Introduction
During the manufacture of fermented dairy products such as yogurt and cheese, change of pH, particularly acidification, occur frequently, affecting the integrity and physicochemical properties of casein micelles through the solubilization of colloidal calcium phosphate (CCP) (Lucey et al., 1996). A pH decline may also occur during heating of dairy products (> 90°C for several minutes), caused by lactose degradation and calcium phosphate precipitation onto the casein micelles (Pouliot et al., 1989).
Buffering refers to resistance to pH change. In dairy products, the buffering capacity (BC) corresponds to the sum of individual ionizable acid-base groups, for which salt, organic acids, and proteins are major contributors (Salaun et al, 2005). The BC exerts a considerable influence on the quality characteristics of dairy products, by altering rate of pH decline or by determining final pH of acidified dairy proteins. For instance, the rate of acid production will likely influence the microstructure of the acid curd present in yoghurt (Kailasapathy and Rybka, 1997). The BC of cheese curd during the maturation stage plays an important role in cheese quality by modulating enzyme activity and microbial growth (Lawrence and Gilles, 1982). In addition, cheese with a high BC will more likely ensure the survival of the beneficial microorganisms in the gastric passage.
Currently, there are a few studies investigating the BC of dairy powders with different constituents and manufactured under different conditions. The present study aimed to examine the changes in the BC of reconstituted skim milk powder (SMP) and whey powder (WP) mixtures and investigate the effects of heating on BC. In addition, we examined changes in the quality characteristics of high-buffering yoghurt including microstructure, water holding capacity (WHC), and viable cell count.
Materials and Methods
SMPs with different heating strength (low-, medium- and high-heat) were provided by Lactoland Trockenmilchwerk GmbH (Germany). Sodium-caseinate (Na-CNate) and WPs were obtained from Arla Food Ingredient (Denmark). Whey protein concentrate (WPC) was provided by Meggle GmbH (Germany).
Four different mixtures of SMP (Seoul dairy Coop., Korea) and DWP90 (90% demineralized whey powder) were prepared by mixing the two powders at the ratios (w/w) of 88:12, 76:24, 64:36, and 52:48 (SMP:DWP90). Each powder mixture (0.5%, w/v, solid basis) was reconstituted by adding distilled water and stirring until complete dispersion.
Samples (0.5%, 50 mL) were titrated to pH 2.0 with 0.5 M HCl. Titration was performed using a Titrino 702 SM Autotitrator (Metrohm, Switzerland) at 25°C under continuous stirring. The titer was added in 20 μL increments, with a 10 s equilibration after each addition. Buffering index values (dB/dpH), defined as BC, were calculated using the following formula (Van Slyke, 1922).
To determine heat-induced changes in the BC, the samples were heated at 85°C for 15 min. The heated samples were immediately cooled to room temperature using ice water, and the BC was determined at 25°C. All measurements were conducted in triplicates.
High-buffering fresh milk was prepared by adding 40 mM NaH2PO4 and 60 mM Na2HPO4 to fresh milk. The pH of high-buffering fresh milk was 6.6. High buffering yoghurt was prepared by inoculating a commercial starter culture (0.06%, ABT-C1, Culture Systems, Inc., Indiana, USA) into high-buffering milk. Fermentation was continued in a 42°C water bath until the pH reached 4.5. Control yoghurt was prepared from fresh milk using the same procedure. The pH changes of samples were monitored using a pH meter and yoghurt samples were stored in a refrigerator immediately after fermentation (pH 4.5).
The WHC of the yoghurt samples was determined by a method of Shori et al. (2013) with slight modifications. Briefly, following completion of fermentation, the yoghurt samples were kept in a refrigerated storage 12 h, after which the samples (10 g) were subjected to centrifugation at 9,800×g for 20 min at 4°C. The separated whey was carefully collected and weighed. The WHC of samples was calculated using the following formula:
The microstructures of the yoghurt samples were observed using confocal laser scanning microscopy according to a previous method (Lee and Lucey, 2004). Briefly, acridine orange (300 μL, 0.2%, w/w, Sigma-Aldrich Inc., St. Louis, USA), a fluorescent protein dye, was added to the samples (50 mL), allowing the visualization of the yoghurt microstructure under an LSM 700 confocal microscope (Carl Zeiss, Germany) equipped with the ZEN 2.1 lite software. Representative images were obtained at an excitation wavelength of 405 nm and an emission wavelength of 460 nm through a 63× water-immersion objective lens (numerical aperture = 1.0).
The quantification of microorganisms in the yoghurt samples was conducted after 12 h of refrigerated storage. For this purpose, each sample (1.0 mL) was added to 9.0 mL of sterilized physicological saline (0.85% NaCl). Streptococcus thermophilus, Lactobacillus acidophilus, and Bifidobacterium longum were counted on M17 agar, MRS-maltose, and BL-NPNL agar, respectively and the plates were incubated under anaerobic conditions at 37°C for 72 h (Marafon et al., 2011). After incubation, the plates containing 30-300 colonies were enumerated and the counts were expressed as Log10 CFU/mL of yoghurt.
