Introduction
Global food demand is expected to increase by approximately 56% by 2050 owing to population growth and economic development, thus highlighting the need for sustainable protein sources that can ensure food security while reducing environmental pollution (Ford et al., 2024). Since 2014, the popularity of plant-based analog meat with consumers has grown steadily due to its sustainability (reducing greenhouse gas emissions) and health benefits (low saturated fat and calorie levels, and high fiber content; Miller et al., 2024). Proteins such as tofu, seitan, and tempeh have been consumed since the 1960s, and research is currently underway to produce plant-based meat analogs using a variety of plant proteins, including mushrooms, potatoes, and carrots (Rout et al., 2024). The aspartic and acidic properties and protein structure of mushrooms give them a strong umami flavor, making them a desirable candidate plant protein source within plant-based meat analog production (Singh et al., 2023).
Mushrooms are considered health foods due to their rich nutritional profile, which includes phenolic compounds, proteins, minerals (such as iron, zinc, and potassium), and fiber. These components contribute to their antioxidant, anti-obesity, and anti-cancer properties (Assemie and Abaya, 2022; Mandliya et al., 2022). Mainly used as food, mushrooms are composed of spore-bearing multicellular structures or ‘fruiting bodies’ and mycelium, which are hyphae clusters with a long fibrous structure. In addition, mushrooms are biodegradable and can be grown with low energy consumption, making them an eco-friendly material that can be produced with low greenhouse gas emissions (Im et al., 2023). Fruiting bodies require a relatively long cultivation time of more than six months, whereas mycelium can be cultivated within 15 days to one month through submerged fermentation. Meat substitutes produced from mycelium have excellent nutritional and textural properties, in addition to a meat-like flavor (Kim et al., 2011). Therefore, this study aimed to identify the quality characteristics of mushroom mycelium and explore their potential as a meat substitute, providing a scientific basis for macrofungi utilization as an alternative protein source within plant-based analog meat production.
Materials and Methods
Shiitake mushroom (Lentinula edodes) mycelium were obtained from Ohsooup (Gangneung, Korea) and cultured as follows: Shiitake mycelium was obtained by inoculating aseptically isolated fruiting body tissue onto potato dextrose agar (PDA) solid medium and incubating at 25°C for 20 days. The shiitake mycelium inoculum culture was prepared by taking a portion of shiitake mycelium cultured on PDA medium and inoculating it into 300 mL potato dextrose broth liquid medium, homogenizing it for 40–60 seconds using a high speed homogenizer set at 6,451×g, and incubating it for 10 days in a shaking incubator (temperature 25°C, stirring speed 1.75×g, air supply 0.5 vvm). For mass production, a liquid medium containing yeast extract 0.5% (w/v), glucose 2.0% (w/v), MgSO4-7H2O 0.05%, KH2PO4-12H2O 0.05% (w/v), and silicon-based antifoaming agent (LS-303, Keumgang Silicone Technology, Daejeon, Korea) 0.05% was prepared. The mycelium was recovered by decompression filtration using filter paper with a pore size within 10 μm, and washed three times with distilled water to remove the medium components.
The pre- and post-rinsing weights of the pressed mushroom mycelium were measured to calculate the water rinsing yield. The mushroom mycelium was mixed with distilled water at a ratio of 1:20, sealed in a vacuum pack and placed on a rocker digital (RK-2D, Daihan Scientific, Wonju, Korea) in a refrigerator at 4°C for 24 hours for stirring. Afterwards, it was placed in a 500 mL bottle for centrifuge and centrifuged for 20 min at 4°C using a centrifuge (Supra R22, Hanil, Gimpo, Korea) set at 6,000×g speed, and the mycelium and separated water were suctioned off and the precipitated mycelium was weighed. The weighed value was substituted into the following equation to calculate the water rinsing yield:
The moisture content of the mushroom mycelium was analyzed by the atmospheric pressure drying method (105°C, AOAC 950.46) according to the AOAC (2016) method and expressed as a percentage.
