Introduction
Lactoferrin is classified as a member of the transferrin family based on amino acid sequence homology (Lambert et al., 2005) and similarities in tertiary structure (Baker et al., 2002). Most transferrin family proteins are composed of a single polypeptide chain with two lobes and glycochains, and have metal-binding properties. As shown in many previous studies and reviews, lactoferrin has various biological functions (Brock, 2002; Nuijens et al., 1996; Orsi, 2004; Shimazaki, 2000; Wakabayashi et al., 2006). Of these, antibacterial activity was the first function reported. The presence of a bacteria-inhibiting substance in bovine milk was reported by Cheeseman and Jayne-Willams (1964). They confirmed that such substances were protein-based, but they were not able to identify the compound. Four years after their study, the bacteriostatic activity of lactoferrin was reported by Reiter and Oram (1967). Such bacteriostatic activity is due to the iron-chelating properties of lactoferrin.
Antibacterial peptides such as lactoferricin, lactoferrampin, and kaliocin-1 have been isolated from enzymatic hydrolysates of the bovine or human lactoferrin N-lobe. Lactoferricin is a cationic N-terminal peptide derived from bovine lactoferrin (lactoferricin B, residues 17-41) (Bellamy et al., 1992) and human lactoferrin (lactoferricin H, residues 1-49) (Hunter et al., 2005). These antibacterial fragments show very broad antibacterial spectra. The essential core sequences responsible for antibacterial activity were reported to be RRWQWR (residues 20-25) for lactoferricin B and FQWQRNMRKVR (residues 21-31) for lactoferricin H (Tomita et al., 1994). Lactoferrampin is a peptide derived from bovine lactoferrin residues 265-284 (van der Kraan et al., 2004). Kaliocin-1 is a peptide (residues 151-181) isolated from human lactoferrin and effective in suppressing the growth of Candida spp. (Viejo-Diaz et al., 2005). On the other hand, lactoferrin N-lobe fragments with bifidobacteria growth-promoting properties have been reported (Liepke et al., 2002). Direct interactions of lactoferrin-derived antibacterial peptides with the lipid bilayer of the bacterial cytoplasmic membrane have been reported for lactoferricin (Umeyama et al., 2006; Vogel et al., 2002) and lactoferrampin (Haney et al., 2007). Such an antibacterial mechanism has been suggested to be similar to those of other antibacterial peptides such as magainin (Matsuzaki, 1999). The location of lactoferricin B, lactoferrampin, and the sequence corresponding to human kaliocin-1 are shown in Fig. 1.
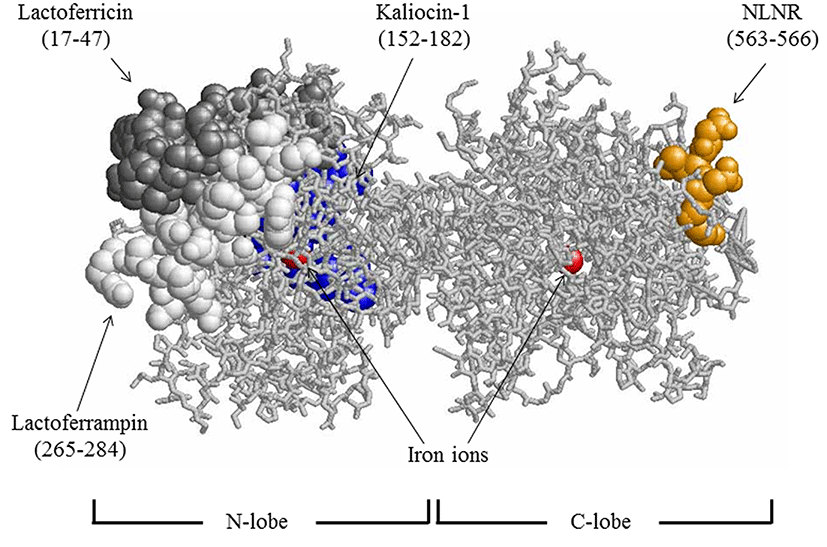
In this study, we investigated the antibacterial effects of synthetic short peptides derived from the bovine lactoferrin sequence on certain kinds of bacteria including Lactobacillus acidophilus, Escherichia coli, Pseudomonas fluorescens, and P. syringae.
