Introduction
Turmeric (Curcuma longa L.) is a plant belonging to the Zingiberaceae family, which is cultivated mainly in South and East Asia, and used as a spice and traditional medicine. The yellow color characteristic of turmeric is given by the pigment curcumin and its analogs demethoxycurcumin and bisdemethoxycurcumin. These compounds are known as curcuminoids and have been reported to exhibit various biological activities including antiinflammatory, antibacterial, anticancer, and antioxidant activities (Anand et al., 2008; Araújo and Leon, 2001). Nevertheless, these compounds exhibit poor water solubility and low bioavailability (Wang et al., 2008). The chemical and metabolic instability of curcumin are also factors that call into question the therapeutic efficacy of this compound (Nelson et al., 2017). By contrast, turmeric extract is composed of a complex mixture of compounds, which may act synergistically (Chempakam and Parthasarathy, 2008; Račková et al., 2009); thus, using turmeric extract instead of isolated curcuminoids appears to be a better course of action for developing bioactive formulations. Turmeric, unlike isolated curcuminoids, is considered a GRAS product by the United States Food and Drug Administration (FDA), and would be a better choice for the development of functional foods.
Considering the poor water solubility of turmeric extract and its compounds, it is necessary to develop delivery systems capable of protecting the bioactive agents and controlling their release in the gut. Nanoemulsions are emulsions in which the droplets are sized at the nano-level (<200 nm) and have been used for encapsulating various lipophilic bioactives (Solans et al., 2005) including curcumin (Aditya et al., 2015; Ahmed et al., 2012). The small particle size of nanoemulsions increases their stability, showing less flocculation and separation than do conventional emulsions, and can also increase the bioaccessibility of lipophilic compounds like curcuminoids (Aditya et al., 2015; Solans et al., 2005). Nevertheless, the development of such delivery agents is only a step in the production of actual functional products, and the final goal would be incorporating these nano-carriers into foods and drinks that can provide health benefits to the consumers. Hence, the developed nanoemulsions have to be spray dried to reduce storage and transportation costs and facilitate their incorporation into foods (Jeong et al., 2017).
Therefore, this study aimed at producing nanoemulsions for encapsulating turmeric extract, and incorporating the dried encapsulated extract into minced pork to make functional canned ham. Considering that the ham would be directed to Korean consumers, Korean turmeric was used for developing the formulation as it is preferred there because of its mild color and flavor. The physicochemical characteristics of the nanoemulsions were determined, and sensory evaluation of the canned ham was also performed.
Materials and Methods
Vegetable oils (olive, grape seed, corn, and soybean) and turmeric powder produced from Jindo in Korea were procured from Ottogi Co. Ltd. (Korea). Oleic acid was obtained from Duksan Pure Chemicals Co. Ltd. (Korea). Tween 20 was purchased from Daejung Chemicals & Metals Co. Ltd. Lecithin was obtained from ESFOOD (Korea) and whey protein isolate (WPI) was purchased from Sungpoong (Korea).
Firstly, turmeric was extracted by immersing the turmeric powder in 50% ethanol at room temperature for 24 h. The extract was filtered and concentrated using a rotary evaporator under reduced pressure to increase the curcumin content (20 brix). Then, it was spray dried with 20% (w/v) dextrin. Final curcumin content in the turmeric extract was measured using HPLC analysis.
The solubility of the turmeric extract in oleic acid, and olive, grape seed, corn, and soybean oils was evaluated. Turmeric extract (1%, w/v) was added to each oil and the mixture was magnetically stirred at 60°C for 10 min. The resulting slurry was centrifuged at 12,000 rpm using a bench top centrifuge (1248R, Gyrozen, Korea) at room temperature for 10 min to remove undissolved material. To determine the curcumin content, the sample was prepared by adding 900 μL of 100% DMSO to 100 μL of the TE-NEs and vortexing thoroughly. A high-performance liquid chromatograph (HPLC; Shimadzu D-20A, Japan) fitted with a UV absorbance detector and an ACE5 C18 column (4.6×250 mm, 5 μm; Advanced Chromatography Technologies, UK) was used. The mobile phase was acetonitrile and 2% acetic acid (65:35, v/v), pumped at a flow rate of 1.0 mL/min. A sample volume of 20 μL was injected, and the detector was operated at 265 nm.
