Introduction
The rapid distribution of beef products and consumer demand have increased the global consumption of red meat obtained from beef (González et al., 2020; Petrovic et al., 2015). In recent decades, the average consumption of Hanwoo beef has increased from approximately 6.7 to 11.5 kg per person in Korea. This increase might be attributed to the presence of favorable meat quality characteristics such as high n-6 polyunsaturated fatty acid levels, high marbling, and high organoleptic properties (Chung et al., 2018). Producing high-quality and safe meat is important top priorities of the global food industry because consumer acceptance and demand mainly depend on these attributes. Currently, various technological approaches that can preserve nutritional properties, such as high pressure, electrical pulses, and ultrasonication, are being employed to manufacture qualitative food products (Alarcon-Rojo et al., 2018).
High intensity-ultrasound (HIU) is a non-thermal sustainable technological approach that can be implemented in the food industry while exerting positive impacts on food processing, the maintenance of texture, and food quality (Chavan et al., 2022). The HIU is also nonionizing, noninvasive, and eco-friendly mechanical energy (Chemat et al., 2011) that enhances the phenomenon of acoustic cavitation (Hoover, 2000). The release of energy together with mechanical shock affects cell architecture in the microenvironment. The characteristics of ultrasound system can be distinguished by ultrasonic intensity or frequency (high-intensity: >1 W/cm2; low-frequency: 20–100 kHz). High-intensity, low-frequency, ultrasound waves can create physical, chemical, and mechanical impacts, which cause structural and physiochemical alterations while inducing chemical reactions (Alarcon-Rojo et al., 2015). Due to the presence of these favorable conditions, ultrasound treatment can preserve or develop the functional properties of foods without causing damage or creating undesirable conditions (Caraveo-Suarez et al., 2022). Confirming that, Terefe et al. (2016) reported that the application of ultrasound resulted in enhanced chemical, physical, and functional properties in the food while modifying them.
Because of the progressive technological development in the food sector, the application of HIU has gained special attention in meat tenderization, crystallization, drying, freezing, emulsification, homogenization, and the inactivation of microbes and enzymes (Kentish and Feng, 2014). The implementation of the ultrasound technique in meat is mostly conducted on bovine longissimus, and semitendinosus (ST) muscles and their meat quality traits (Amiri et al., 2018; Caraveo et al., 2014; Chang et al., 2015; Chemat et al., 2011; Peña-González et al., 2017; Xiong et al., 2012). However, some studies are being conducted on the quality characteristics of pork (Ojha et al., 2016), chicken (Li et al., 2015), fish (Fan et al., 2017), and rabbit meat (Gómez-Salazar et al., 2018). Improved tenderness in meat has a significant impact on determining the commercial value of the final meat product (Morgan et al., 1991; Stadnik and Dolatowski, 2011). Caraveo et al. (2014) and Dolatowski et al. (2000) reported that the sonication of beef ST muscle for 60 and 90 min at 40 kHz and 11 W/cm2 and the sonication of beef semimembranosus muscle at 25 kHz and 2 W/cm2 positively affected meat tenderness and water-holding capacity (WHC), respectively. Amiri et al. (2018) found that ultrasound of beef longissimus dorsi muscle resulted in higher pH, and increased elasticity and WHC. Conversely, some experiments reported no changes in meat pH, WHC, and other quality traits from the ultrasound treatment of beef semimembranosus muscle (Stadnik and Dolatowski, 2011). Other than physio-chemical characteristics, meat sensory attributes are also considered important quality parameters in meat processing since they determine consumer meat preferences. Accordingly, the application of 40 kHz and 11 W/cm2 sonication treatment to beef longissimus dorsi muscle resulted in more tenderness and juiciness, which led to developed overall sensory attributes (Caraveo et al., 2014). However, only limited scientific approaches have been aimed at clarifying the mechanism of HIU on the sensory attributes of beef during postmortem cold storage. Most of the studies demonstrated that the ultrasound treatment of meat successfully tenderized and reduced the aging time of meat without negatively affecting any qualitative traits (Wang et al., 2018; Yeung and Huang, 2017; Zhang et al., 2019).
Therefore, ultrasound application could be an efficient alternative cost-effective method to enhance meat quality characteristics including the sensory attributes. Hence, the purpose of this study was to elucidate HIU treatments as a novel technological approach to enhance meat quality properties, sensory evaluation, fatty acid composition, and microstructural changes in beef ST muscle.
