Introduction
Extracellular microvesicles (EMVs) are membranous nanosized cellular organelles naturally released by cells in vitro and in living organisms (Yamada et al., 2012). Microvesicles have been found in a number of human body fluids, including blood plasma (Caby et al., 2005), urine (Pisitkun et al., 2004), breast milk (Admyre et al., 2007), amniotic fluid (Keller et al., 2007) and their widespread presence shows they have important functions in vivo. Being charged with functional proteins, RNA molecules and antigens, EMVs have been shown to be a novel way of cell-to-cell communication (Ekström et al., 2012; Momen-Heravi et al., 2012).
Previous work using bovine milk microvesicles has shown that they contain mRNA and miRNA that can be transferred to immune cells to potentially modulate immune cell function (Hata et al., 2010). A variety of methods to isolate EMVs have been documented each with its own advantages and disadvantages when isolation scale, purity and ease are desired (Yuana et al., 2011). For a particular application of microvesicles such as for RNA analyses, proteomics, and biological assays choice of method is particularly important (Peterson et al., 2015). For different biofluids, different methods have been developed and validated yielding different levels of vesicular RNA, related proteins and number of vesicles (Yamada et al., 2012).
Selection of microvesicle isolation method is important in order to ascertain reproducibility of studies involving microvesicle analysis or functionality. In this work, differential ultracentrifugation as a conventional method, ultracentrifugation with some modification such as additional precipitations, ultrafiltration, and ExoQuickTM as a commercial reagent were used. The goal was to evaluate six possible methods of microvesicle isolation and compare the yield and purity microvesicular microRNA.
Materials and Methods
Bovine colostrum samples were collected on the 1st and 2nd d of lactating healthy Holstein cows (Korea). Milk samples were frozen immediately after milking and were kept at −80°C until use. Whey for all experiments was prepared by centrifuging colostrum at 1,500 g for 10 min at 4°C and then at 16,500 g for 1 h at 4°C. The resulting supernatant was then ultracentrifuged at 50,000 g for 1 h. In this work, differential ultracentrifugation as a conventional method, modified ultracentrifugation method including additional precipitations and commercial reagent (ExoQuick™) were adopted to compare the yield of total RNA, their purity and microvesicle internalization. Methods used were classified into four general categories: Conventional Methods (1 and 2), Exclusion Methods (Lactic acid and EDTA method), ExoQuick™ methods and OptiPrep™ sucrose gradient separation.
1) Conventional method 1 To pellet microvesicles, 10 mL of samples were ultracentrifuged wit 100,000 g speed for 90 min at 4°C. The obtained pellet was resuspended in PBS and ultracentrifuged at the same condition again twice to wash it.
2) Conventional method 2 For this method, 10 mL of samples were ultracentrifuged at 100,000 g for 1 h. After discarding pellet, the supernatant was further ultracentrifuged at 135,000 g to pellet microvesicles and then resuspended in PBS and washed at the same conditions twice.
3) Lactic acid exclusion method pH of colostrum samples was adjusted to 4.6 using lactic acid and incubated on ice for 15 min to allow for maximum protein precipitation. Mixture was ultracentrifuged at 100,000 g for 1 h, and the supernatant retained and subjected to a further separation using ultracentrifugation at 135,000 g for 1 h. The resulting pellet was resuspended in PBS and ultra-centrifuged at the same speed twice to wash the pellet. The pellet was then resuspended in 200 mL of PBS and stored at −80°C for further analysis. Acidification step did not cause negative effect on microvesicle quality (Hata et al., 2010).
4) EDTA exclusion method Ten milliliters of EDTA were added to 10 mL of samples and incubated for 15 min on ice. The mixture was then separated by ultracentrifugation with 100,000 g for 1 h at 4°C, and the supernatant retained and subjected to a further ultracentrifugation stage at 135,000 g for 1 h at 4°C. The resulting pellet was resuspended in cold PBS and ultracentrifuged at the same speed twice to wash the pellet. The pellet was then resuspended in of PBS and stored at −80°C for further analysis.
5) ExoQuick™ method Ten milliliters of samples were mixed with 2 mL of ExoQuick™ solution and stored at 4°C overnight. Solution was then centrifuged at 1,500 g for 30 min at room temperature and the supernatant was removed. The pellet was further centrifuged for 5 min at 1,500 g to remove traces of fluid. The pellet was then resuspended in 10 mL of PBS and ultracentrifuged at 135,000 g for 90 min.