Four formulations were prepared, varying the ratios of surfactants: control (lecithin:Tween 20, 1:1), Form-A (lecithin:Tween 20: WPI, 1:1:1), Form-B (lecithin:Tween 20: WPI, 0:1:2), Form-C (lecithin:Tween 20:WPI, 1:0:2). To prepare the TE-NEs, the oil phase was first prepared by dissolving 1% (w/v) turmeric extract in oleic acid containing soy lecithin (lipophilic surfactant). The aqueous phase was prepared by mixing Tween 20 and whey protein isolate (hydrophilic surfactants) with distilled water (Tween 20 was omitted in one of the formulations tested). The mixture was subjected to magnetic stirring at room temperature for 2 h to obtain a coarse emulsion. Nanoemulsions were then prepared by further homogenizing the coarse emulsion. The mixture was first subjected to high-speed homogenization (HSH) (HG-15D, Daihan Scientific Co. Ltd., Korea) at 5,000 rpm for 10 min and then to ultrasonication (US) with a Vibra Cell (VCX-750, Sonics & Materials, Inc., USA) operated at 750 W and 40% amplitude for 15 min.
To be able to produce TE-NE for commercial purposes, the process should be scaled-up. Thus, the total volume of the laboratory scale production was fixed to 30 mL, whereas the large scale production was increased to 10 L using a manufacturing equipment composed of a mixing module with a heating system and an ultrasonication module with a cooling system. All connecting pipes and containers were double-walled allowing for temperature control during stirring and US. For the laboratory scale production, the module was used at 20 kHz and amplitude of 40% for 15 min. The scaled-up process was conducted in two steps. In the first step, the oil phase and aqueous phase were mixed at 75°C for 2 h. In the second step, the mixed solution was homogenized by US at 20 kHz, amplitude of 80%, and pulse on/off of 3 s/2 s for 5 h.
The scaled-up nanoemulsions were spray dried in a pilot dryer (KL-8, Seogang Engineering Co., Ltd., Korea). The dimensions of the drying chamber were H 1.1 m × L 0.8 m. The produced TE-NEs were combined with dextrin at a 100% concentration (w/w, based on the nanoemulsion as dry solids) and stirred overnight to lower the viscosity of the mixture and prevent aggregation. The initial liquid emulsion was atomized with a rotary atomizer (diameter = 5 cm, 24 rectangular vanes of 3.5 × 6 mm) operated by compressed air. The injection temperature of the spray drier was set at 180°C and the release temperature was set at 100°C. The TE-NE powders obtained were re-dispersed in water before analysis.
The mean droplet size, PDI, and zeta potential of the TE-NEs and re-dispersed TE-NE powders were determined by dynamic light scattering (ELSZ-2000, Otsuka Electronics, Japan). A 1-mL sample was loaded onto a polystyrene latex cell, and the mean droplet size and PDI were measured at 25°C at a detector angle of 90°and wavelength of 633 nm. Each sample was measured at least 3 times and the average values were used. The mean droplet size was expressed as the Z-average diameter. The PDI indicated the particle size distribution. To measure the zeta potential, the sample was diluted 10-fold in distilled water, 1 mL of the diluted sample was loaded onto a disposable cell, and the surface charge was determined by measuring the electrophoretic mobility at 25°C.
The particle size and droplet shape of the TE-NEs and TE-NE powders were determined by transmission electron microscopy (TEM; HT 7700, Hitachi, Ltd., Japan). The samples were diluted 10-fold, and a drop of the diluted sample was applied onto a carbon-coated 300-mesh copper grid, which was kept under ambient conditions for 30 s. Phosphotungstic acid was applied to the grid for 10 s as a negative staining agent, and the grid was then dried overnight and imaged.
Pork meat was purchased from a local market and kept in a refrigerator until use. The meat was chopped into small pieces (4-5 mm), weighed, mixed with TE-NE powder (or TE) and other ingredients under vacuum and −20°C, aged for 18-28 h, canned, and sterilized at 121°C for 22 min.