The results were expressed as the mean value ± standard deviation. All statistical analysis was performed using SPSS (SPSS Inc. ver.21, USA). When data shows significant differences (p<0.05) in ANOVA, Duncan’s multiple range test was carried out to find significant difference among the means. A paired t-test was used to compare the heat-induced BC changes in SMP:WP mixtures and compare the BC of control and high- buffering milk.
Results and Discussion
The BCs of various dairy ingredients are shown in Fig. 1. The results from the triplicate measurements showed that the calculation of BC was highly reproducible with little variation. Na-CNate had the highest BC of the tested samples. WPC, which has greater protein content showed a higher BC than those of SMPs and WPs. In general, sample components that act as acid-base groups contribute to the BC. Thus, the BC of dairy ingredients is determined by summing up the individual acid-base constituents within each product. The relative contribution of milk constituents for the BC is ranked as follows: soluble minerals (40%), caseins (35%), CCP (20%), and whey proteins (5%) (Salaun et al., 2005). The BC of Na-CNate was significantly higher than that of WPC, even after the total protein content of Na-CNate was adjusted to the same as WPC (78%) (data not shown). In the acidic pH range, casein micelles display a maximum BC at pH 5.2 due to CCP and phosphoserine residues, whereas whey proteins have a maximum BC at pH 3-4 due to acidic amino acids (Metwally and Awad, 2001).
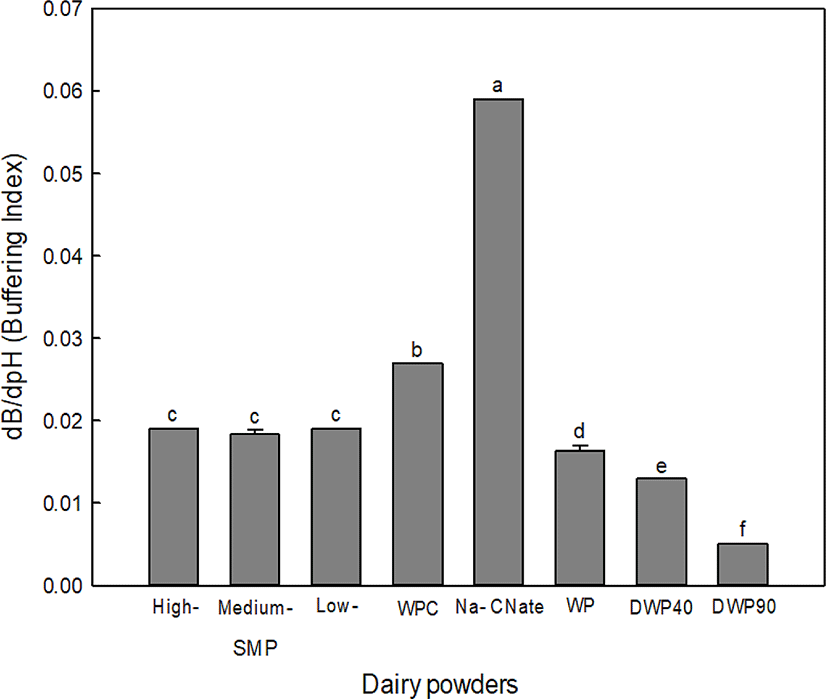
No significant differences were found regarding the BC of SMPs propduced under different heating conditions. Traditionally, SMPs are calssified as low-, medium-, high-heat powders according to the whey protein nitrogen index (WPNI). The WPNI indicates the contentration of undenatured whey protein and low, medium-, and high-heat SMPs have a WPNI≥6.00, 1.51-5.99, and≤1.50 mg per g powder, respectively (Caric and Kalab, 1987). Accordingly, the heating conditions typically used at SMP manufactures (70°C/15 s, 85°C/60 s, and 90°C/5 min for low-, medium-, and high heat-SMP, respectively; Patel et al., 2007) did not lead to significant differences in the BC of SMP. The BC of WPs was negatively proportional to the extent of demineralization. The BC of DWP90, which presents the highest demineralization among commercial WPs, corresponded to 58% of non-demineralized WP. In WP, the BC was found to be correlated with the ash content (r2=0.90) (data not shown) suggesting that the BC values of WP determined under standardized conditions can be used to confirm the degree of demineralization in WP products during quality assurance procedures.