Materials and Methods
L. acidophilus CH-2 was obtained from Chr. Hansen A/S (Denmark), and E. coli JCM1649 was purchased from RIKEN BRC-JCM (Japan). P. fluorescens DSM 50090 and P. syringae DSM5175 were obtained from the DSMZ-German Collection of Microorganisms and Cell Cultures (Germany). Bovine lactoferrin was obtained from Morinaga Milk Industry Co., Ltd. (Japan). TPCK-treated trypsin (Type XIII, Sigma Chem. Co., Ltd., USA) was used for lactoferrin hydrolysis. Peptides were synthesized using the Fmoc method at Sigma-Aldrich Japan (Japan). The centrifugal ultrafiltration membrane apparatus (Apollo® 7 mL UF) was obtained from Orbital Biosciences, LLC (USA) and Minisart was obtained from Sartorius Biotech GmbH (Germany).
Bovine lactoferrin was initially dissolved in 0.05 M Tris-HCl buffer (pH 7.8) containing 0.02 M CaCl2, then mixed with trypsin solution at a substrate/enzyme weight ratio of 1/50, and incubated at 37℃ for 12 h. Afterward, the reaction mixture was heated at 80℃ for 15 min to stop the enzymatic reaction. The hydrolyzed solution was subjected to centrifugal ultrafiltration, and the filtrate passing through the membrane (cut-off size, 5 kDa) was collected. The filtrate was further separated into its components on a preparative reverse-phase column of TSKgel ODS-80TM (column size, 21.5 mm I.D. × 30 cm; Tosoh, Japan) using a high-performance liquid chromatography (HPLC) system as shown in Fig. 2. Linear gradient elution was carried out with 0.01% trifluoroacetic acid (TFA) in water and 5-50% acetonitrile. The flow rate was 4.0 mL/min, and ab- sorbance was measured at 220 nm to detect the eluted compounds. After removal of acetonitrile and TFA in the fractions using a centrifugal evaporator, each solution was neutralized by adding alkaline solution. All fractions were assayed for their effects on bacterial growth.
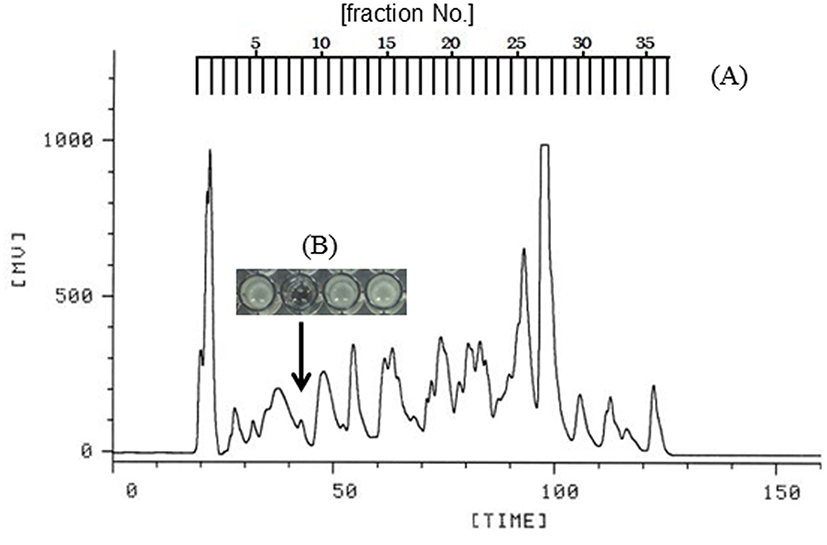
The effect of each peptide against L. acidophilus CH-2, E. coli JCM1649, P. fluorescens JCM1649, and P. syringae DSM5175 was measured both by disc assay and turbidity measurements at 620 nm. L. acidophilus was preincubated in MRS broth at 37℃, and E. coli was preincubated in Luria-Bertani (LB) medium at 37℃. P. fluorescens and P. syringae were preincubated in LB medium at 30 and 25℃, respectively. Each peptide solution was filtered through a 0.2 μm (pore-size) membrane filter to remove microorganism contaminants. Disc assays were performed according to the standard method (Bruhn et al., 1985) with some modifications. Ten milliliters of agar containing MRS or LB medium was mixed with 1% (v/v) of the precultured target bacteria in a petri dish (diameter, 10 cm), and then overlaid with agar containing each medium. Sterile filter paper discs (8 mm diameter) were placed on the agar surface, and 30 μL of each peptide solution was adsorbed on the filter paper disc. Then, the petri dishes containing agar and paper discs were maintained in an upright position for drying at room temperature before being inverted and incubated at the appropriate temperature for 24 h. Antimicrobial activities of the sample solutions were evaluated according to the size of the growth inhibition zones. Turbidimetric measurements for bacterial growth were performed using 96-well ELISA plates. The results represent average values obtained from triplicate assays. In our previous study, the relationship between absorbance and CFU was confirmed for the growth of L. acidophilus (Kim et al., 2004).