Color values were obtained using a colorimeter (Color-flex, USA) after standardizing its original value with a standard plate. The L*, a*, and b* values were determined as indicators of lightness, redness, and yellowness, respectively. All samples in the experiment were tested in triplicate.
For the sensory test, samples of canned ham supplemented with turmeric extract (TE) and TE-NE powder were made with the previously mentioned procedure. The sensory panel was composed of 11 well-trained researchers from the Ottogi Research Center, who assessed the hams in terms of their appearance (color), off-flavor, and overall acceptability. Uncooked and cooked hams were evaluated randomly by the panelists. Samples were served with a glass of water for palate cleansing, and were left at room temperature for 10 min before the evaluation. The appearance, off-flavor, and overall acceptability were evaluated on a 10-point scale (1 = the least/lowest, 5 = moderate, 10 = the most/highest). A randomized and composed block design was used.
All measurements were performed in triplicate using freshly prepared samples and were reported as means and standard deviations (mean ± SD). Statistical analysis was performed by one-way analysis of variance (ANOVA) using the SPSS software (SPSS Inc., USA). Duncan’s multiple range test was used to detect significant (p<0.05) differences among the mean values of the samples.
Results and Discussion
Nanoemulsions are carrier systems composed of an oil phase and a water phase kept together by surfactants, and differ from traditional emulsions in that the droplets are in the nanometer scale (Solans et al., 2005). Turmeric extract is a complex mixture of active compounds called curcuminoids, water, carbohydrates, minerals, and other components (Chempakam and Parthasarathy, 2008). Thus, an appropriate oil phase capable of encapsulating whole turmeric extract was required in this study. The solubility of turmeric extract in different oil types was measured to identify the most appropriate oil phase. The optical density (OD) value was used to indicate the concentration of dissolved turmeric extract. Oleic acid, corn, grape seed, olive, and soybean oils were screened. Oleic acid exhibited the highest OD value (1.07), followed by soybean oil (0.78), whereas olive oil showed the lowest OD value (0.59) as shown in Fig. 1. These results indicate that the solubility of the turmeric extract in oleic acid was substantially higher than that observed in other oils, and thus, oleic acid was used for the formulation. Oleic acid is a monounsaturated fatty acid that is an important component of olive oil, and its ingestion has been associated with health benefits including lowered blood pressure and a decrease in the risk of heart disease (Lopez-Huertas, 2010; Terés et al., 2008).
The current nanoemulsion system consisted in an oil-in- water dispersion. At the initial stage of development, soy lecithin and Tween 20 (1:1) were used as surfactants. Several formulations with varying water contents were evaluated. It was observed that the particle diameter tended to decrease as the water content increased, with the smallest droplets (<170 nm) corresponding to the formulation with 90% water. By contrast, the formulation containing 75% water exhibited notably bigger droplets (>1000 nm) that were outside the “nano” range and a high PDI (0.5). The formulation containing 85% water exhibited small droplets (<300 nm) with a low PDI (0.23-0.33) and high zeta potential (average of -35 mV) (Fig. 2), and was thus chosen for further experiments. This formulation contained 6% oleic acid, 4.5% of each lecithin and Tween 20, and 0.45 g of turmeric extract. Small droplets are desirable as they increase the stability and transparency of the nanoemulsion as well as the bioavailability of the encapsulated compound (Mayer et al., 2013). A low PDI value indicates homogeneity in the size distribution, whereas a high zeta potential is a measure of the surface charge of the droplets and their stability (McClements, 2013). The fact that a greater water content resulted in large droplets with a low zeta potential may be explained by the instability of the emulsion, which led to droplet coalescence and an increased particle size (Rezaee et al., 2014).