Materials and Methods
ST muscle samples from Korean native cattle (Hanwoo, 29 months old, 460–501 kg hot carcass weight) were obtained from the Hadong Livestock Industry Cooperatives, Hadong-gun, Korea. All fattening steers used in the experiment were raised on the same farm with the same feed and specification methods. At 24 h of post mortem, all visible bone, fat, and connective tissues surrounding each muscle, were carefully removed without damaging the muscle. Six samples were cut into 12×8×8.5 cm (length×width×height, respectively) slices in size and 200±5 g in weight. The prepared samples were vaccum-packaged using polyvinyl chloride bags (Koch easy-pack 2001, Koch Supplies, Kansas City, MO, USA) and randomly divided into two treatments that designated as control (CON) and HIU.
According to the storage length (0, 3, or 7 days at 1°C), samples were further identified as; C0, C3, and C7, and HIU0, HIU3, and HIU7, respectively. The vacuum-packaged muscle samples were treated with HIU at powers of either 0 or 800 W/cm2 and a frequency of 35 kHz. The samples were treated for 60 min in a modified high-intensity ultrasonic bath (Branson® 1510 model 1510R-MTH, Branson Ultrasonics, Danbury, CT, USA) using distilled water as the diffusion medium. The height and width of the ultrasonic bath was 56 and 36 cm, respectively. The bath temperature was approximately 20°C and it was maintained at 1°C using cold water during HIU treatment. The established power of the HIU system was determined using a calorimetric technique previously described by Margulis and Margulis (2003). Then, the CON and treated samples were aged at 1°C and analyzed at each aging period.
Meat color was evaluated in triplicate using a colorimeter (Konica Minolta CR-300, Konica Minolta, Osaka, Japan) that was standardized with a white plate (Y=93.5, X=0.3132, y=0.3198) before measuring CIE L*, CIE a*, and CIE b* at three different areas of the meat samples. The pH of the meat was evaluated using a digital pH meter (MP 230, Mettler-Toledo, Schwerzenbach, Switzerland). Approximately 3±0.5 g of meat sample was mixed with 27 mL of deionized water and then homogenized (IKA T25 ULTRA-TURAX, IKA-Werke, Staufen, Germany) for 30 sec. The probe was previously calibrated at 25°C with calibration solutions at pH values of 7.00, 4.01, and 9.21.
Drip loss (%) was evaluated in meat samples in triplicate according to the procedure of (Joo et al., 2002). The samples for driploss weighed approximately 25±0.5 g (2 cm in diameter, 2 cm thick) and were suspended on a string and an unfolded paperclip (hook shape) within a plastic box (18×15×10 cm). Thereafter, the samples were placed in cold conditions at 4°C for 24 h, and DL was expressed as a percentage of the original weight of the muscle as follows:
The cooking loss (in triplicate) of the meat samples was determined as the percentage of the weight loss and was evaluated by placing a 1.5-cm-thick muscle sample of about 25±0.5 g in a polythene zipper bag, heating it in a water bath at 75°C for 30 min, cooling it to room temperature, and holding for 30 min. Following cooking loss determination, the shear force value of the meat samples was (WBSF, kg/cm2) evaluated in triplicate using an Instron Universal Testing Machine (Model 3343, Instron, Norwood, MA, USA) with a V-shaped shear blade. A cross-sectional area as close as practicable in size to 0.5 cm×4.0 cm (approximately 2.0 cm2) was cut across the fibers in each sample to measure the cutting forces. The crosshead speed was set at 100 mm/min. The maximum scale load was 50 kg.
Releasing water content (RW%) was measured based on the method of Joo (2018). Approximately 3±0.5 g of meat sample was placed on weighed filter paper (Whatman No. 1, 11 cm in diameter) covered with two thin plastic films. The filter paper and plastic film consist of the meat samples were placed between Plexiglas plates, upon which a constant 2.5 kg pressure was applied for 5 min. Then, the percentage of RW was calculated as follows:
Intramuscular fat (IMF) content was determined using a modification of the method of Folch et al. (1957). Briefly, lipid was extracted from a 3±0.5 g of homogenized meat sample treated with 30 mL of Folch solution (chloroform: methanol=2: 1, v/v). The homogenate was filtered (Whatman no.1 filter paper) and the filtrate was treated with 8 mL of NaCl (0.88%) and vortexed. Then, after 12 hours, the top layer was removed and the remaining bottom layer was transferred to a dish to dry at 50°C. Finally, the IMF content was measured using the weight differences of both empty and dried dishes.
Briefly, 5±0.5 g of beef meat sample was homogenized using 15 mL of distilled water and 50 μL of butylated hydroxytoluene (BHT) solution. Then, 1 mL of the supernatant and 2 mL of TBA/TCA solution were added to a glass tube. The mixture was incubated at 90°C for 15 min in a shaking water bath followed by cooling at room temperature for 10 min. The sample was centrifuged at 1,000×g for 10 min, and the absorbance was evaluated at 531 nm using a UV-Vis spectrophotometer (G1115AA, Agilent Technologies, Santa Clara, CA, USA) against a blank containing 2 mL of TBA/TCA/HCl mixing solution in 20 mL of distilled water and 50 μL of BHT solution without muscle sample. The thiobarbituric acid-reactive substance (TBARS) values were expressed as miligrams of malonaldehyde/kg of meat.
