Introduction
From multicellular microorganisms to bacteria, many organisms produce antimicrobial peptides. Antimicrobial-peptide-producing bacteria are thought to gain a competitive advantage in specific ecological niches. The antimicrobial peptides produced by bacteria are called bacteriocins. In general, bacteriocins have quite a narrow antimicrobial spectrum. Bacteriocins generally exhibit antimicrobial efficacy toward a single species, particularly against species that are phylogenetically related to the bacteriocin-producing bacteria. These peptides are very potent and are effective at pico- or nanomolar concentrations (Hassan et al., 2012).
Bacteriocins form channels in the target cell membrane, causing low-molecular-weight ions to leak from the cell, leading to collapse of the proton motive force (Dicks et al., 2011; Hassan et al., 2012). Bacteriocins are highly heterogeneous peptides in terms of size, structure and activity. According to a recent classification, bacteriocins produced by Gram-positive bacteria have been divided into two large groups (Chen and Hoover, 2003; Cotter and Ross, 2005);
Class I, lantibiotics, are small peptides with post-translational modifications. Class Ia peptides are small peptides containing 19–38 amino acids. Nisin, which is produced by Lactococcus lactis, is the best known lantibiotic and was the first bacteriocin allowed to be used as a natural preservative in foods. Class Ib peptides are globular peptides that affect the essential enzymes of the target cell.
Class II, non-lanthionines, are small peptides containing 25–60 amino acids; these peptides are non-modified and heat-resistant. LAB are mostly producers of class II bacteriocins. Class II peptides are divided into 4 subgroups. Class IIa bacteriocins are known for their anti-listerial activity. These peptides are also called pediocin-like bacteriocins because pediocin was the first peptide in this group to be characterized. Class IIb bacteriocins consist of two peptides, and both peptides are required for activity. Class IIc bacteriocins are cyclic peptides that are covalently bonded at the N and C termini. Class IId bacteriocins are linear, single-peptide and non-pediocin-like bacteriocins.
When the characteristics of bacteriocins are taken into consideration, these peptides are thought to be promising therapeutic agents for the control of microbial pathogens, including multidrug-resistant pathogens (Dicks et al., 2011). Some in vivo studies have determined that various bacteriocins inhibit antibiotic-resistant bacteria. For example, mersacidin is produced by the Bacillus sp. HIL-Y85/54728 strain to inhibit methicillin-resistant Staphylococcus aureus strains in mice (Kruszewska et al., 2004). Lacticin 3147, produced by L. lactis subsp. lactis, has been reported to inhibit S. aureus, methicillin-resistant S. aureus and vancomycin-resistant E. faecalis (VRE) (Galvin et al., 1999). These studies have shown that bacteriocin-based therapeutic approaches contribute to the battle against these pathogens.
Probiotic bacteria (lactobacilli, bifidobacteria and enterococci) are natural producers of bacteriocins. Rea et al. (2010) have shown that bacteriocin-producing probiotics play an important role in the fight against infectious bacteria in the human gastrointestinal tract. Potential medical applications of bacteriocins have been well documented against various systemic urogenital, gastrointestinal, respiratory and skin infections, including infections caused by multidrug-resistant bacteria (Dicks et al., 2011).
Because LAB are accepted as “generally recognized as safe” (GRAS), the bacteriocins produced by the LAB are also accepted as being safe (Abbasiliasi et al., 2017; Macaluso et al., 2016; Yang et al., 2014). Patented applications of nisin and pediocin, either alone or in combination with other hurdle technologies, for the inhibition of various pathogenic or saprophytic bacteria in food have been reported by Cleveland et al. (2001).
Bacteria of the genus Enterococcus belong to the LAB group (von Right and Axelson, 2011). It is known that Enterococcus spp. are components of the microbiota of many fermented foods such as cheeses, olives and other plant products (Giraffa, 2003). High salt and pH tolerance make these bacteria particularly interesting in terms of their use as starters or co-cultures in food fermentations. On the other hand, enterococci are also associated with hospital-acquired infections. Such pathogens have multiple antibiotic-resistance and virulence factors (Franz et al., 2011; von Right and Axelson, 2011).
In the present study, the antimicrobial activity of the bacteriocin produced by E. faecalis KT11 against some food-borne and clinical pathogens, including vancomycin- and/or methicillin-resistant bacteria, was examined. Additionally results of partial purification and characterization studies of bacteriocin reported. To the best of our knowledge, this is the first study about the characterization and partial purification of bacteriocin produced by a lactic acid bacterium (E. faecalis KT11) isolated from Kargı Tulum cheese, an artisanal cheese produced by traditional methods with natural fermentation.