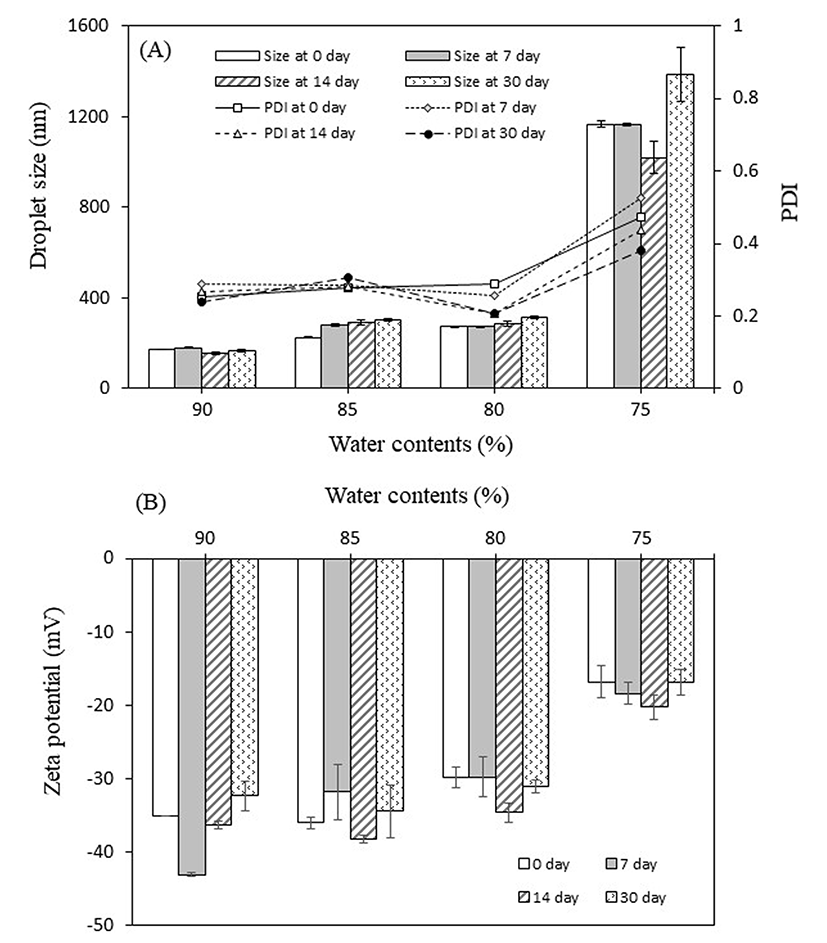
In addition, the storage stability of the TE-NEs was evaluated at 4°C during 30 d. As a result, only small variations in particle diameter were observed for the formulations containing 80-90% water, whereas that containing 75% water exhibited a decrease in particle diameter after 14 d, followed by a substantial increase by the end of the experiment, reaching a diameter of 1385 nm. In addition to coalescence, Ostwald ripening may have contributed to this increase in size (Rezaee et al., 2014; Solans et al., 2005). By contrast, the zeta potential tended to increase in the middle of the evaluation period for all formulations, followed by a decrease after 30 d of storage. The formulation containing 85% water exhibited the highest zeta potential (−34.5 mV) after 30 d of storage, whereas that of the formulation with 75% water remained the lowest throughout the evaluation period (Fig. 2). Therefore, the formulation containing 85% water also demonstrated to remain stable during storage.
The formulation was first designed with a water content of 85% and an oil: surfactant ratio of 1:1.5, using equal amounts of Tween 20 and lecithin. Tween 20 is a non-ionic surfactant that is considered to be safe for food applications; however, it was reported that it can induce cell apoptosis (Eskandani et al., 2013), which is of concern as its carcinogenic potential has not been thoroughly studied. In addition, consumers nowadays prefer more natural foods, free of artificial additives. Therefore, we aimed to replace the Tween 20 with whey protein isolate (WPI). Whey contains various proteins, among which β-lactoglobulin and α-lactalbumin predominate (Livney, 2010). Whey proteins are negatively charged emulsifiers that exhibit high water solubility over a wide range of pH and can readily adsorb to the droplet surface, decreasing the surface tension, and preventing aggregation; thus, they have been successfully used to prepare nanoemulsions (Teo et al., 2016).