MFI values were determined according to the method as suggested by Hopkins et al. (2000) and Nakyinsige et al. (2014). Briefly, 2±0.5 g of meat samples were mixed in 40 mL of ice-cold buffer solution (0.1 M potassium chloride, 0.02 M potassium phosphate, 0.001 M magnesium chloride, 0.001 M sodium azide, and 0.001 M ethylenediaminetetraacetic acid disodium salt) and homogenized at 11,000×g for 30 s. Then, the homogenate was centrifuged at 1,000×g for 15 min and the supernate was discarded. The residue was suspended in 40 mL of the buffer and the homogenate was centrifuged again according to the previous conditions. Thereafter, the resulted residue was suspended in 10 mL of the buffer solution and homogenized at 11,000×g for 30 s.
The homogenate was filtered through a polythene screen (18 mesh) and the tube was washed using 10 mL of buffer solution to eliminate attached connective tissues. The protein concentration was evaluated using the biuret method. After the dilution of the myofibril suspension with potassium phosphate buffer, the absorbance value of the suspension was evaluated at 540 nm with a UV-Vis spectroscopy (Agilent 8453, Agilent Technologies). Finally, the MFI was expressed taking averaged three absorbance reading and multiplying them by 150.
The extraction of lipid from muscle samples was carried out according to method described by Folch et al. (1957). The lipid methyl esters were evaluated by treating with 1.0 N methanolic NaOH and methylated by boron trifluoride in methanol solution. Fatty acid methyl ester (FAME) determination was conducted by gas chromatography HP6890N (Hewlett-Packard, Santa Clara, CA, USA) using an automatic sampler HP7683 (Hewlett-Packard). The separation of FAME from the samples was conducted under the following conditions: Column oven temperature was increased from 50°C to 180°C at 10°C per min and maintained at 180°C for 20 min, the temperature of both the injector and detector was 250°C, and the sample injection volume was 1 μL. Finally, resulted each fatty acids content was recognized by comparing the retention times to those of standards FAME mixture (Supelco 37 Components FAME Mix, Sigma-Aldrich, St. Louis, MO, USA). The results are expressed as the percentage of total fatty acids detected based on the total peak area.
Electronic tongue analysis of the beef muscle samples was conducted using an electronic tongue system (ETS; INSENT SA402B Electric Sensing System, Insent, Tokyo, Japan) according to the method explained by Ismail et al. (2020). The ETS system mainly consisted of sensor arrays, electrodes, a data analysis software, and specific artificial lipid membranes. The ETS can distinguish five different flavors according to the flavor identification traits. The analysis of each sampled parameter was carried out after all membranes were stabilized in a standard meat taste (SMT) solution. The SMT solution was comprised of 0.01% lactic acid (sourness), 0.25% monosodium glutamate (umami), 0.0005% quinine hydrochloride (bitterness), 0.55% sodium chloride (saltiness), and 0.8% sucrose (sweetness). Briefly, 100±0.5 g of minced meat sample was mixed with 400 mL of hot water (95°C; 20 min). The mixed solution was centrifuged for 15 min at 1,000×g, and the supernate was stored at –70°C for further analysis.
Sensory assessment was conducted according to the modified Spectrum TM method for descriptive analysis by eight trained judges (4 male: 4 female members) with more than 50 hours of experience in beef evaluation (Meilgaard et al., 1999). The panelists evaluated the cooked meat samples for flavor, juiciness, tenderness, umami, and overall using a 9-point hedonic scale (from 1 point, extremely dislike to 9 points, extremely like). Marbling, color, texture, surface retention water, and overall of fresh meat were evaluated on a 5-point hedonic scale (from 1 point, extremely bad to 5 points, extremely good). The approved consent procedure for sensory evaluation is Institutional Review Board (IRB): GIRB-G21-Y-0059. The signed consent from all the panelists was taken before the sensory evaluation.
The effects of HIU treatment on meat microstructure were investigated using TEM. Slices of sonicated and non-sonicated samples from ST muscle were fixed in 2.5% glutaraldehyde and 1% osmium tetroxide for 72 h at 4°C. The slices were then dehydrated in an ethanol series (50% to 100%) for 20 min. After polymerization in epoxy resin, 30-nm-thick sections were collected and stained with 3% uranyl acetate and lead citrate. Representative micrographs of the samples were taken with a Talos L120C transmission electron microscope (120 kV, Talos L120C, FEI, Hillsboro, OR, USA).