Before and after rinsing, mushroom mycelium and mushroom mycelium sausages were mixed with distilled water at a 1:4 ratio and homogenized for 1 min using an Ultra Turrax homogenizer (HMZ-20DN, Pooglim Tech, Seongnam, Korea) at a speed of 6,451×g. The pH was then measured using a glass electrode pH meter (Model S220, Mettler-Toledo, Schwerzenbach, Switzerland) calibrated with 4.01, pH 7.00, and pH 10.00 buffer solutions (Suntex Instruments, New Taipei City, Taiwan).
A colorimeter (CR-10, Konica Minolta, Osaka, Japan) was used to measure the CIE L*, CIE a*, and CIE b* of the mushroom mycelium and mushroom mycelium sausages before and after rinsing. A white standard plate was used as the reference color with a CIE L* value of +97.83, CIE a* value of –0.43, and CIE b* value of +1.98.
Vials containing 2 g of mushroom mycelium and mushroom mycelium sausage samples before and after rinsing were measured at 70°C using an electronic nose (E-nose; HERACLES-2-E-NOSE, Alpha MOS, Toulouse, France) equipped with two columns MXT-5 (Restek, Bellefonte, PA, USA) and MXT-1701 (Restek). The analytical conditions were set as follows: reaction time 110 s, injection volume 5,000 μL, injection rate 125 μL/s, injection site temperature 200°C, detector temperature 260°C. Data were analyzed using Alphasoft (Alpha MOS).
Before and after rinsing, the mushroom mycelium and mushroom mycelium sausages were mixed with distilled water at a 1:4 ratio and homogenized using a homogenizer (AM-5, Nissei, Anjo, Japan) for 1 min, and filter paper (Whatman No. 1, Whatman, Maidstone, UK) was used to extract the supernatant. The supernatant was diluted 1,000-fold with distilled water and measured using an electronic tongue (E-tongue) system (Astree 5; Alpha MOS). The CTS (salty), NMS (umami), and AHS (acidic) sensors; PKS and ANS (standard sensors); and SCS and CPS (sensors) were used to determine the signals of the flavor components represented by each sensor.
The formulation ratios of the sausages are presented in Table 1. Pork ham (24 h after slaughter; Jeong-Dam, Suwon, Korea) was used as raw meat, which was ground using a grinder (PA-82, Mainca, Horton, UK) equipped with a 3 mm plate. The final emulsion was prepared using a bowl cutter (K-30, Talsa, Trujillo, Peru) to mix and ground the raw meat, shaved ice, and mushroom mycelium three times for 1 min. The prepared emulsion was filled in a natural hog casing using a stuffer (EM-12, Mainca), heated in a chamber (10.10ESI/S, Alto Shaam, Menomonee Falls, WI, USA) set at 80°C for 30 min and cooled at room temperature for 30 min. The sausages, sealed in vacuum packs, were stored in a refrigerator at 4°C and used in the experiments.
Ingredients (%) | Wet mushroom mycelium (%) | |||||
---|---|---|---|---|---|---|
C (control) | MM25 | MM50 | MM75 | MM100 | ||
Main | Meat | 80 | 60 | 40 | 20 | 0 |
Wet mushroom mycelium | 0 | 20 | 40 | 60 | 80 | |
Ice | 20 | 20 | 20 | 20 | 20 | |
Additives | Salt | 1.5 | 1.5 | 1.5 | 1.5 | 1.5 |
The general composition of the mushroom mycelium sausage was determined according to the AOAC (2016) method, and the moisture content was analyzed by the normal pressure drying method according to AOAC 950.46, the crude protein content by the Kjeldahl method according to AOAC 992.15, the crude fat content by the Soxhlet method according to AOAC 960.39, and the ash content by the direct painting method according to AOAC 920.153, and expressed as a percentage. Carbohydrate content was roughly calculated by subtracting moisture, crude protein, crude fat, and crude ash values from 100.
The cooking yield was calculated by weighing the mushroom mycelium sausages before and after heating. The heating conditions were the same as those used in the sausage manufacturing process. The weighed values were substituted into the following equation to calculate cooking yield:
The viscosity of the mushroom mycelium sausage emulsion was measured for 1 min using a rotational viscometer (MerlinVR, Rheosys, Trenton, NJ, USA) equipped with a 2 mm gap in a 30 mm parallel plate set at a head speed of 0.015×g at a temperature of 25°C.