To determine the effect of replacing the Tween 20 with WPI and determine the feasibility of such a formulation, surfactant ratios of 1:1:1, 0:1:2, and 1:0:2 of lecithin: Tween 20: WPI were evaluated and identified as Form-A, -B, and -C, respectively (Fig. 3). As a result, Form-B resulted in the droplets with the smallest diameter (104.2 nm) and PDI (0.15), whereas the other two formulations, which contained lecithin, exhibited substantially larger diameters. This agrees with a previous study that reported that using protein emulsifiers resulted in smaller droplets than those attained with other stabilizers (Teo et al., 2016). The high surface activity of WPI leads to the formation of thin interfacial layers and small particles (Ozturk et al., 2015a). The formulation without Tween 20 (Form-C) resulted in droplets with a diameter of 165.3 nm, which was still in the range considered optimal (<200 nm) for attaining greater intestinal residence time and absorbability (Ensign et al., 2012) as well as a low PDI of 0.17, indicating a homogeneous particle size distribution. In addition, the zeta potential (−31.80 mV) was high, indicating that the emulsion was stable (Park et al., 2017). The negative zeta potential was expected as lecithin and WPI are negatively charged (Hu et al., 2016; Teo et al., 2016). In summary, the optimized formulation (Form-C) was composed of 25.5 g water, 1.8 g oleic acid, 0.45 g turmeric extract, 0.9 g lecithin, and 1.8 g WPI, equivalent to an oil: surfactant ratio of 1:1.5, and was used for further experiments.
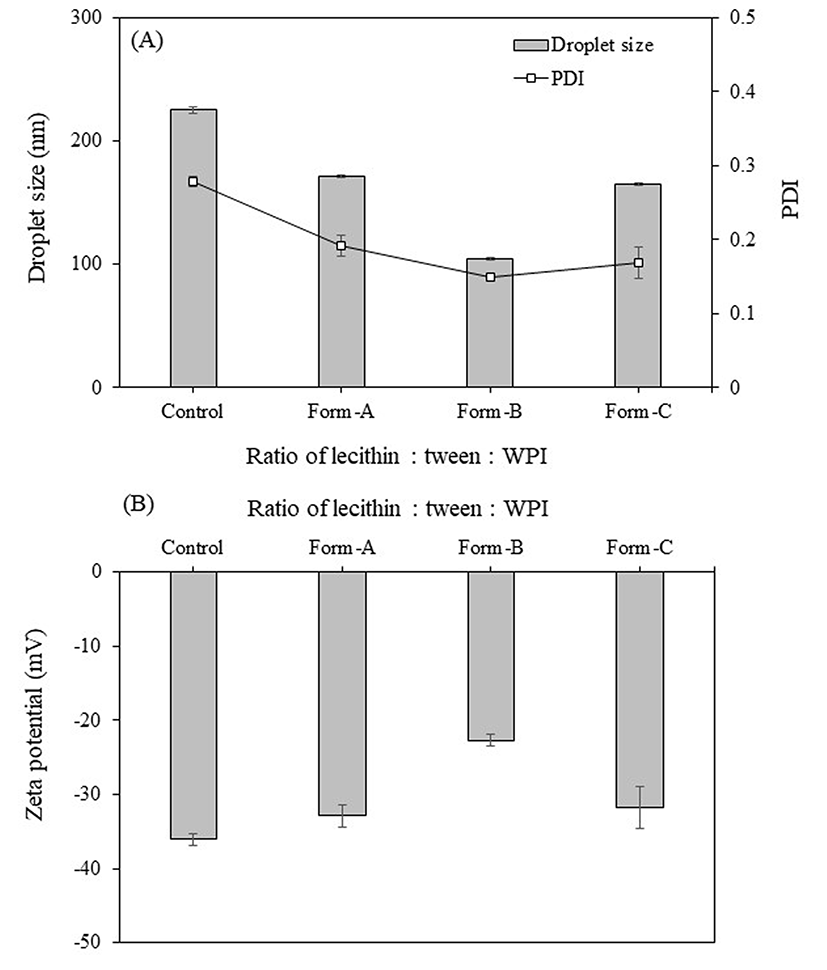
The pH of foods and in the various sections of the gastrointestinal tract varies from highly acidic to alkaline; thus, the stability of the TE-NEs (Form-C) in various pH was evaluated and compared to that of the control formulation (Fig. 4). The size and PDI of the control TE-NE remained stable (average of 220 nm) regardless of the pH, indicating that no flocculation or agglomeration occurred in any pH conditions, demonstrating their stability. This stability was also reflected in their high zeta potential, which increased from −43.81 mV in pH 2.0 to −59.90 in pH 8.0. The increase in zeta potential in alkaline conditions may be due to the adsorption of anionic species to the surface of the droplets and agrees with previous reports (Choi et al., 2014; Ozturk et al., 2015b). Furthermore, the stability of this nanoemulsion in a wide range of pH may be due to the action of Tween 20 as this emulsifier is non-ionic and provides steric stabilization that is resistant to pH changes (Teo et al., 2016).