Statistical analysis of the obtained data was analyzed using two-way anova of variance (factorial ANOVA) using SPSS version 23 (IBM, Armonk, NY, USA). The comparison of treatment means was evaluated using Tukey’s post hoc test. A p-value of <0.05 was considered significant. The illustration of the hierarchical cluster tree was generated according to the relative composition of individual fatty acids using the Complex Heatmap package within R software version 4.0.3 (R Core Team, 2020).
Results and Discussion
The surface appearance of meat, especially color, has a significant impact on both consumers and producers. Consumers’ purchasing decisions are mainly determined by fresh beef color (cherry-red color; Alarcon-Rojo et al., 2019). The meat quality characteristics due to ultrasound treatment are presented in Table 1. The results revealed that sonication for 60 min had no significant effect on the CIE L* and CIE a* of beef ST samples (p>0.05) over the storage period. Confirming that, Alves et al. (2018) found that ultrasound applied (45 kHz) at different times (0, 60, 120, or 240 s) did not result in color changes during the cold storage period. Previous studies also reported that HIU treatment had no impact on beef meat color, which was due to the lower oxidization of color pigments and lower heat generation (Chang et al., 2015; Jayasooriya et al., 2007; Sikes et al., 2014). They speculated that the heat produced by the ultrasound technique was insufficient to denature proteins and color pigments in meat (myoglobin and metmyoglobin). In contrast, the cold environment created by the water bath might maintain constant lower temperatures during HIU treatment (Caraveo-Suarez et al., 2022; Chang et al., 2012). In the present study, the CIE b* was significantly increased on days 0 and 3 in HIU-treated ST muscle samples. Chang et al. (2012) reported that ultrasound treatment caused lower CIE b* in beef ST muscle. This discrepancy is probably associated with the different frequency and intensity levels (1,500 W and 40 kHz) compared to the present study (800 W and 35 kHz), as well as meat steak size differences. The contradictory results might also be due to the type of muscle, the animal age, aging time, and ultrasound treatment parameters such as frequency, intensity, and duration.
A,B Different letters within a row of aging time indicate statistically significant differences at p<0.05.
The pH of meat greatly influences the qualitative and functional characteristics of muscle proteins, and higher pH values increase the WHC of meat due to alterations in the electrical charges within muscle protein (Alarcon-Rojo et al., 2019). The pH of neither muscle sample changed significantly due to sonication over the storage period (p>0.05). The above results were consistent with the findings of Caraveo-Suarez et al. (2022) who reported unvarying pH values in bovine longissimus dorsi and triceps brachii muscles that were treated with HIU (45 kHz frequency and 11 W/cm2 intensity). However, the pH in the current study was within the optimum beef muscle pH range of 5.4–5.6 (Węglarz, 2010). The TBARS value was increased in both CON and HIU-treated ST muscles during the storage period. In particular, the aging period caused elevated TBARS levels on days 0, 3, and 7, whereas HIU treatment elevated TBARS on the third day of the storage period (p<0.05). The higher TBARS in the sonicated treatment might be attributed to higher meat oxidation by free radical reactions, which are generated through myofibrillar fragmentation, protein oxidation, and the destruction of protein structure (Kuijpers et al., 2002; Vestergaard et al., 2000). The aging period also contributed to free radical generation and the degradation of fat and fat-like molecules (Jayasooriya et al., 2007). The combination of these two phenomena may have increased TBARS in the ST muscle during cold storage.
The RW% content was not significantly different in sonicated ST muscle over the storage period (p>0.05). However, it was tended to increase numerically in sonicated meat samples over the cold storing period. Our results are similar to the results obtained by Chang et al. (2015) who reported higher water-loss rates in ultrasound treated meat samples than in CON samples. Reduced WHC may be caused by the destruction of the structural integrity of myofibrils due to HIU, leading to higher water releasing rates over the storage period. Furthermore, the generation of greater cavitation effects by HIU produces cellular rupture, which subsequently enhances the movement of water molecules out of muscle tissues (Siró et al., 2009). In some instances, ultrasound treatment has been shown to increase WHC in muscle tissues, along with HIU and chilled storage conditions (Caraveo-Suarez et al., 2022; Chang et al., 2015). The cooking loss of HIU-treated meat on days 0 and 7 was increased due to both aging and HIU treatment (p<0.05). In meat, cooking loss and compression losses are considered as the basic indicators of WHC. When cooking loss increases, WHC decreases and vice versa (Chen et al., 2015). Therefore, we speculated that the higher cooking loss in ST muscle was associated with lower WHC during the storage period.