3.5 g of the pork and mushroom mycelium sausage, 50 mL of distilled water, and 50 mL of cooking oil were homogenized for 2 min with an ultra-Turrax (HMZ-20DN, Pooglim Tech) at 5,822×g. The homogenate was then transferred to a cylinder, and after 3 h, the middle scale of the separated homogenate and emulsified layer were measured and calculated by substituting into the following equation:
A texture analyzer (TA 1, Lloyd, Phoenix, AZ, USA) was used to measure the texture profiles of a sample cut to a height of 1 cm and a diameter of 2.8 cm. A 100 mm cylinder probe was mounted and measured under the following analysis conditions: pre-test speed 2.0 mm/s, post-test speed 5.0 mm/s, maximum load 2 kg, head speed 2.0 mm/s, distance 8.0 mm, force 5 g. Hardness (kgf), springiness, cohesiveness, chewiness (kgf), and gumminess (kgf) were measured.
Sensory evaluation was approved by the Kongju National University Institutional Review Board (Authority No: KNU_ IRB_2024-124). Sensory evaluation was conducted by selecting 17 panelists with specialized knowledge of the samples and familiarity with the evaluation criteria. Heat-treated mushroom mycelium-treated sausages were evaluated on a 10-point scale for color, pork flavor, mushroom flavor, tenderness, juiciness, and overall acceptability per treatment group (pork/mushroom flavor: 10=high pork/mushroom flavor, 1=low pork/mushroom flavor, color and overall acceptability: 10=positive, 1=negative; tenderness: 10=high tenderness, 1=low tenderness, juiciness: 10=high juiciness, 1=low juiciness), and the evaluations were averaged for comparison.
All experiments were repeated at least three times to analyze the results, and the mean values and SD of the results were expressed using SAS (version 9.4, SAS Institute, Cary, NC, USA). One-way analysis of variance was used to analyze the results of the experiments, and the mean comparison of mushroom mycelium before and after rinsing (pH, color, E-nose) showed significant differences at p<0.01 and p<0.0001 using t-test. Significance between mushroom mycelium sausage samples was verified at p<0.05 level using Ducan’s multiple range test.
Results
The water rinsing yield and moisture content of the cultured mushroom mycelium after rinsing and the pH and color before and after rinsing are shown in Table 2. The water rinsing yield and water content of the cultured mushroom mycelium is ~81% and 81.00±0.38, respectively. Girometta et al. (2019) reported that the moisture content of mushroom mycelium-based composites was 59%–80% (based on wet mass), which was similar to our results. In addition, the study by Aiduang et al. (2022) showed a moisture content of 61.23%–74.51%, and reported that the moisture content of mycelium can vary depending on the substrate type and fungal species. The pH was significantly higher after water rinsing, and the color was significantly higher after water rinsing for CIE L* and water rinsing for and CIE b* (p<0.05).
Principal component analysis (Fig. 1A) revealed 99.918% and 0.06577% reliability for PC1 and PC2, respectively. The difference before and after rinsing was mainly distinguished by PC1. Based on the x-axis, before rinsing was negative and after rinsing was positive, indicating different flavors. Changes in volatile compounds before and after rinsing are shown in Table 3. Alcohols and aldehydes are the major groups that form volatile compounds and are derived from unsaturated fat oxidation and amino acid metabolism (Hu et al., 2024). Ethanol, a primary alcohol, has a strong, irritating, and chemical odor that causes discomfort (Wang et al., 2021) and 2-Methyl-1-propanol causes a musty odor. Acetoin is a naturally-occurring metabolite that primarily stores carbon and has a ‘buttery’ flavor (Inamdar et al., 2020; Qu et al., 2024). Hexanal is an aldehyde compound and metabolite produced by linoleic acid oxidation, which is characterized by an “oxidized” odor that contributes to its unpleasant flavor (Wang et al., 2025; Zhou et al., 2024). Since the values of the negative-flavor producing compounds (ethanol, 2-Methyl-1-propanol, and hexanal) were lower in the mycelium after water rinsing than those before water rinsing, we concluded that water-rinsed mycelium are suitable for meat product manufacturing.