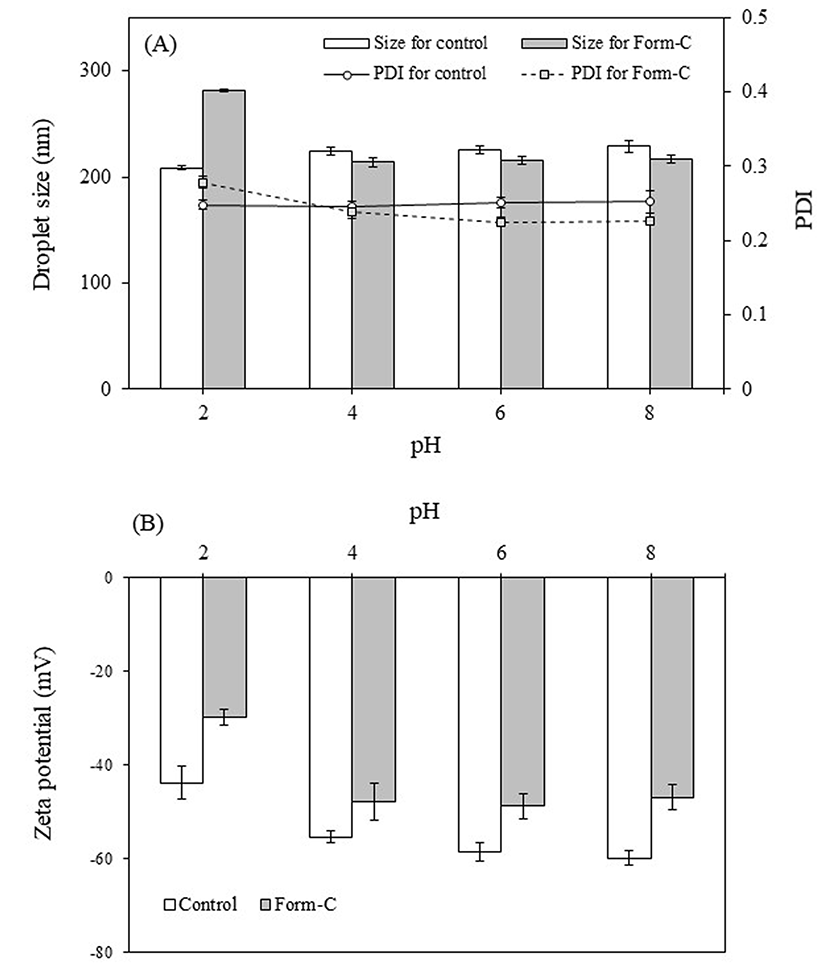
On the other hand, the TE-NEs containing WPI (Form-C) showed an increase in size (281.7 nm) under pH 2.0 conditions, followed by a sharp decrease (213.9 nm) at pH 4.0, although no further changes in size were observed at higher pH values. Likewise, the PDI was greater at pH 2.0, but decreased as the pH increased, reaching a value of 0.23 for pH above 4.0. This results contrasts with previous reports that indicated an increase in particle diameter at pH values around the isoelectric point of WPI (4.9) (Lee et al., 2011; Teo et al., 2016). In addition, the zeta potential of the TE-NEs decreased substantially at pH 2.0 (−29.75 mV) compared to higher pH values (−46.9~-48.8 mV). The lowered zeta potential observed may be explained by the loss of charge of the phospholipid groups of lecithin (Ozturk et al., 2014). Furthermore, WPI forms thin interfacial layers, stabilizing nanoemulsions by electrostatic repulsion; hence, a loss of charge results in instability and particle growth by flocculation or agglomeration (Ozturk et al., 2015a). However, the increase in size and lowered zeta potential was observed only under highly acidic conditions, and the TE-NE containing WPI was able to remain stable in a wide range of pH. This stability is likely to be due to the synergistic effect between lecithin and WPI as the phospholipid groups of lecithin can displace small peptides from the nanoparticle interface, resulting in a thin interfacial layer and smaller droplets, as well as in an increased charge, boosting the stability of the nanoemulsion (Adjonu et al., 2014).