The effect of heating on the BC of SMP, DWP90, and their mixtures was examined after heating the reconstituted powders at 85°C for 15 min. As shown in Fig. 2A, the samples containing both caseins and whey proteins showed a significant decrease in the BC, whereas DWP90, which only contained whey proteins, was not significantly affected by heating. The effect of heating on the BC increased with the proportion of whey proteins in the samples increased (Fig. 2B). This result suggests that the interaction between caseins and whey proteins may underlie the heat-induced BC reduction. The presence of ionizable acidic and basic amino acids in proteins contributes to the BC, and the availability of these ionizable amino acids to protonation can be reduced by heat treatment (Salaun et al., 2005). It is well known that β-lactoglobulin, a major whey proteins, readily interacts with κ-casein forming a protein complex on the surface of casein micelles in heated milk (Hill, 1989). The molar ratio for the complex formation was estimated to be ≅1.5 mole whey protein/κ-casein (Corredig and Dalgleish, 1996), indicating that the complex formation of such a complex can be accelerated via an incresase in the proportion of whey proteins in the samples up to κ-casein saturation. The SH-SS interchange has been suggested as a major interaction force underlying complex formation, but ionic and hydrophobic interactions are also involved in the association between denaturaed whey proteins and casein micelles (Mulvihill and Donovan, 1987). Furthermore, heat-induced changes in the equilibrium between calcium and phosphate may alter the BC of samplers, although a previous study by Li and Corredig (2014) reported that heating of UF-concentrated milk (2X) at 80°C for 15 min did not lead to significant differences in the CCP equilibrium.
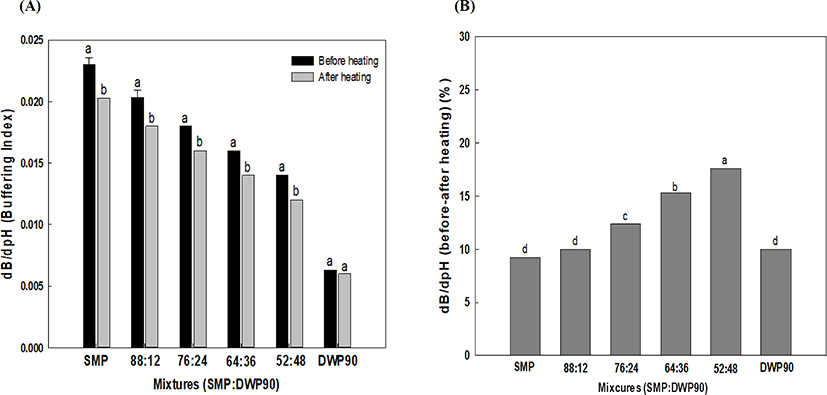
The high-buffering milk was prepared by adding phosphate salts to fresh milk and high-buffering yoghurt was made by adding a commercial starter. The addition of phosphate salts significantly increased the BC of milk, in that the BC of high-buffering milk was approximately 2 times higher than that of fresh milk (Fig. 3A). The pH of milk and high-buffering milk was 6.6 and 6.77, respectively. The high-buffering milk presented a lower speed of acid production during fermentation, as the time to reach pH 4.5 was increased from 8 to 12 h (Fig. 3B).
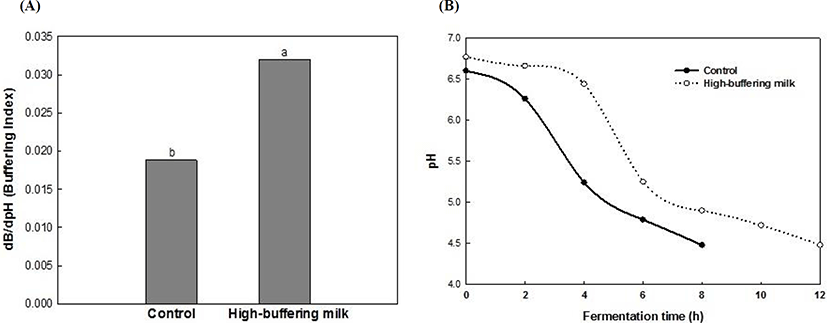
Phosphate is often added to concentrated dairy products to improve heat stability (Augustin and Clarke, 1990). In high-buffering milk, an increased BC was observed at both neutral (near pH 6.5) and acidic pH (pH 4.5-5.0), probably due to the ionization state of H2PO4- and HPO42-. Upreti et al. (2006) reported that calcium and phosphate treatment in cheese manufacture increases the BC when the pH ranges between 4.5 and 5.5.
Both texture and sensitivity to syneresis are important quality characteristics in yoghurt. Fig. 4 indicates WHC of samples, as determined 12 h after completion of fermentation. The WHC of high-buffering yoghurt was significantly higher than that of the control yoghurt. This result suggests that high-buffering yoghurt presents a reinforced protein network because whey separation under centrifugal force is closely related to the rigidity of the casein matrix (Harwalkar and Kalab, 1986).