Results and Discussion
Lactoferrin was digested with trypsin, and fragments with a molecular weight <5 kDa were collected by ultrafiltration. The fragments were separated into 36 fractions by reverse-phase column chromatography, as shown in Fig. 2A. The 36 fractions were assayed for antibacterial activity against E. coli. Fraction 8 inhibited growth of E. coli (Fig. 2B). Hence, fraction 8 was selected to further study the antibacterial effect against pathogenic bacteria. The chromatographic chart for sequencing of fraction 8 suggested that this fraction contained peptides starting from the sequence of Asn-Leu-Asn-Arg. Thus, we chemically synthesized the tetrapeptide Asn-Leu-Asn-Arg. This peptide is located in the C-lobe (residues 563-566) as Fig. 1.
From the disc assay (Fig. 3), inhibition zones or a clear zone of L. acidophilus was not observed with synthetic Asn-Leu-Asn-Arg. On the other hand, synthetic Asn-Leu-Asn-Arg showed distinct growth inhibitory activity against E. coli, P. fluorescens, and P. syringae (Fig. 3). Furthermore, antibacterial activity test results of synthesized Asn-Leu-Asn-Arg were confirmed by turbidity measurements, and data for Asn-Leu-Asn-Arg is shown in Fig. 4, which shows the time- and concentration-dependent effects of the synthetic peptide on bacterial growth. Synthetic Asn-Leu-Asn-Arg exhibited significant antibacterial activity against E. coli, P. fluorescens, and P. syringae (Fig. 4). However, this peptide showed no inhibitory effect on the growth of L. acidophilus, and actually showed a slight growth-promoting effect. As shown in Fig. 4, the peptide Asn-Leu-Asn-Arg strongly promoted L. acidophilus growth at all concentrations tested. The stimulating activity of Asn-Leu-Asn-Arg against L. acidophilus was lower than that of the human lactoferrin fragment against bifidobacteria, as reported by Liepke et al. (2002). It is generally recognized that the suppression vs. stimulation of bacterial growth by proteins and peptides depends on the strain and culture media. Thus, it is possible that under other conditions these peptides express more potent activity.