6) OptiPrep™ method Colostrum samples were separated by ultracentrifugation for 1 h at 135,000 g to isolate a crude microvesicles. The pellet was resuspended in 500 mL of PBS and this crude sample was then laid on top of a sucrose gradient solution and centrifuged for 16 h. The gradient was generated by diluting a stock solution of OptiPrep™. The gradient was formed by adding 3 mL of 40% iodixanol solution, followed by careful layering of 3 mL each of 20% and 10% solutions, and 2.5 mL of the 5% solution (Tauro et al., 2012). After centrifugation, 12 individual 1 mL gradient fractions are collected manually (with increasing density by top-bottom collection). Fraction 7 (corresponding to the density 1.11 g/mL) which has been proven (Lobb et al., 2015) to be the richest in micro-vesicles when this method is employed was collected into a separate microcentrifuge tube. And then, it was resuspended in PBS and washed two times at 135,000 g for 1 h to give the final microvesicle pellet.
Total RNA extraction was done using miRNeasy Serum/Plasma Kit (Qiagen) following the manufacturer’s protocol with minor modifications. RNA yield and purity were verified by measuring absorbance (A260/280) on a Nanodrop spectrophotometer (Optizen-NanoQ, Mecasys Co. Ltd., Korea). Protein levels in samples were determined with the Bradford assay (Bio-Rad). The standard curves for the assay was obtained using bovine serum albumin.
RNA integrity and miRNA content for each sample was determined using a Bioanalyser using Small RNA Kits (Agilent). Electropherograms and miRNA concentration data from the Bioanalyser were used to compare the different methods. The one microliter of RNA was analyzed an Agilent 2100 Bioanalyzer using an RNA 6000 nano Kit (Agilent Technologies, USA), according to the manufacturer’s protocol.
Total RNA extracted from microvesicle isolates from the different methods was reverse transcribed to make cDNA using miScript II RT kit following the manufacturer’s instructions (Qiagen). qPCR was performed using a StepOne Plus Real Time PCR System (Applied Biosystems, USA) with miScript SYBR Green PCR Kit. Threshold cycle (CT value) is a relative measure of the concentration of an individual miRNA in the PCR reaction and lower CT value indicates higher expression. qPCR values for four detectable microvesicular miRNAs were normalized to Cel_miR_39 (a spike in control miRNA) and the delta Ct values were graphed for microvesicles from the different methods.
Western blot was carried out as previously described (Cvjetkovic et al., 2014). Briefly, samples were homogenized using radio immunoprecipitation assay (RIPA) containing protease inhibitor (Cell Biolabs, USA). Volumes containing equal amounts of proteins were mixed with reducing Laemmli-buffer. The proteins were the separated on 4-20% Tris-glyceride SDS polyacrylamide gels (Bio-Rad). Proteins were blotted onto a polyvinylidene difluoride membrane (Pall Life Sciences). The membrane was blocked in 5% non-fat milk followed by incubation in primary antibody (anti-CD9 [1:500; Invitrogen] for 1 h at room temperature. Blots were washed 3 times in TBS-T (TBS buffer containing 0.05% Tween 20; 15 min per each wash) and then incubated HRP-conjugated antibody for 1 h at room temperature. The blots were washed again 3 times in TBS-T and signals were visualized using the Amersham™ enhanced chemiluminescence kit (GE Healthcare Companies) by an imaging instrument (C-DiGit® Blot Scanner, Li-COR).
Microvesicles were stained using PKH67 Green Fluorescent Cell linker Kit for cell membrane labeling (Sigma Aldrich, USA). Labeled microvesicles were incubated with RAW264.7 cells at 37°C. Cellular uptake of labeled microvesicles was determined using fluorescent microscopy. Cells were seeded at a rate of 104 cells/cm2 in 24 well plates and treated with a portion of microvesicles equating to 20 mg of protein.
Data were expressed as mean±standard deviation. One way ANOVA was carried out to compare miRNA yields and RNA:Protein ratios for the selected methods. Turkey’s multiple comparison test was the used to identify which exact methods differed from each other. The differences were considered statistically significant at p<0.05.