In the current study, the shear force of meat was significantly lower during the storage period in the HIU-treated ST muscle samples (Fig. 1). Especially on days 0 and 7 of storage, the values were significantly lower (p<0.05) due to both aging and HIU application. Previous studies elucidated that the application of the ultrasound technique to bovine muscles resulted in higher tenderness by reducing shear force over the storage periods (Caraveo-Suarez et al., 2021; Chang et al., 2015; Ojha et al., 2016; Siró et al., 2009; Xiong et al., 2012; Zhou et al., 2010). In contrast, studies have reported that ultrasound application caused greater tenderness and shortened the aging period without exerting negative effects due to acoustic cavitation in meat (Lyng et al., 1999). This increased tenderness was mainly correlated to the destruction of myofibrillar protein structures, the disintegration of collagen macromolecules, and the movement of proteins, minerals, and other compounds within the muscle (Stadnik and Dolatowski, 2011). The tenderization of meat can also be influenced by the disruption of lysosomes due to ultrasound assisted shock waves, which consist of cathepsins and intracellular calcium ions that stimulate calpain activity (Alarcon-Rojo et al., 2019; Chang et al., 2015), subsequently reducing the aging period (Chandrapala, 2015). In meat, thermal, chemical, and mechanical effects occur through acoustic cavitation due to ultrasound application. These phenomena influence the formation of hydroxyl radicals and numerous physical factors that further modify tenderness and other physical properties (Taylor et al., 1995).
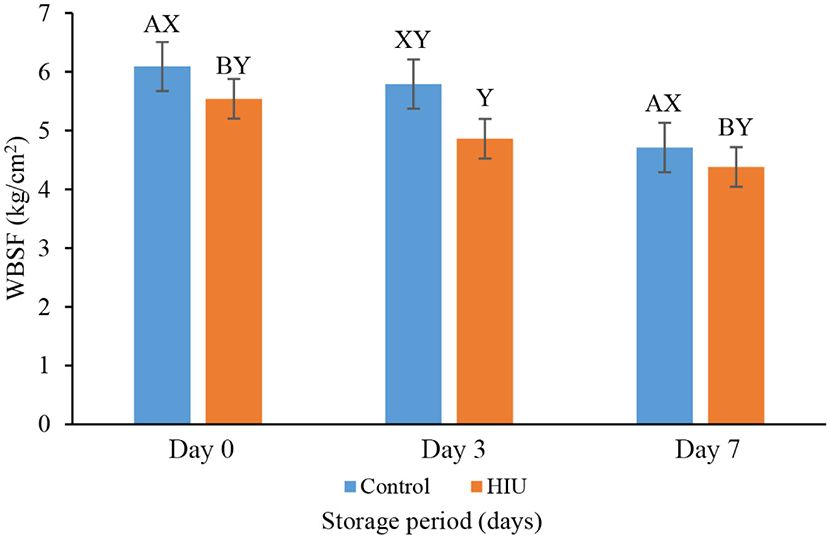
The results showed that HIU treatment significantly increased (p<0.05) the MFI values of bovine ST muscle (63.30%, and 54.44%) at postmortem storage times (days 3 and 7), respectively (Fig. 2). Generally, the MFI evaluates the average length of myofibrils in muscles, and a higher MFI value represents more myofibril ruptures in the I band in sarcomeres during the storage period (Karumendu et al., 2009). The higher MFI value in our study might be linked with meat tenderness and postmortem myofibril proteolysis. The increased MFI value due to increased proteolysis by calpains is then reflected in the increased tenderness of the meat.