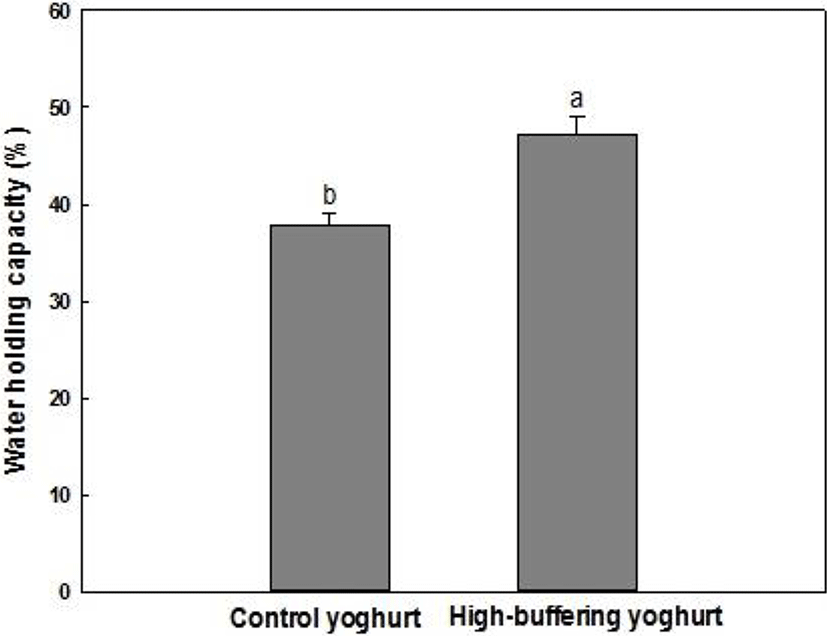
The actual microstructure of yoghurts was examined using confocal laser scanning microscopy. As shown in Fig. 5, compared with the control yoghurt, the high-buffering yoghurt has a more interconnected, uniform structure with smaller pores, which may be related to an improved WHC. Lee and Lucey (2004) reported that in acid milk gels, rapid acidifying conditions such as high temperature and high starter concentration, resulted in increased whey separation by allowing a rapid rearrangement of the casein network. In this context, a delay in acidification may account for the improved WHC observed in the high-buffering yoghurt.
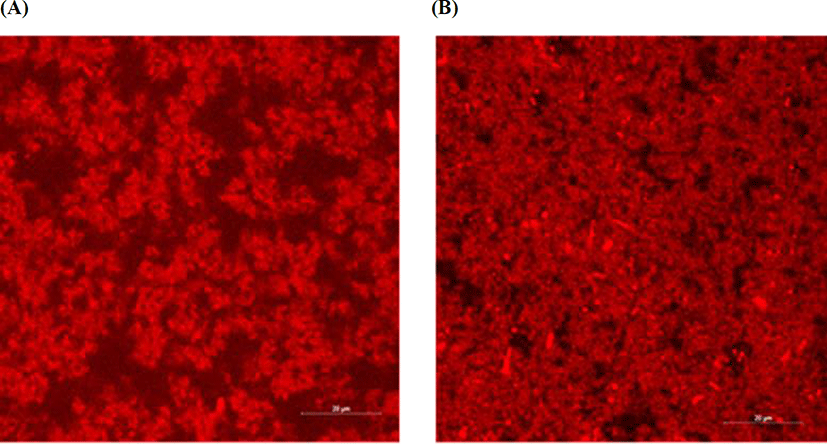
The number of viable cells (Str. thermophilus, L. aciodphilus, and B. longum) in the yoghurts was determined. As shown in Table 1, no significant differences were found regarding viable cell counts, indicating that bacterial growth was not affected by the addition of phosphate. The number of live bifidobacteria was within the recommended level (6-8 Log10 CFU/mL) (Vasiljevic and Shah, 2008). Martini et al. (1987) reported that the activity of β-galactosidase in yoghurt was significantly decreased when the pH was ≤4.0. This result suggests that the BC of yoghurt may influence lactose digestion particularly in lactase-deficient subjects, by maintaining β-galactosidase activity in yoghurts. The proper manipulation of the BC in yoghurt may improve the survival of beneficial bacteria particularly concerning long-term storage and temperature-abused conditions. However, further research is needed to investigate the relationship between the BC and the survival of probiotics in yoghurts during storage.
Conclusion
The manufacturing conditions influence the BC of dairy powders. Preheating conditions (low-, medium-, and high heat-) did not show significant difference on BC of SMPs whereas the level of demineralization significantly affect the BC of WPs. The heat treatment (85°C for 30 min) of SMP and DWP90 mixtures resulted in a decreased BC, and the extent of this reduction increased as the proportion of WP increased. The manipulation of BC in yoghurt manufacture has the potential to improve the quality characteristics of yoghurt including WHC and texture.