Results
All methodology in this study are summarized at Fig. 1. Total RNA determined using a nanodrop. The results are shown in Table 1. This then analysed for miRNA using a Bioanaliser and Small RNA chips. All methods had a generally similar pattern for small RNA (Fig. 2A). Conventional methods had the highest small RNA yields and between the two conventional methods, conventional method 2 had a slightly lower percentage of small RNA (data not shown) likely because the initial pellet from 100,000 g centrifugation contained a significant amount of microvesicules. Centrifugation proved to be an effective method to pellet microvesicles.
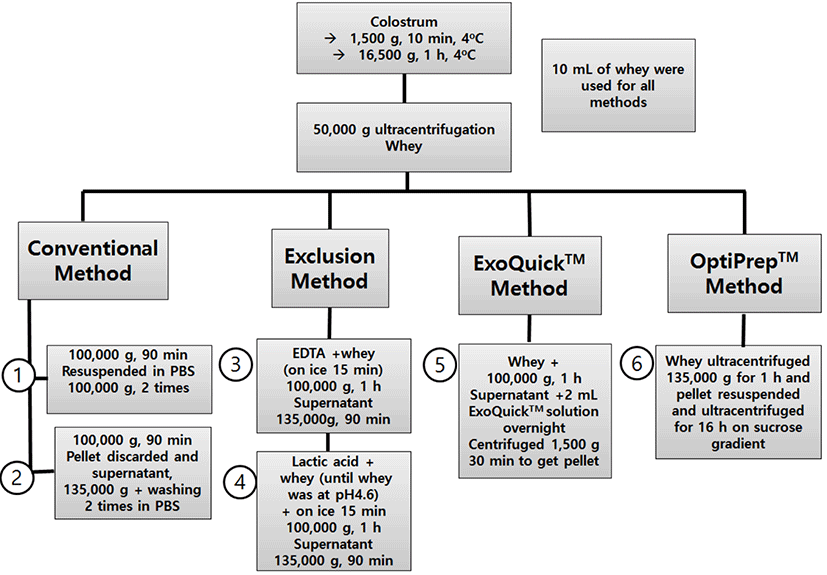
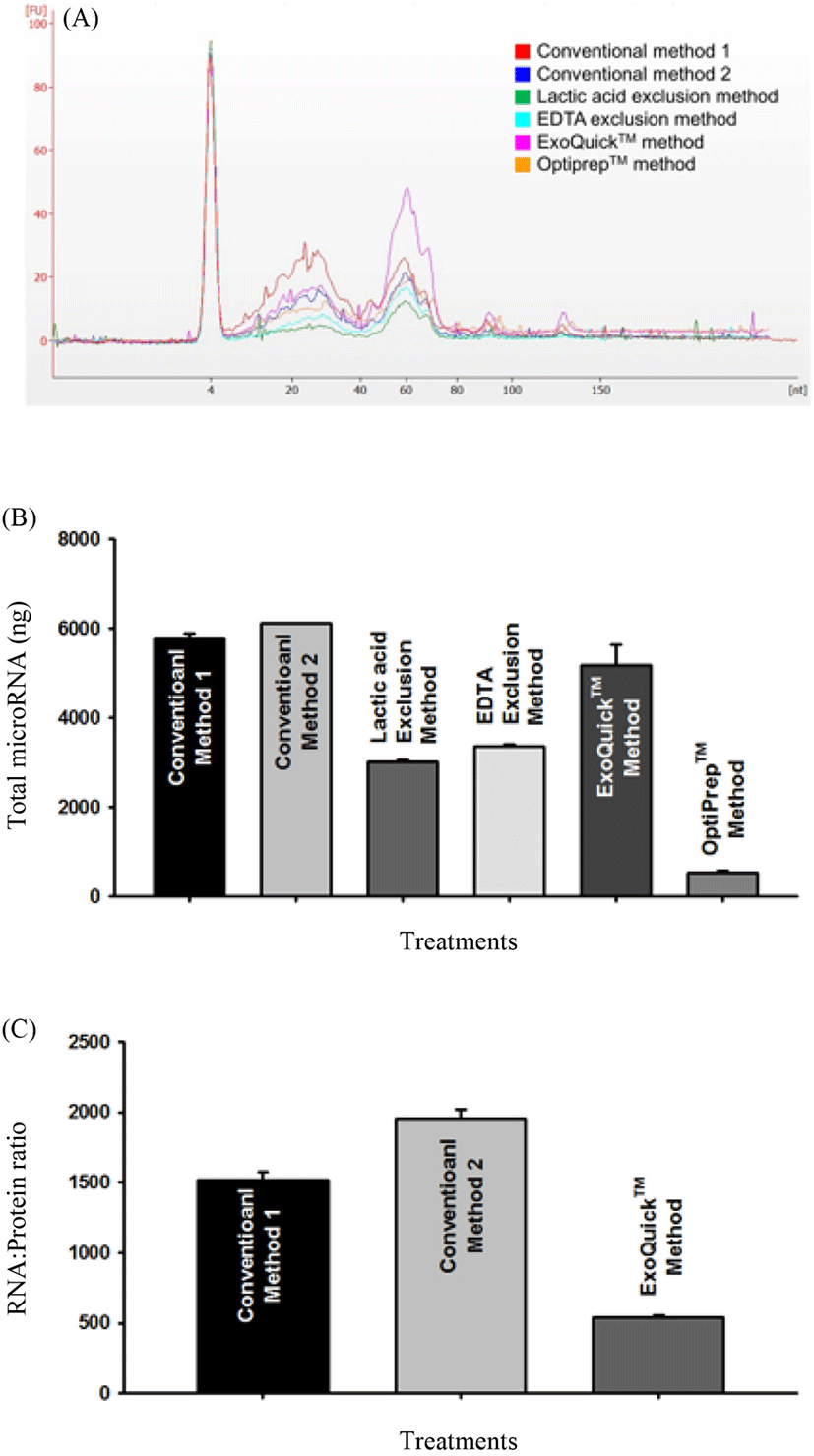
Bioanalyser results to asses levels of small RNA showed that the different method categories yielded differing levels of small RNA. Conventional methods combined had higher miRNA concentrations in total RNA per sample (avg = 71%) compared to other methods combined (avg = 55%). This shows that ultracentrifugation has a greater yielding effect on miRNAs in colostrum microvesicles when compared to chemical based precipitation methods. The results of this experiment affirm that the method used to isolate microvesicles from bovine colostrum will give a unique distribution of small RNA in the extracted vesicles.
The microvesicular miRNA yields differed substantially between different microvesicle isolation method categories. As shown in Fig. 2B, for conventional ultracentrifugation methods the microvesicular RNA yields were highest at 5,772 ng (Conventional Method 1) and 6,110 ng (Conventional Method 2). Ultracentrifugation with modification by addition of lactic acid to precipitate protein yielded 3,028 ng while use of EDTA yielded 3,358 ng. Conventional methods and exclusion methods had no significant differences in yield for both methods within each group (p>0.05). ExoQuickTM method had a yield of 5,178 ng and was the third highest yielding method category but significantly lower in yield than Conventional method 2 yet not significantly different from conventional method 1. Optiprep™ method yielded 541 ng a distant lowest among all methods.