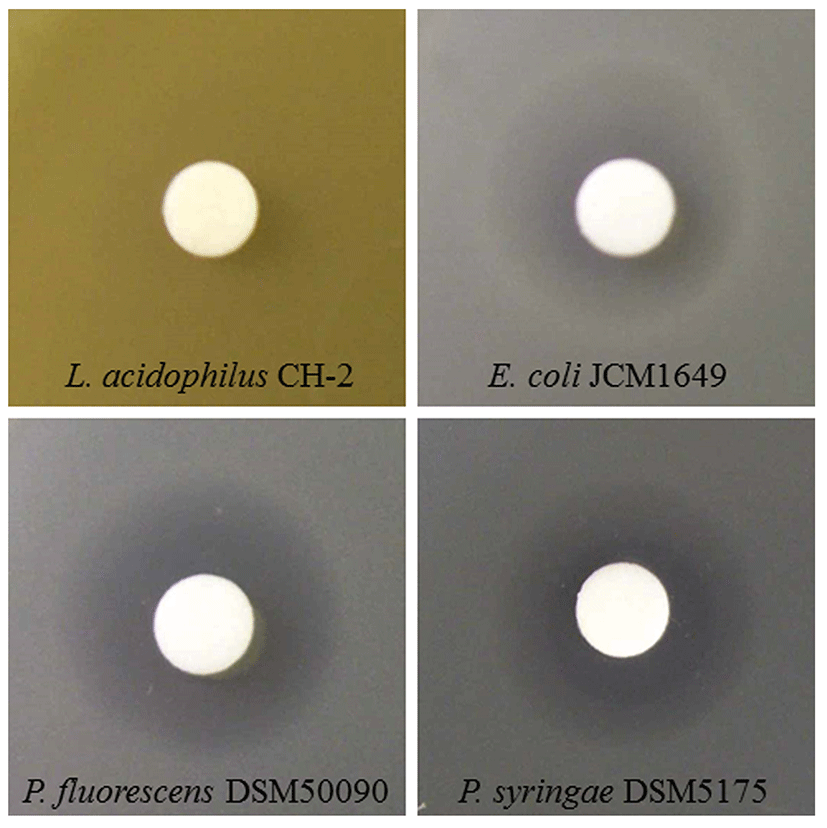
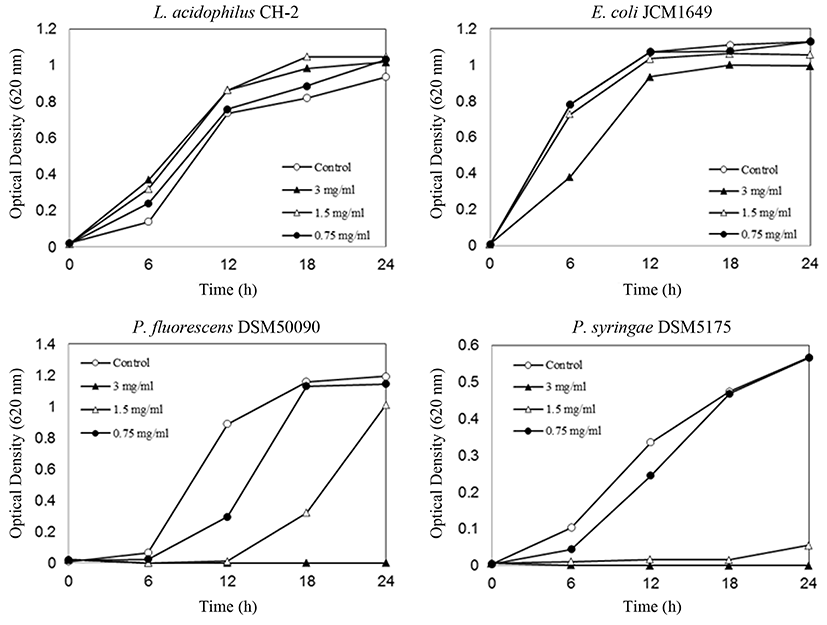
L. acidophilus is a lactic acid bacteria used for producing fermented milk (Robinson and Tamime, 1981). P. fluorescens is a psychrotrophic bacteria that accounts for 67 to 95% of bacteria found in raw and pasteurized milk (Craven and Macauley, 1992). These bacteria contaminate milk and reduce its quality during refrigerated transport after pasteurization and packaging even at low temperatures (Garcia et al., 1989). P. syringae is a harmful bacterium that is highly infectious to plants and that induces necrosis of leaves, fruits, and stems (Vidaver, 2002).
Considering the relationship between lactoferrin and microorganisms, the lactoferrin molecule has various modes of action against different microorganisms, one of which is growth suppression of various bacteria through its bacteriostatic or bactericidal action.