The droplet size, polydispersity index, and zeta potential of the re-dispersed TE-NE powder (Form-C) in water were 136 nm, 0.35, and −31.8 mV, respectively. Thus, compared to the pristine TE-NE, the droplets of the re-dispersed TE-NE powder exhibited a smaller diameter and a similar zeta potential, but the PDI increased almost two-fold, suggesting a more heterogeneous size distribution. The smaller diameter observed is likely a result of shrinkage during spray-drying (Jafari et al., 2008). The TE-NE powder exhibited a light yellowish appearance and its curcuminoids content was 0.39 mg/g. By comparison, turmeric extract contained a much higher amount of curcuminoids (12.45 mg/g).
In a previous study, the biological effects of the same amount of TE-NEs and pure curcumin were very similar in vivo. Nevertheless, the TE-NEs represented a curcumin content approximately 30 times lower than pure curcumin (Ahn et al., 2017). Therefore, even though the final amount of curcuminoids in the meat would be low when incorporating the TE-NE powder. It is expected that it would still be able to provide health benefits as nanoemulsions can increase the solubility and bioaccessibility of the curcuminoids; thus a small amount of nanoencapsulated turmeric would be expected to be more effective than the same amount of turmeric powder. The sensory effect of incorporating turmeric has also to be considered, as it prevents the use of high concentrations of this spice.
Fig. 5 shows the TEM images of the TE-NE dispersion and re-dispersed TE-NE powder in water. The pristine TE-NE droplets showed the classic spherical shape and a smooth surface. On the other hand, the droplets of the redispersed TE-NE powder were not perfect spheres but exhibited many rough and irregular structures because of the dextrin molecules adsorbed onto the droplet surface. It has been reported that polymer coatings can change the morphology of nanoparticles from smooth to slightly irregular and a rough spherical shape (Li et al., 2013; Shin et al., 2013), which can explain these observations. This heterogeneous morphology may also explain the increase in PDI observed.
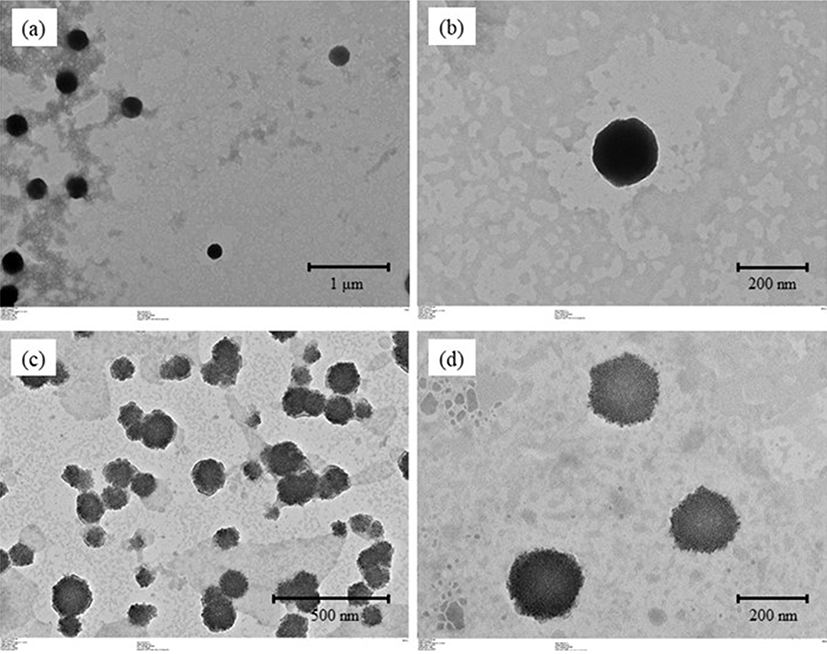
The canned ham formulations are shown in Table 1. An amount of 0.3% of TE or TE-NE powder were incorporated into the hams. Nevertheless, the content of curcuminoids in TE was more than 30 times higher, as previously described. The appearance of the canned hams incorporating TE-NE powder is shown in Fig. 6. As expected, the ham incorporating TE exhibited a more intense yellow color, whereas that incorporating TE-NE powder showed a more similar appearance to that of the control with only slight yellowing. Changes in the color of canned ham supplemented with Korean turmeric extract (TE and TE-NE powder) are shown in Table 2. The L* values of hams incorporating TE and TE-NE