Materials and Methods
The E. faecalis strain KT11 (NCBI accession number: MH746081) used in this study was isolated (from Kargı Tulum cheese) as a part of our previous project in the Food Microbiology Laboratory of Eskişehir Osmangazi University. API 50CH and API Strep20 test kits (bioMérieux, France) and real-time PCR identification methods were used for species-level identification. E. faecalis KT11 was grown in de Man-Rogosa-Sharpe broth (MRS; Merck, Germany) at 37℃ for 24 h and maintained as a frozen stock at –20℃ in MRS broth containing 20% (v/v) glycerol. E. faecalis KT11 stock cultures revived in MRS broth and incubated aerobically for 24 h at 37℃ three times before use.
E. faecalis KT11 was inoculated (1% v/v) into MRS broth and incubated aerobically for 24 h at 37℃. Then, the culture was centrifuged at 5,000 rpm for 10 min at 4℃ (Sigma-3-16K, UK), and the supernatant was collected. To avoid the inhibitory effects of organic acids and hydrogen peroxide (H2O2), the pH of the CFS was adjusted to 6.5 with 5 M NaOH, and catalase (1 mg/mL) was added. The CFS was then filtered through a 0.22-µm pore size filter (Millipore, Merck). Catalase-free CFSs were used as the control series.
To determine the antimicrobial potential of the CFS, an agar well diffusion assay (AWDA) was performed. All indicator test strains (30 strains) used in this assay and the collection numbers of these strains are shown Table 1. Indicator test strains were first cultivated in appropriate broth media (MRS broth for LAB and nutrient broth for the other strains; Merck). Final cell density of each indicator strain was adjusted to 106 CFU/mL using McFarland standard no. 1 (Merck). Then, 100 µL of these cultures was inoculated into molten soft MRS/nutrient agar tubes (MRS broth/nutrient broth+agar (0.7% w/v)). After homogenizing, the inoculated soft agar was rapidly poured onto pre-prepared plates containing MRS/nutrient agar (Merck). After solidification, the soft agar plates were maintained at 4℃ for 1 h, and then, wells with 8-mm diameters were made with a sterile cork borer. Then, CFS was added into each well (100 µL/well), and the plates were incubated for 24 h at 28℃ for P. aeruginosa, B. cereus and M. luteus and 37℃ for the remaining indicator strains. Then diameter of the inhibition zone around the well was measured (including well diameter).
In this study, all antimicrobial activity measurements were made in duplicate and repeated at least twice.
The effects of hydrolytic enzymes, heat, pH, surfactants, organic solvents, lyophilization and storage temperature on the stability of bacteriocin KT11 was determined. S. aureus ATCC 25923 was used as indicator test strain to determine the effects of different treatment conditions on the activity of bacteriocin from E. faecalis KT11.
Sensitivity of bacteriocin KT11 to hydrolytic enzymes was determined by treatment with proteinase K, α-chymotrypsin, protease, pepsin, trypsin, catalase and α-amylase. All enzymes were supplied by Sigma-Aldrich, Germany; the enzymes were diluted (final concentration of 1 mg/mL) and then filter sterilized. Each enzyme solution was added to the bacteriocin KT11 samples and incubated (37℃ for 2 h). Then, the enzymes in the bacteriocin KT11 samples were heat inactivated (3 min at 100℃). The pH of the samples was adjusted to 6.5. Residual antimicrobial activity was monitored by conducting an AWDA as described above. Bacteriocin KT11+buffer and buffer alone served as controls.
Heat resistance of bacteriocin KT11 was determined by heating the bacteriocin KT11 samples to 60℃, 80℃, or 100℃ (30 or 60 min) or 121℃ (15 or 30 min). Antimicrobial activity was determined by conducting an AWDA. Untreated bacteriocin KT11 was used as a control. Percentage of activity was calculated for each replicate with this formula; % activity=inhibition zone of the treated sample/inhibition zone of the control sample×100.
The pH of the bacteriocin KT11 samples was adjusted to 2, 3, 4, 5, 6, 7, 8, 9, 10 or 11, and the samples were maintained at 4℃ for 0, 6, 12, 18 or 24 h. At specified timepoints, pH values of samples were re-adjusted to 6.5 and their residual antimicrobial activity were determined.
The effect of various organic solvents (all from Sigma-Aldrich, Germany) on bacteriocin KT11 was determined by adding chloroform (10%), propanol (10%), methanol (10%), ethyl alcohol (10%), acetone (10%), hexane (25%) or ethyl ether (25%) to the samples. Bacteriocin KT11 were incubated at 25℃ for 1 h and then evaporated in a vacuum concentrator (Hernández et al., 2005). Untreated bacteriocin KT11 and the organic solvents in MRS broth at the concentrations mentioned above were used as controls.
The effect of surfactants was determined by adding (1% v/v) ethylenediaminetetraacetic acid (EDTA), sodium dodecyl sulfate (SDS), Triton X-100, Tween 80 or urea (all from Sigma-Aldrich, Germany) to the bacteriocin KT11 samples (Todorov and Dicks, 2005). Untreated bacteriocin KT11 and the surfactants in MRS broth at the concentration mentioned above were used as controls.
