the consumer acceptance of the meat. In severe cases, meats with abnormalities are downgraded, and the defected meats are often used in processed products (e.g., sausages or nuggets) where the chemical composition can be modified during formulation (Qin, 2013). However, it only alleviates the quality problems (texture, color), not completely solve the problems. In 2017, USDA (2017) announced that the breast meat with the signs of inflammation (WS and WB abnormalities) should be trimmed off. The meats with inflammation are considered unwholesome and unfit for human consumption. Therefore, the economic loss due to the myopathies can be greater than the impact caused by the quality loss alone. So, more fundamental works to develop birds with no myopathic conditions or studies to prevent the initiating process through genetic selections or management for the high fibroblastic expression (collagen development) and angiogenesis may be necessary.