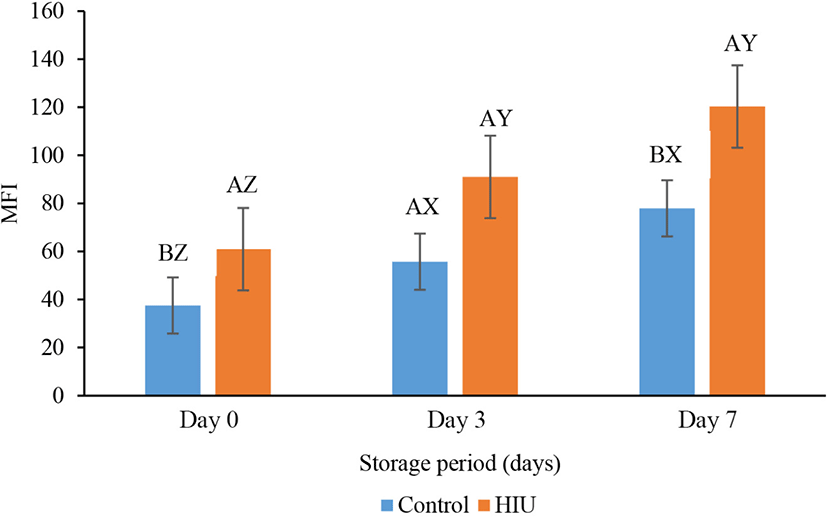
The effect of HIU application contributed to the development of sensory attributes in cooked ST muscle samples as compared to the CON treatment (Fig. 3). The flavor was significantly enhanced in sonicated muscles on days 0 and 3 of the storage period (p<0.05). High tenderness was perceived in sonicated ST muscle samples on days 0, 3, and 7, leading to overall higher sensory traits. Under the application conditions, HIU treatment significantly enhanced (p<0.05) umami flavor in cooked meat during the storage period. However, in the fresh meat sensory evaluation, there were no significant differences (p>0.05) in marbling, surface retention water, and the overall quality of the HIU-treated ST muscle samples (Fig. 4). Nevertheless, fresh meat color was significantly decreased on the third day of storage. This may be related to the results obtained by Chang et al. (2012) and Jayasooriya et al. (2007) who reported that HIU treatment can induce muscle temperature. Consequently, the thermal denaturation and oxidation of the color pigments could negatively affect meat color, as reflected in the fresh meat color sensory evaluation.
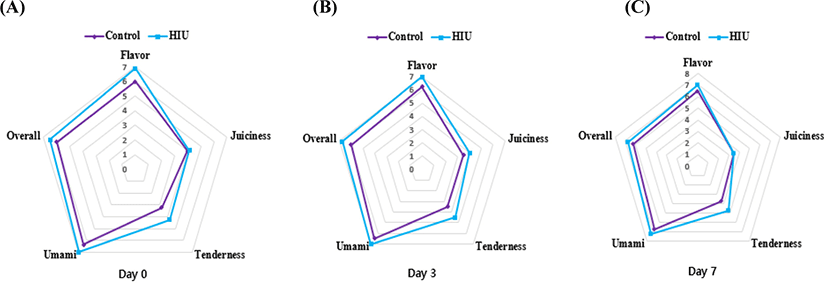
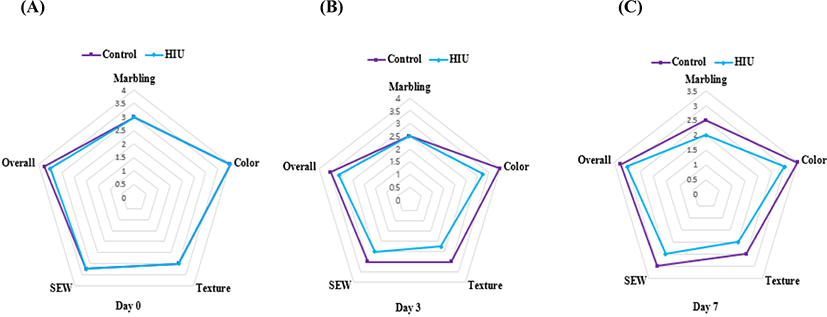
Fig. 5 presents the electronic tongue’s response values for sourness, bitterness, umami, and richness in ST muscle treated with HIU. There were no significant differences in flavor attributes except for umami and bitterness. The umami content was significantly reduced (p<0.05) on day 7 and bitterness was reduced due to HIU application. Kim et al. (2015) and Poojary et al. (2017) reported that umami enhanced meat flavor and suppressed bitterness and astringency.