Five milliliters of filter-sterilized bacteriocin KT11 samples were lyophilized (Christ, Alpha 1-4, UK; under 0.0010 mbar at –80℃ for 48 h) and stored at 4℃, –20℃, and –80℃ for 3 months. At timepoints of 1, 15, 30, 60, and 90 d, the samples were resuspended in the same volume of sterile deionized water, and the residual activity of each sample was tested by an AWDA (Cherif et al., 2003).
Five milliliters of an overnight E. faecalis KT11 culture was inoculated into 500 mL of MRS broth and incubated at 37℃ for 24 h. At 2-h intervals, 1 mL samples were taken, and the cell density of each sample was determined (OD600 nm). At the same sampling time, 10 mL of sample was used to prepare a CFS. Then serial two-fold dilutions of the CFS were prepared with sterile deionized water. Wells in the S. aureus ATCC 25923-seeded plates were loaded with 95 µL of each dilution of the CFS (95 µL/well). Then plates were incubated at 37℃ for 24 h, and bacteriocin activity (AU/mL) was determined (Jack et al., 1995).
E. faecalis KT11 was cultured as described previously and lyophilized. The lyophilized culture samples were stored for 3 months at different temperatures (4℃, –20℃, and –80℃). During storage, culture samples were extracted at timepoints of 1, 15, 30, 60, and 90 d and inoculated into MRS broth. After incubation, CFSs were prepared as described previously, and then, the antimicrobial activity of bacteriocin KT11 was evaluated by conducting an AWDA.
E. faecalis KT11 was cultured at 37℃ for 18 h, and bacteriocin KT11 was obtained as described above. The CFS was gradually saturated in three steps, with final ammonium sulfate concentrations of 40%, 60%, and 80%, and incubated overnight at 4℃, shaking at 100 rpm. Protein precipitate was obtained by centrifugation (Hettich, Mikro200R, Germany) at 10,000 rpm at 4℃ for 1 h (Pingitore et al., 2007). The precipitate was dissolved in 3 mL of 0.1 M KH2PO4 buffer (pH 6.0) and dialyzed overnight against same buffer in SnakeSkin dialysis tubing (3.5 kDa molecular weight cut-off, Thermo Fisher Scientific, USA). The dialysate (5.5 mL) was collected and stored at –20℃ until further use. The amount of protein in the CFS, ammonium sulfate precipitate and dialysate was determined by the Bradford method (Bradford, 1976) by using a NanoDrop spectrophotometer at 595 nm (NanoDrop 2000c, Thermo Fisher Scientific, USA).
Tricine-SDS-PAGE was performed to determine the molecular weight of partially purified bacteriocin KT11 (Schägger and von Jagow (1987). The ultra-low-range molecular weight marker (M.W. 1.06–26.6 kDa, Sigma-Aldrich) was used as a protein standard. A 16% Tris-tricine gel was prepared for electrophoresis. The amount of protein in the dialysate to be loaded into the gel was calculated as 5 μg. Dialysate samples were dissolved in tricine loading buffer and loaded into the gel. After electrophoresis, the gel was sliced into two pieces. One piece of the gel was assayed for molecular weight determination of bacteriocin KT11 (Lane M and Lane 1) by staining with Coomasie blue R 250 for 3 h. The other piece of the gel (Lane 2) was not stained and was used for a direct antimicrobial activity assay (overlay method). To remove SDS from the second lane, the gel was washed three times with 1% Tween 80 for 40 min (Yamamoto, 2003), transferred into a petri dish, and then overlaid with 15 mL of soft nutrient agar (seeded with indicator test strain at 106 CFU/mL). After incubation at 37℃ for 24 h, the gel was examined for the presence of an inhibitory zone.
Mean and standard error values of two or three experiments were calculated using Microsoft Excel®. One way analysis variance (ANOVA) was performed using PASW-Statistics18-SPSS software (Hong Kong) to determine statistically significant difference (95% confidence interval) among experimental variables.
Results and Discussion
Enterococcus spp. are classified as LAB and are frequently isolated from cheese samples. These bacteria play important roles in the ripening of cheese and confer the typical taste and aroma to the product (Foulquié Moreno et al., 2006). The E. faecalis KT11 strain used in this study was isolated from an artisanal Tulum cheese produced in Kargı, Turkey. This cheese is produced from raw goat, sheep or cow milk or a mixture of these milks. The E. faecalis KT11 strain was identified in our previous project with classic biochemical and physiological tests and molecular identification techniques with species-specific primers based on 16S rDNA (Kunduhoglu et al., 2012). LAB can produce inhibitory substances such as bacteriocins, H2O2 and organic acids in growth media (Lahtinen et al., 2011). Therefore, to eliminate the inhibitory effects of H2O2 and organic acids, the CFS of E. faecalis KT11 was first treated with catalase, and the pH of the CFS was adjusted to 6.5; then, the CFS was used in antimicrobial activity tests. Catalase-free CFSs served as a control series. The antimicrobial spectrum of the CFS was determined by an AWDA against indicator test bacteria.