To separate between the three highest yielding methods, RNA:Protein ratio was used and it is a measure of how much protein is precipitated in relation to miRNA. RNA: Protein ratio is an important factor for in vivo and in vitro applications of microvesicles to assess microvesicle functionalities without risk of high contaminating non-vesicular biomaterial in the form of co-precipitating proteins during isolation. Conventional method 2 (Fig. 2C), had a significantly higher RNA:Protein ratio compared to the other methods and was thus the selected method for this work.
There were no substantial differences between protein levels for both conventional methods (Table 1). Exclusion methods were also not significantly different but were both substantially lower than conventional and ExoQuick™ methods. Pellet from 135,000 g method intended for OptiPrep™ method had highest protein level followed by ExoQuick™ method and both were significantly different from each other. OptiPrep™ derived microvesicles had the lowest level of protein compared to microvesicles from all other methods. The low protein level in OptiPrep™ method pellet is an indication of the ability of the method to isolate microvesicles into a particular layer with minimum non-exosome materials. The protein measured was not specifically microvesicular protein and thus was only a rough indicator of possible microvesicle amount in the sample.
After analysis of small RNA using the Bioanalyser, presence of miRNA in the extracted RNA was confirmed using qPCR. Different miRNAs isolated with same trend within each method across all methods (Fig. 3). miR-200c has lowest delta CTs and seems to be abundant in microvesicle fraction of colostrum while miR-155 had a consistently lower CT value indicating its lower presence in microvesicles isolated using the different methods. Besides miR-200c, other miRNAs have a trend of expression representative of their count read data where a high count read data in colostrum (data not shown) resulted in a lower CT value for qPCR.