The e-tongue results are shown in (B) of Fig. 1. The salty taste was higher, while the sour umami taste was higher after rinsing. Chemical elements such as sodium (Na+), potassium (K+), and lithium (Li+) and containing Na+, are perceived as ‘salty’ to taste upon ingestion within the amiloride-sensitive pathway (Sood et al., 2024). Higher salty taste of mycelium before rinsing was due to the accumulation of inorganic salts or media residues on the surface; however, the salty taste is reduced when elements such as Na+ and K+ are washed by water. Sourness is influenced by the content or ratio of organic acids, such as lactic acid and citric acid, whereas umami is influenced by aspartic acid, and glutamic acid, with glutamic acid playing an important role in the umami taste of meat (Min et al., 2023; Zhang et al., 2022). Yu et al. (2023) reported that the content of glutamic acid was the highest at 4.93% in a study analyzing the amino acid composition of L. edodes mycelia, and that the content of umami amino acids was 30.83% of the total amino acids. Cell membrane permeability is an indicator of the structure and function of the cell membrane, and when the integrity is damaged due to external factors, the cell membrane is destroyed and the permeability increases, which may cause the contents inside the cell to be released (Qiu et al., 2021; Yan et al., 2021). Zhao et al. (2019) reported that the contents of citric acid, aspartic acid, and glutamic acid increased in L. edodes mycelia when the cell membrane was damaged in a high temperature stress environment. We propose that the rinsing process and stirring for 24 h acted as physical stress on the mushroom mycelium, causing damage to the cell membrane, loss of vitality, and release of internal organic acids, which increased the sour taste. Further, umami taste increased as cell membrane permeability and glutamic acid content increased.
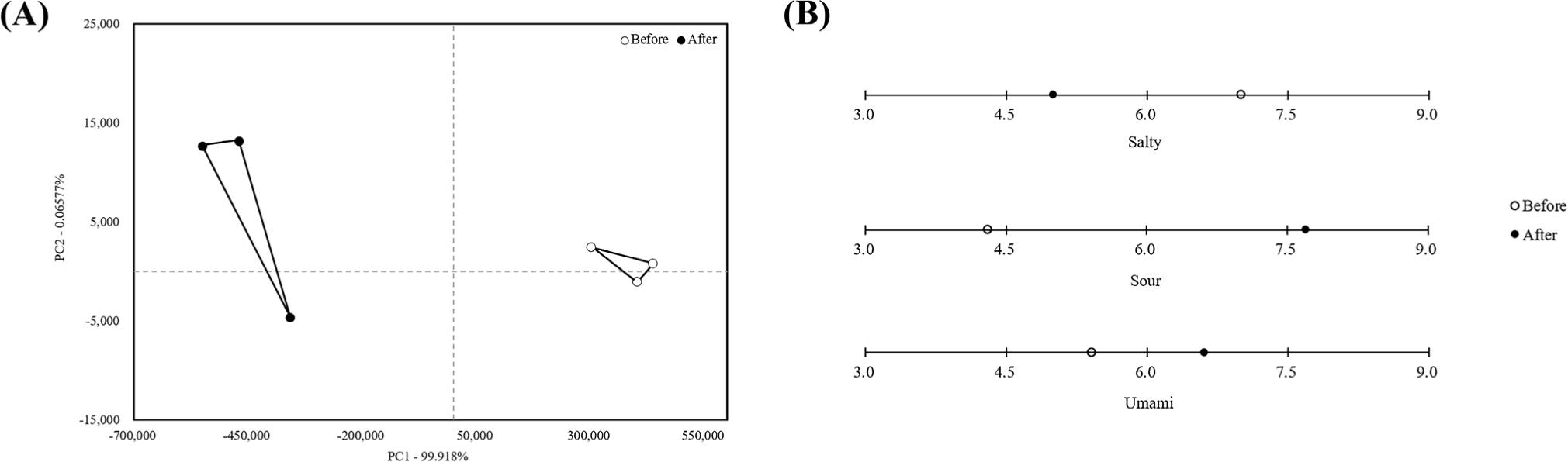
The results of the general component analysis of the mushroom mycelium sausages are shown in Table 4. The moisture content increased significantly with increasing mushroom mycelium content, and the moisture content value was higher in 100% mushroom mycelium without meat treatment (p<0.05) than in that of the controls and other treatments. The dietary fibers contained in mushrooms have a porous structure that can absorb and bind water, thus maintaining emulsion gel stability in meat products (Baek et al., 2024; Boylu et al., 2022). Therefore, sausage moisture content is enhanced by the action of dietary fiber in the mushroom mycelium, which has hydrophilic properties. Kumari (2020) reported a mushroom moisture content of ~89%, and proposed that the MM100 treatment had the highest moisture content because of the high moisture content of the mushrooms themselves. However, protein content significantly decreased with increasing myceliuml content (p<0.05). This result may be due to the higher moisture content of the mycelium, which resulted in a relative decrease in protein content. In sausages with oyster mushroom powder, Jung et al. (2022) found that increasing the mushroom powder content increased the moisture content but decreased the protein content, which is similar to the results of this study. Fat content decreased significantly with increasing mushroom mycelium content (p<0.05), and ash content was significantly higher in all treatments with mushroom mycelium than that in the control (p<0.05). The addition of dietary fiber to emulsified meat products increases moisture content and decreases fat content because of the high water-absorption capacity of dietary