It is known that most bacteriocins inhibit Gram-positive bacteria, while the outer lipopolysaccharide membrane of Gram-negative bacteria acts as natural barrier against the entry of bacteriocins into the cell (Gyawali and Ibrahim, 2014; Yildirim et al., 2014). However, in our study, the CFS obtained from E. faecalis KT11 exhibited antimicrobial activity against 16 of the 30 indicator bacteria, including Gram-negative bacteria, with inhibition zones ranging from 14 to 20 mm (Table 1). Catalase-free CFSs exhibited the same activity against the indicator bacteria. The CFS inhibited 12 of the 24 Gram-positive indicator bacteria, including L. monocytogenes, S. aureus ATCC 25923 and the spore-forming Bacillus subtilis, with inhibition zones ranging from 14 to 20 mm. Similar to our results, Xi et al. (2017) reported that cell-free extracts of E. faecalis TG2 isolated from tofu showed antimicrobial activity against Gram-positive indicator bacteria such as L. monocytogenes, L. ivanovii, L. innocua, E. faecalis, Lactobacillus sp., Pediococcus sp., and Leuconostoc sp. In another study, bacteriocin obtained from a E. faecium strain isolated from donkey milk exhibited antimicrobial activity against L. monocytogenes, S. aureus and Bacillus cereus (Aspri et al., 2017). Vimont et al. (2017) reported that E. faecium LCW 44 exhibited antimicrobial activity against Clostridium, Listeria, Staphylococcus, and Lactobacillus but not against Gram-negative bacteria. Sensitivity of Listeria spp. against bacteriocins of enterococci can be explained by the close phylogenetic relationship between Listeria and enterococci (Foulquié Moreno et al., 2006).
The CFS of E. faecalis KT11 showed antimicrobial activity against 4 of the 6 Gram-negative indicator bacteria, namely, P. aeruginosa, K. pneumoniae, S. marcescens and E. aerogenes, with inhibition zones ranging from 14 to 18 mm. Similar to our results, Perumal and Venkatasen (2017) obtained enterocin CV7 from E. faecalis CV7 and reported that this bacteriocin exhibited broad antimicrobial activity against both Gram-positive bacteria (L. monocytogenes and S. aureus) and Gram-negative bacteria (Salmonella sp., S. thypi, S. enterica, E. coli and Vibrio fischeri).Abriouel et al. (2005) reported that two enterocins obtained from E. faecalis inhibited E. coli and Shigella sonnei.
The CFS of E. faecalis KT11 inhibited the growth of methicillin- and/or vancomycin-resistant test bacteria, with inhibition zones ranging from 15 to 20 mm. Similarly, Phumisantiphong et al. (2017) reported that a bacteriocin from E. faecalis EF 478 exhibited antimicrobial activity against E. faecalis and E. faecium. Additionally, they reported that this bacteriocin has an inhibitory effect on 28 of the 68 (41.1%) multidrug-resistant enterococci and vancomycin-resistant enterococci. Saelim et al. (2015) also reported that enterocin CE5-1 from E. faecium CE5-1 inhibited some vancomycin-resistant strains of E. faecalis, E. faecium, and E. gallinarum. In our study, the test strains that were most susceptible to CFS were found to be S. epidermidis (methicillin-resistant), S. warneri (methicillin- and vancomycin-resistant), S. marcescens and Enterococcus sp. (vancomycin-resistant).
Effect of hydrolytic enzymes on the stability of the bacteriocin: LAB synthesize antimicrobial substances, and many of these agents are proteinaceous bacteriocins, while others are non-protein agents such as lactic acid, H2O2 and diacetyl (Lahtinen et al., 2011). In our study, to prove the proteinaceous nature of the antimicrobial compound(s), the CFS was treated with proteolytic enzymes, and then, residual bacteriocin activity was determined against S. aureus ATCC 25923, P. aeruginosa and M. luteus test strains. The residual activity of the CFS decreased by 40% after pepsin treatment, and the activity of the CFS was found to be 100% sensitive to trypsin, α-chymotrypsin, protease and proteinase K, suggesting the proteinaceous nature of the compound (Table 2). Therefore, the CFS from E. faecalis KT11 was referred to as “bacteriocin KT11” in the rest of this text. Additionally, the CFS retained its activity after treatment with catalase and α-amylase. Similar findings have been reported in studies with LAB, where bacteriocin activity was totally abolished after treatment with various proteolytic enzymes (Aspri et al., 2017; Chen et al., 2016; Xi et al., 2017; Yanagida et al., 2005). Isleroglu et al. (2011) determined that enterocin KP, obtained from E. faecalis KP, was stable after treatment with pepsin. In a study by Gupta et al. (2016) the supernatant of E. hirae LD3 lost antimicrobial activity upon treatment with proteolytic enzymes; however, this supernatant remained active after catalase and α-amylase treatment, suggesting that H2O2 and carbohydrate moieties played no role in the activity.