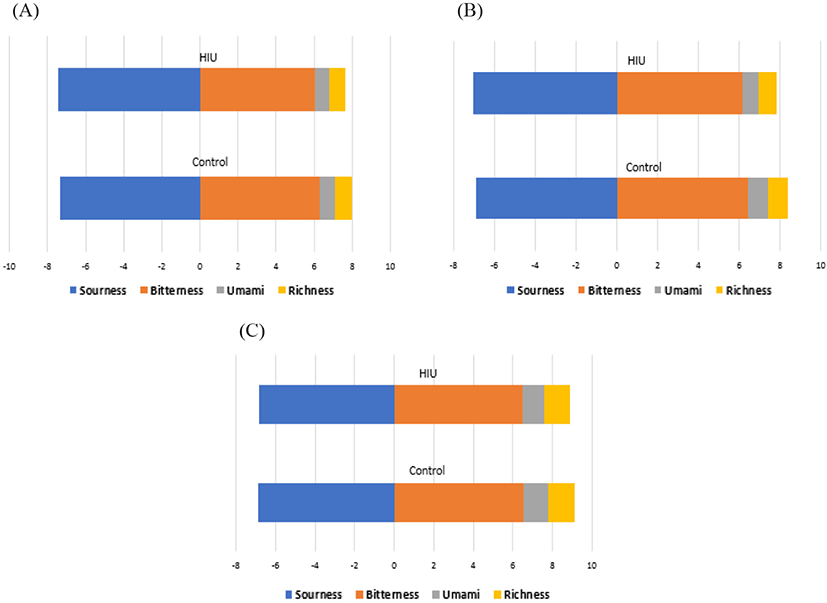
The development of sensory attributes is accelerated by various biochemical enzymatic reactions during the postmortem aging of muscles. Similarly, ultrasound applications can have a significant impact on enzymatic activity, thereby leading to proteolysis and the oxidation of meat compounds. Subsequently, changes in meat color, flavor, and aroma can occur over the storage period (MacLeod, 1998; Ozuna et al., 2013). Zou et al. (2018) found that sonication enhanced the sodium chloride, sugar, 5′-ribonucleotides and their degradation products, essential amino acid and lipid oxidation products, which influence meat sensory characteristics. Furthermore, it increased the concentration of volatile flavor substances including aldehydes, ketones, and alcohols. Ultrasound application induces protein degradation and consequently, free amino acid concentrations, in meat, which could further improve meat sensory traits. We speculated that the high concentration of umami-related compounds such as glutamic, glycine, and alanine affected higher umami intensity in cooked meat due to HIU application. To our best knowledge, only one study has reported lower glutamic, glycine, and alanine content corresponding to increasing ultrasound levels (0, 200, 300, 400 W) in yak ST muscle (Bao et al., 2022). The majority of studies have observed positive sensory effects in spite of the difficulty in analyzing different experiments due to differences in frequency, intensity, and time combinations used in sonicated treatments of meat. Hence, the implementation of HIU in bovine muscle requires further investigation.
The microstructure of the bovine ST muscle in a HIU-treated sample is presented in Fig. 6. Some microstructural alterations such as disorganization in the myofibrillar arrangement in muscle can be observed after HIU application. In general, HIU-treated muscle samples showed disrupted microstructure during the storage period. Especially, swelling in the A-band region was observed, particularly near the H-band on days 3 and 7. Moreover, the area surrounded by the Z-line became much more irregular, whereas the CON treatment displayed a properly compact myofiber matrix. Similar to our results, microstructural misalignment and disintegration at the junction of the Z-line of bovine ST muscle exposed to 25 W/cm2 for 20 or 40 min of ultrasound treatment were observed by Wang et al. (2018). One possible reason for the findings is that ultrasound application increases sarcomere lengths and subsequently affects the stretching of sarcomeres. As a result, the inter-myofibrillar spaces become enlarged and the Z-lines become altered (Dolatowski et al., 2000). In contrast, the changes in the I-band and Z-line in the sarcomere could be associated with the release of Ca2+ into the cytoplasm. The Ca2+ concentration influences calpain activity, which can determine the rate of myofibrillar proteolysis (Peña-González et al., 2017). In the current study, the fragmentation of connective tissue fiber content and the visible longitudinal space between the HIU treated muscle samples on days 0, 3, and 7 due to cavitation energy was obviously visible. Generally, ultrasound induced cavitation and the collapse of bubbles create shockwaves in muscles. Consequently, the destruction of connective tissues leads to overall changes in muscle architecture (Wang et al., 2018).