were slightly lower than that of the control. However, the b* value of canned ham incorporating TE was higher than those of the control and canned ham with TE-NE powder. In other words, the yellowness score increased in the formulation with turmeric extract, whereas that incorporating nanoemulsion exhibited a color more similar to that of the blank sample. In addition, the ham incorporating TE-NE powder exhibited a greater a* value, indicating increased redness compared to the control and canned ham with TE.
CH-Con, Control canned ham; CH-TE, Canned ham incorporating turmeric extract; CH-TENE, Canned ham incorporating turmeric extract nanoemulsion.
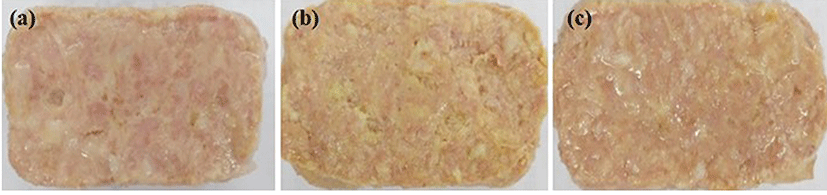
L | a | b | ΔE | |
---|---|---|---|---|
Control | 58.62±0.02c | 9.42±0.03b | 9.63±0.06a | - |
CH-TE | 55.43±0.01a | 9.27±0.04a | 13.99±0.05c | 5.4±0.04 |
CH-TENE | 55.68±0.02b | 11.77±0.02c | 11.01±0.04b | 4.0±0.01 |
*Different letters in the same column indicate a significant difference at p<0.05.
Sensory analysis of the canned hams revealed that the ham incorporating TE exhibited slightly lower sensory scores compared to the ham incorporating TE-NE powder (Table 3), although the overall acceptability of the samples was not significantly different and neither sample presented substantial off-flavor. The appearance (color) of the canned ham incorporating TE was judged to be poorer by the panelists regardless if the ham was raw or cooked. This result may be explained by the more intense yellow color of the TE compared to the nanoencapsulated form; indeed, the appearance of the ham incorporating TE was judged to be significantly different from that of the control and TE-NE ham. The nanoencapsulation results in a milder flavor and paler color; hence, the cooked canned hams incorporating nanoemulsion received the same overall acceptability score as did the control (Table 3).
Different letters in the same column indicate a significant difference at p<0.05.
Conclusion
The physicochemical characteristics of turmeric extract nanoemulsions and sensory characteristics of canned ham supplemented with turmeric extract and turmeric extract nanoemulsion were evaluated in this study. The nanoemulsions were spray-dried and incorporated into the meat to produce the fortified hams. Supplementation with the nanoemulsions successfully incorporated curcuminoids into the hams, without affecting their sensory characteristics. In particular, compared to the control, the supplemented ham exhibited only slight yellowing. Even though the amount of nanoencapsulated curcuminoids in the ham is low, the carrier increases their solubility and bioaccessibility so they could still provide health benefits. Therefore, the spray-dried turmeric extract nanoemulsion can be successfully used in the production of functional canned ham that could be accepted by the consumers.