Bacteriocin KT11 samples were subjected to different temperatures (60℃, 80℃, 100℃, and 121℃) for 30 min, and the residual antimicrobial activity against S. aureus ATCC 25923 was assessed by an AWDA. The results showed that bacteriocin KT11 was thermostable even at 121℃ for 30 min (Fig. 1). Effect of the different treatment temperatures towards to stability of KT11 was not statistically significant (p>0.05). However, bacteriocin KT11 lost 8.3%–11.1% of its activity after the heat treatments when compared with controls (p<0.05).
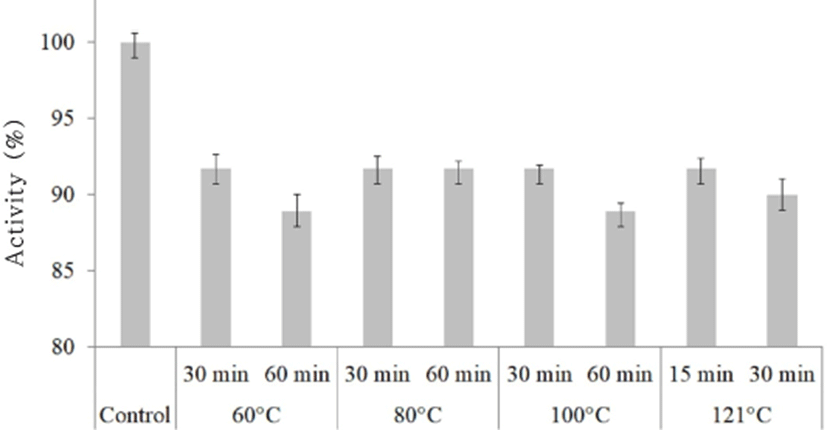
Similar to our findings, enterocins from E. durans (Du et al., 2017; Yanagida et al., 2005), E. faecium (Kumar et al., 2010), E. hirae (Gupta et al., 2016) and E. faecalis (Khalkhali and Mojgani, 2017; Xi et al., 2017) were reported to be stable for 10–20 min at 121℃. It was also determined that some enterocins were stable at lower temperatures. For example, Cocolin et al. (2007) reported that a bacteriocin from E. faecium M241 remained completely stable after a 10-min heat treatment at 100℃, while the activity decreased at higher temperatures. Phumisantiphong et al. (2017) reported that a bacteriocin that they obtained from E. faecalis EF 478 remained completely stable after heat treatment at 60℃ for 1 h, but that activity decreased at 80℃ for 1 h and at higher temperatures. Braïek et al. (2017) reported that the heat tolerance limit of the inhibitory substance obtained from the E. lactis Q1 strain was 60℃/30 min or 100℃/15 min.
Therefore, based on its proteinaceous nature and thermostability at 121℃ for 30 min, bacteriocin KT11 could be classified as a heat-stable bacteriocin.
The stability of bacteriocin KT11 at different pH levels was studied between pH 2 and 11 for 24 h. All the bacteriocin KT11 samples treated with different pH levels remained stable and showed antimicrobial activity against the indicator S. aureus ATCC 25923 strain. Effect of the different pH levels towards to stability of KT11 was not statistically significant (p>0.05). Maximum bacteriocin activity was recorded at pH 2–5. However, 5.9%–10% of the activity of bacteriocin KT11 was lost at alkaline pH levels (Fig. 2). Similarly, it has been reported that bacteriocins of E. faecium LR/6 (Kumar et al., 2010), E. faecalis CV7 (Perumal and Vankatesen, 2017), and E. hirae LD3 (Gupta et al., 2016) were stable in the pH ranges 2–6, 4–6 and 2–6, respectively. Du et al. (2017) reported that the anti-listerial activity of a bacteriocin obtained from E. durans 152 was completely retained in the pH range of 2–8.