To determine presence of vesicles in the isolates and assess purity of isolated vesicles, samples were analysed using western blot for presence of CD9 a protein previously found in extracellular vesicles (Mathivanan and Simpson, 2009). Equal amounts of protein were added for each sample. All samples were successfully positive for CD9 proving that isolates did contain microvesicles (Fig. 4). Visible band of CD9 was barely detected in OptiPrep™ method and this result indicates that it had low yield of microvesicles as also supported by its low micoRNA yield when compared to other methods.

For SEM imaging, microvesicles were fixed in 2% paraformaldehyde for 15 min at room temperature. Drops of fixed samples were placed on thoroughly silicon chips and air dried before imaging. Fig. 5 shows a SEM image of microvesicles isolated using conventional method 2. RAW 264.7 cells showed successful uptake of PKH labeled microvesicles as examined using fluorescent microscopy (Fig. 5).
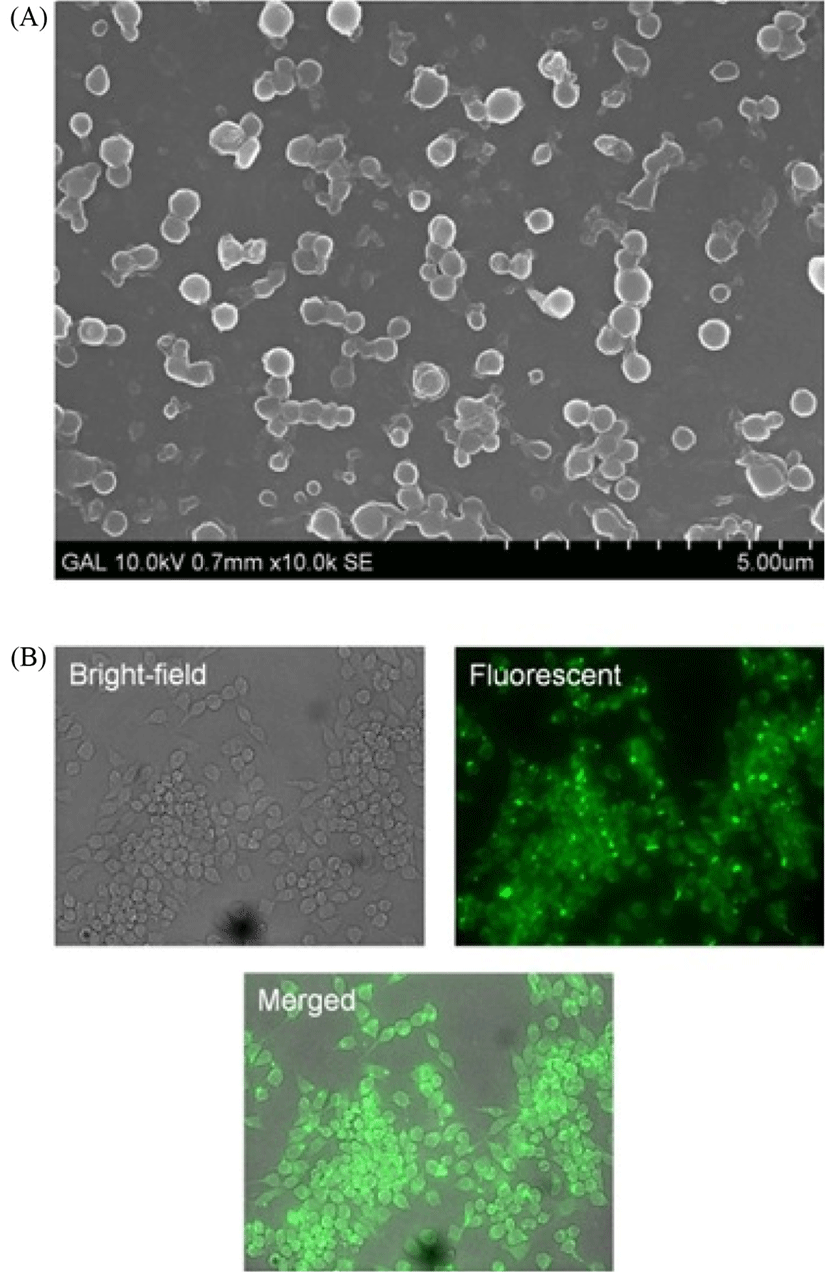
Discussion
This study evaluated six different methods of exosome isolation to compare their efficiency in yielding microvesicles and microvesicular biomolecules specifically micro-RNA. We focused this study on the miRNA yield, protein yield, and analysis of small RNA including miRNA abundance and distribution. Importantly microvesicular miRNA yield differed substantially between the different isolation methods. There are many documented methods for isolating microvesicles from different biological fluids but none has been done specifically for bovine colostrum. Efficiency of each method will vary with type of biofluid and as such methods employed should be optimized for a specific biofluid (Momen-Heravi et al., 2012). The results presented here indicate that the microvesicle isolation method based on serial centrifugation (first at 100,000 g then at 135,000 g) had the highest levels of miRNA in the total miRNA levels. Ultracentrifugation has been proved an efficient way for isolating microvesicles (Dimov et al., 2009) albeit with further purification of such vesicles by methods such as sucrose gradient ultracentrifugation (Xiao et al., 2009).