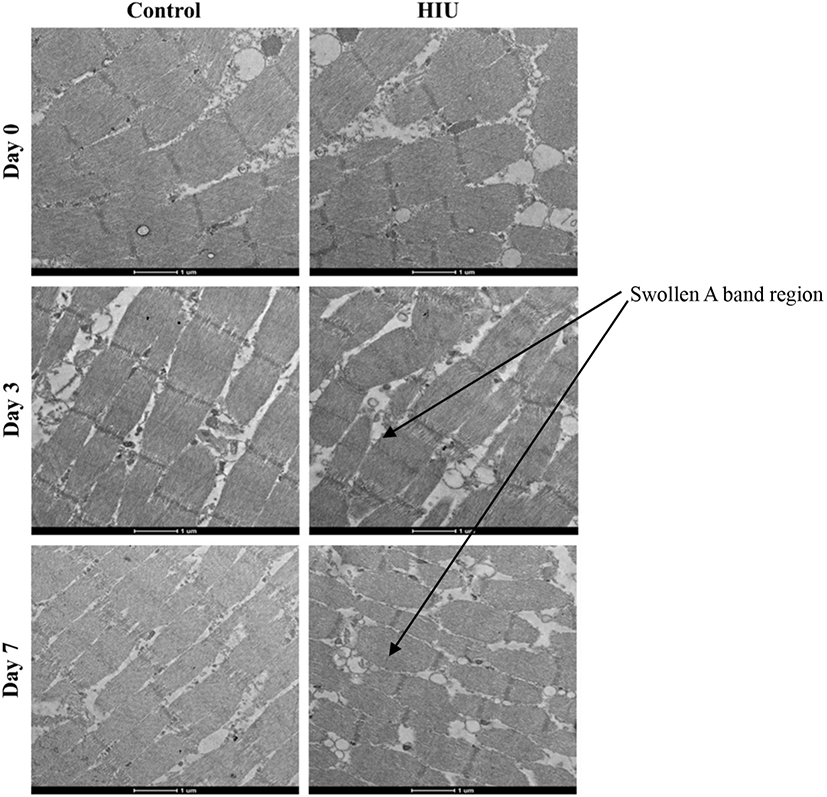
The relative fatty acid composition data were clustered into four different groups according to Euclidian distance (Fig. 7). As is evident from the clustering, the relative docosahexaenoic acid (C22:6n3) content was reduced (high blue intensity) on the third day of the storage period, and a higher relative proportion was seen in the CON group. Similarly in the second cluster, the content of C22:6n3 was lower in the CON group. However, on day 0, the C22:6n3 content was distributed almost equally in both two treatments. The relative composition of α-linolenic acid (C20:5n3) and eicosapentaenoic acid (C18:3n3) was increased (high red intensity) in HIU-treated meat samples on the third day of storage. In the present study, there was no significant impact of HIU treatment on saturated fatty acid (SFA) and polyunsaturated fatty acid content. However, a study by Bao et al. (2022) concluded that higher SFA content in dry-cured yak meat occurred from 400 W and the 20 kHz ultrasound application. They speculated that the cavitation effect of HIU application accelerated the oxidation of unsaturated fatty acids, and thus, ultimately, the SFA content tended to increase. Nevertheless, knowledge concerning the effect of HIU remains mostly focused on physio-chemical traits, whereas for sensory attributes, and it remains limited to the fatty acid composition. Therefore, further investigations should be conducted to elucidate the effect of HIU application on the above-mentioned parameters.
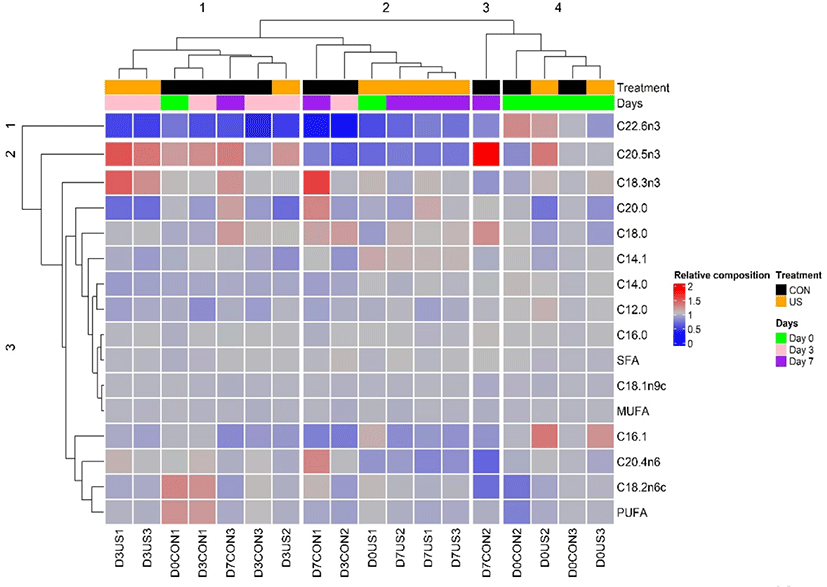
Conclusion
Implementing HIU treatment, especially for meat, is a promising strategy for improving meat quality and achieving a more sustainable food production process. In the present study, the exposure of bovine ST muscle to HIU (35 kHz and 800 W/cm2) for 60 min after the storage period improved tenderness and MFI values without negatively affecting other meat quality attributes. Based on the evaluated meat sensory attributes, the flavor, tenderness, and umami content were enhanced in cooked meat treated with HIU. Even though HIU application did not significantly impact the electronic tongue evaluations, the values remained at optimum levels. Microscopically, the sonication of meat induced the degradation of connective tissues, reflected in higher tenderization effects and spaces among the muscle fiber matrix over the cold storage period. However, further scientific explorations are required to evaluate the effect of HIU application on fatty acid composition in meat. It is also necessary to determine the free amino acid composition in bovine muscle to better clarify the mechanisms behind the effects of HIU on meat sensory attributes. In summary, HIU is an emerging technology that could add value to indigenous cattle breeds and provide new insight into the growing meat industry.