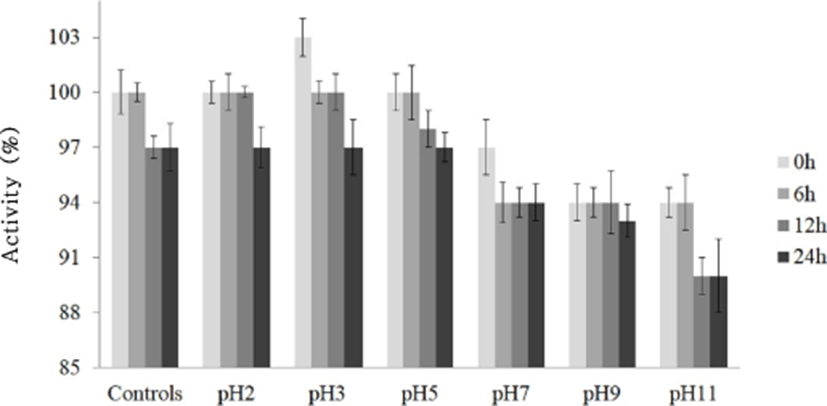
Bacteriocins that are stable over a wide pH range have a significant advantage in terms of their potential use as bio-preservatives in food products and fermented foods (Franz et al., 1996). Therefore, bacteriocin KT11 has promising potential for use as a preservative in foods of a very wide pH range.
The activity of bacteriocin KT11 samples treated with chloroform, methanol, ethanol, hexane or acetone decreased approximately 8%–13% (Fig. 3). Bacteriocin KT11 was completely stable after other solvent treatments. Similar to our findings, it has been previously reported that enterocins remain completely stable after treatment with organic solvents (Isleroglu et al., 2011; Perumal and Vankatesen, 2017). The stability of bacteriocins after treatment with solvents proves that bacteriocins do not have lipid moieties (Yildirim et al., 2014). This finding is also important because many organic solvents are used in bacteriocin purification from supernatants (Aspri et al., 2017).
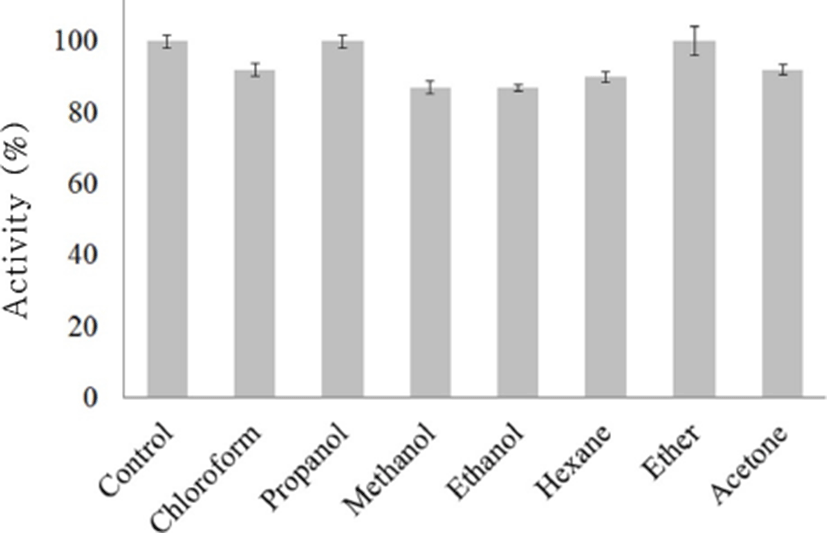
Bacteriocin KT11 samples were treated with various surfactants at 37℃ for 180 min, and residual antimicrobial activities were determined. Overall, it was observed that the activity against S. aureus ATCC 25923 was completely (100%) preserved after these treatments. Similar to our findings, it has been previously reported that enterocins remain stable after treatment with surfactants (Isleroglu et al., 2011; Kumar et al., 2010; Perumal and Vankatesen, 2017).
E. faecalis KT11 was cultured for 24 h, and aliquots were obtained at regular intervals. At each sampling timepoint, the bacteriocin activity (AU/mL) against S. aureus ATCC 25923 and the optical density of the CFS was determined (Fig. 4). Bacteriocin KT11 production started during the early exponential phase, and the bacteriocin activity was determined to be 22 AU/mL. The maximum bacteriocin activity (178 AU/mL) was observed between the late exponential and early stationary growth phases (18–22 h of incubation), and the bacteriocin activity decreased after 22 h. The maximum cell count was observed after 20–24 h of incubation. Extended growth did not lead to higher levels of bacteriocin activity. In some studies, similar growth and enterocin production kinetics were reported for E. faecalis MR99 (Sparo et al., 2006), E. faecium MMT21 strains (Ghrairi et al., 2008) and E. lactis Q1 (Braïek et al. (2017). It has been thought that decrease in bacteriocin activity might be due to digestion of the antimicrobial compounds by proteases released from the cells, protein aggregation, adsorption to cell surface or feedback regulation (Todorov and Dicks, 2006; Gong et al., 2010).