Another mode of action is the stimulation of bacterial growth seen in lactic acid bacteria and bifidobacteria. These actions imply that that lactoferrin has many mechanisms for protecting the host against bacterial infection. Certain kinds of lactic acid bacteria and bifidobacteria stimulate the mucosal immune system of intestinal tissues (Gill, 1998; Ziemer and Gibson, 1998). Considering such effects, lactoferrin plays important roles in maintaining the integrity and function of living tissues through various mechanisms. On the other hand, some bacteria survive by utilizing lactoferrin as an iron source. One of these groups is the Neisseriaceae family, and bacteria belonging to this family do not secrete siderophores and have lactoferrin-binding proteins on their cell surface (Gray-Owen and Schryvers, 1996).
Several mechanisms have been proposed to explain the bactericidal effects of lactoferrin. Lactoferrin molecules can interact or bind directly to bacterial cell surface components such as lipopolysaccharides (Ellison et al., 1988), porins (Sallmann et al., 1999), and other proteins, and such interaction induces the degradation of bacterial cells. The lipopolysaccharide-binding site is located in the N-terminal portion of lactoferrin (Elass-Rochard et al., 1995; van Berkel et al., 1997; Wang et al., 1995), and the N-terminal site composed of up to 42 residues is responsible for binding porin (OmpC) (Sallmann et al., 1999). It was also reported that residues 473-538 of bovine lactoferrin bind to surface protein antigen (PAc) to prevent the binding and growth of Streptococcus mutans, a tooth decayinducing bacterium (Mitoma et al., 2001). Moreover, inhibition of S. mutans biofilm formation has also been reported (Berlutti et al., 2004). Another antibacterial mechanism of lactoferrin is the inhibition of attachment and colony formation of Haemophilus influenzae on epithelial cells, through its peptidase activity (Qiu et al., 1998). In addition to the aforementioned mechanisms, lactoferrin also acts on intestinal cells and mucosal tissues to stimulate host immune systems (Kuhara et al., 2000; Kuhara et al., 2006; Takakura et al., 2006).
Some lactoferrin-derived fragments exhibit antibacterial activity, and lactoferricin was the first antibacterial peptide isolated from lactoferrin (Bellamy et al., 1992).
Bellamy et al. (1992) reported that the mean inhibitory concentration (MIC) of lactoferricin B was > 60 mg/mL for P. fluorescens. Jones et al. (1994) reported a 15.6 mg/mL MIC for P. fluoresens. Dionysius and Milne (1997) reported a 50 mg/mL MIC for P. fluorescens isolated from raw milk. The MIC values of lactoferricin B for other Pseudomonas spp. were reported to range from 6-45 mg/mL for five strains of P. aeruginosa (Bellamy et al., 1992), 15.60-31.25 mg/mL for P. aeruginosa, and 31.25 mg/mL for P. aeruginosa 10662 (Jones et al., 1994). The MIC values for other strains, namely, P. putida (1 strain) and P. cepacia (4 strains) were reported to be 15.6 and >500 mg/mL, respectively (Jones et al., 1994). On the other hand, MICs for P. aeruginosa PAK and P. gingivalis were reported to be 14.3 and >200 mg/mL, respectively. Candida spp. are susceptible to kaliocin-1, and the effective growth-inhibitory concentration of this peptide is 480 mg/mL (150 mM) (Viejo-Diaz et al., 2005).
The homologous amino acid sequences found in our study have been searched using FASTA software against the DNA Database of Japan (DDBJ). Many proteins containing the same 4-amino acid sequence as our experimental tetrapeptide (Asn-Leu-Asn-Arg) were identified. Bovine lactoferrin contains only one part of this sequence, and lactoferrin from other animals does not contain the same sequence. This suggests that the bioactive peptide could be produced by many proteins through proteolysis.
Conclusions
Antibacterial activity of bovine lactoferrin hydrolysates was measured in vitro against pathogenic bacteria and lactic acid bacteria. A synthetic peptide, Asn-Leu-Asn-Arg, derived from the bovine lactoferrin C-lobe, showed antibacterial activity against Pseudomonas spp. and E. coli. However, the synthetic Asn-Leu-Asn-Arg stimulated the growth of L. acidophilus. The tetrapeptide Asn-Leu-Asn-Arg is the first antibacterial peptide sequence found in the bovine lactoferrin C-lobe.