Total protein level in microvesicle isolate was highest in the pellet obtained from ExoQuick™ method. This result shows that the used chemical for precipitating microvesicles is not specific to microvesicles only but will efficiently precipitate vesicles and protein-protein, lipoprotein or protein-RNA complexes present in the sample regardless of their origin (Rekker et al., 2014). This unspecific precipitation may in downwards experiments cause a confounding effect if microvesicles from colostrum need to be characterized as the resulting pellet will be highly heterozygous in terms of biomolecule content (Rekker et al., 2014). The result of this high protein yield in ExoQuick™ derived microvesicle was a corresponding low RNA:Protein ratio for the method. The high RNA:Protein ratio for conventional method 2 compared to conventional method 1 is likely a result of the high centrifuging speed with 100,000 g used to remove excess protein prior to collecting microvesicles in the subsequent step. A lower speed of only 50,000 g was used for conventional method 1 and would have had less precipitating effect on contaminating proteins. Such effects on yielded microvesicle characteristics by centrifugal variations have been documented by (Cvjetkovic et al., 2014). OptiPrep™ method yielded the lowest protein yield indicating a more refined set of microvesicles as the gradient formed separates different layers and the specific layer enriched in microvesicles is taken out. It is worth noting that when the ultimate goal is microvesicular RNA profiling or proteomic studies using western blot or ELISA, the abundant protein levels in ExoQuick™ method will not pose a problem (Alvarez et al., 2012).
Overall microvesicular miRNA distributions were similar regardless of method showing that microvesicular cargo is somewhat similar between different methods. The miRNA levels were however different between methods. In short conventional methods proved to be more efficient at isolating high microvesicular miRNA. Different processing methods for obtaining microvesicles have been shown before to yield different amounts of microvesicular cargo (Yamada et al., 2012) and this result is in line with such existing information. The qPCR results showed possibility of some miRNAs having a uniform expression in colostrum and microvesicles when count data and delta CT values are compared. This is the case with miRNAs such as miR-21-5p, miR-93 and miR-155 whose count data values in colostrum follow a similar trend to their respective delta CT values with qPCR. In the case of miRNA miR-200c expressions however do not show a typical trend between colostrum count read data and delta CT data from qPCR showing that there could be different amounts of such miRNAs in microvesicles and whole colostrum. As such, different miRNAs could be following in unique patterns between different components of colostrum.
In conclusion, we have demonstrated the uniqueness in characteristics of microvesicles isolated using 6 different methods. The methods were evaluated for microvesicular miRNA yield, distribution and purity. Each method had unique characteristics for these even though the same sample was used as the initial source for the microvesicles. As each method gave a unique set of characteristics for microvesicles, it is imperative that informed choice of method be made during studies aiming to evaluate microvesicle functionality studies as functionality will be a result of specific set of cargo in the microvesicles. For purposes of high microvesicular yield targeting, our method of choice is Conventional Method 2 because it yielded the most relatively pure and highest amount of microvesicular miRNA. Successful uptake by cells shows potential of microvesicles to deliver their cargo (miRNAs) in cells in vitro and in vivo thus they can be used in functional studies.