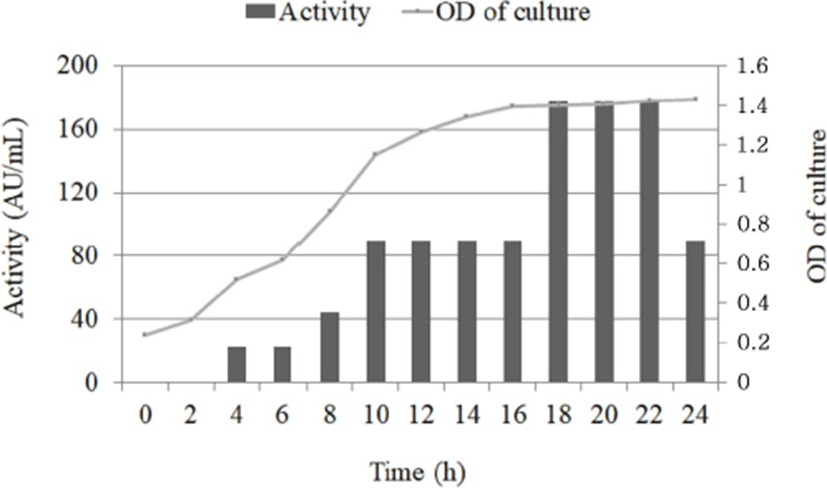
For potential application of bacteriocin KT11, it has to be produced on a large scale and must be properly concentrated and stocked without losing antimicrobial activity during storage. For this reason, bacteriocin KT11 was lyophilized and stored at different temperatures for 90 d, and the residual antimicrobial activity was determined. Although the antimicrobial activity of lyophilized bacteriocin KT11 stored at 4℃ was slightly reduced (p>0.05), 98%–100% of the activity was preserved at the other storage temperatures (–20℃ and –80℃) after 90 days (Fig. 5). Therefore, lyophilized bacteriocin KT11 can be stored at –20℃ and –80℃ for at least 90 days without loss of activity. Similar studies have shown that enterocins from E. faecalis (Phumisantiphong et al., 2017) and E. faecalis KP (Isleroglu et al., 2011) did not lose their activities at 4℃ for 6 months/–20℃ for 1 year and –20℃/–80℃ for 2 months, respectively. Kumar et al. (2010) reported that an enterocin from E. faecium LR/6 retained complete activity after one year at 4℃/–20℃.
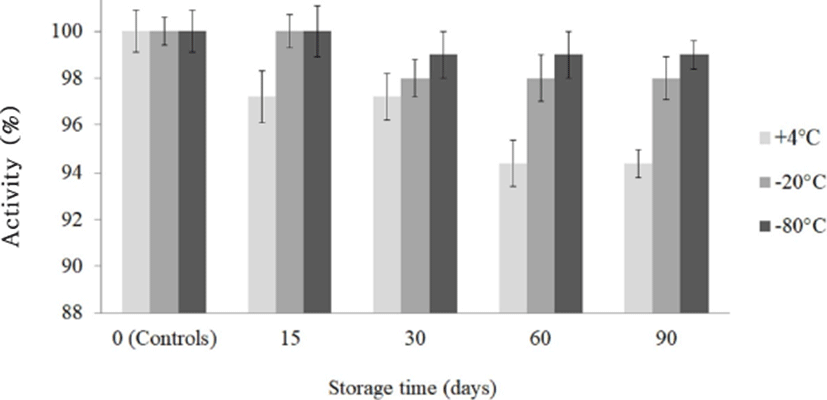
To purify the antimicrobial protein, the CFS (50 mL) was gradually saturated in three steps with ammonium sulfate at final concentrations of 40%, 60%, and 80%. Then, the ammonium sulfate precipitate was dissolved in 3 mL of 0.1 M K2HPO4 buffer (pH 6.0), desalted by using 3.5-kDa cut-off dialysis membrane and separated by Tris-tricine-SDS-PAGE. The amount of protein in the CFS at specific purification stages was determined by the Bradford method. S. aureus ATCC 25923 was used to test bacteriocin activity at each purification level of bacteriocin KT11 (Table 3). It was determined that the activity increased as the purification steps increased. While bacteriocin activity was 178 AU/mL in the CFS, the activity was 711 AU/mL in dialysate.
d Specific activity (AU/mg): Total activity of the subsequent purification step/total protein of the same step.
Tris-Tricine SDS-PAGE was performed to determine the molecular weight of partially purified bacteriocin KT11. The amount of protein in the dialysate to be loaded into the gel was calculated to be 5 μg, and the dialysate samples were dissolved in tricine loading buffer. After electrophoresis, the gel was sliced into two pieces. The marker lane (M) and the first lane (lane 1) of gel were assayed for molecular weight determination of bacteriocin KT11 by staining with Coomassie blue. The second lane (lane 2) of gel was not stained and was used for a direct antimicrobial activity assay (overlay method) and examined for the presence of an inhibitory zone. One antimicrobial peptide band was observed on the overlaid SDS-PAGE gel (Fig. 6). Based on the SDS-PAGE analysis, it can be concluded that the peptide responsible for antimicrobial activity has a molecular size of ~3.5 kDa. The molecular weights of enterocins obtained from some Enterococcus strains have been reported to be as follows: ~6.0 kDa from E. faecium LR/6 (Kumar et al., 2010), 5.8 kDa from E. faecalis KP (Isleroglu et al., 2011), 5 kDa from E. durans (Du et al., 2017) and 4.829 kDa from E. faecalis CV7 (Perumal and Venkatasen, 2017). Yanagida et al. (2005) identified two peptides with antimicrobial activity from E. durans L28-1 strain that had molecular weights of 2.5 and 3.4 kDa.
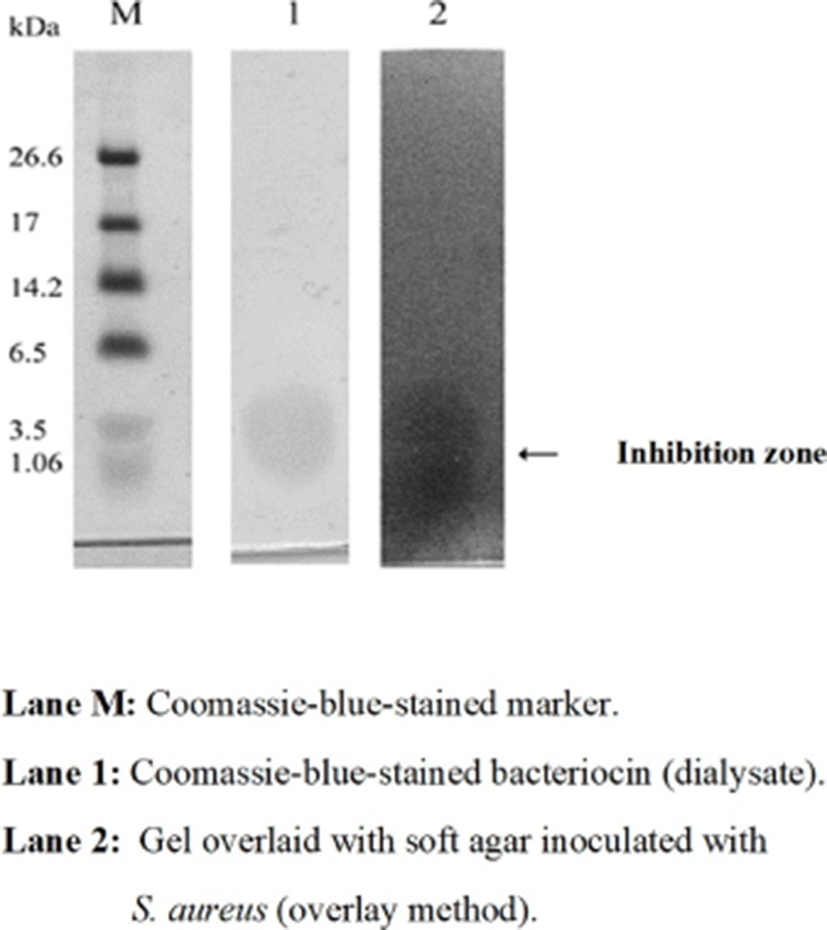
Conclusion
The bacteriocin obtained from E. faecalis KT11 had a broad antimicrobial spectrum, and inhibited foodborne pathogens and vancomycin- and/or methicillin-resistant bacteria. The sensitivity of the CFS to proteolytic enzymes demonstrated the proteinaceous nature of the antimicrobial compound. One peptide band that exhibited antimicrobial activity, with a ~3.5 kDa molecular weight, was identified after tricine-SDS-PAGE analysis. Therefore, the partially purified substance was referred to as “bacteriocin KT11”. Bacteriocin KT11 was highly heat-stable (121℃ for 30 min) and maintained its activity in a pH range from 2.0 to 11.0. Additionally, bacteriocin KT11 was stable after treatment with some surfactants and organic solvents. Moreover, the activity of lyophilized bacteriocin KT11 was stable after three months of storage at –20℃ and –80℃.
In conclusion, due to its broad antimicrobial spectrum and stability at high temperatures and over a wide pH range, bacteriocin KT11 is thought to be a potential efficient bio-preservative in various food products. Furthermore, the fact that bacteriocin KT11 is effective against the drug-resistant pathogens used in this study makes it a promising antimicrobial agent in combating multidrug-resistant pathogens. The utilization of bacteriocin KT11 alone or in combination with conventional antibiotics may be an effective therapeutic option for the treatment of multidrug-resistant clinical pathogens. However, in future studies, bacteriocin KT11 should be purified and identified. In addition, the antimicrobial effectiveness of bacteriocin KT11 should be evaluated in vivo.