fiber (Ham et al., 2017). In addition, previous research has observed that the fat content of sausage decreases as the amount of added mushroom mycelium increased, owing to the lower fat content of mushroom mycelium than that of pork. Ahmad et al. (2020) reported that an increase in dietary fiber increased ash content, and that replacing part of the chicken with oyster mushrooms in chicken sausages resulted in lower fat content and higher ash content, which is similar to the results of this study. Carbohydrate content tended to increase as the mycelium content increased, and was significantly lower in the control and MM25, and significantly higher in MM75 and MM100 (p<0.05). Ulziijargal and Mau (2011) measured the general composition of various mushroom mycelium and reported that the carbohydrate content was 41.23%–76.80%, of which 8.02%–57.24% was dietary fiber, indicating that mycelium are a rich source of dietary fiber. On the other hand, meat contains a very small amount of carbohydrates, about 1% (Cobos and Díaz, 2015), so it is thought that the carbohydrate content of sausages tends to increase as the amount of mycelium, which has a higher carbohydrate content than meat, increases.
The pH values of the myceliuml sausages before and after heating are presented in Table 4. The pH of the mushroom mycelium sausage emulsion before and mushroom mycelium sausage after heating significantly decreases with increasing mushroom mycelium content (p<0.05). In addition, the pH of the sausages after heating was significantly higher than that of the emulsion before heating in all control and treatment groups (p<0.05). Shiitake mushrooms contain organic acids, such as malic, succinic, fumaric, and lactic acid (Chen et al., 2021). Thus, this suggests that the pH of sausages could be decreased as the amount of mushroom mycelium added increased, which increased the organic acid content. Yang et al. (2021) reported that the pH of meat can increase with protein denaturation during heating, resulting in dynamic changes that rearrange or expose acidic and basic groups. Acidic groups are donors of H+, while basic groups are acceptors of H+ (Aydogdu et al., 2023). Consequently, protein denaturation during the heating process of the sausage may result in the dominance of basic groups over acidic groups due to the exposed basic groups, causing an increase in the pH of the sausage post-heating.
The colorimetric analysis of the mushroom mycelium sausages is shown in Table 4. CIE L* decreases significantly with increasing mycelium content (p<0.05), and CIE a* decreases significantly from the MM50 treatment, except for the MM25 mycelium treatment (p<0.05). CIE b* was significantly higher in all treatments than that in the control (p<0.05). However, CIE b* does not differ significantly between the MM25, MM50, and MM75 treatments (p>0.05). The CIE L* of the mushroom mycelium itself is 53.977±0.32, with 5.43±0.28 and 8.60±0.11 for CIE a* and CIE b*, respectively. CIE L* of the control with meat alone is 71.34±0.38, with 6.27±0.60 CIE a* and 6.19±0.25 CIE b*. Therefore, it is judged that as the amount added increases, the CIE L* and CIE a* increase and the CIE b* decreases due to the color of the mushroom mycelium itself.
The cooking yield of mycelium sausages is shown in Fig. 2A. The MM100 treatment is excluded from the results due to insufficient emulsification from the absence of meat protein. Cooking yield improved with increasing mycelium content, with no significant difference between the control and MM25 treatments (p>0.05); however, the cooking yield of the MM50 and MM75 treatments was significantly higher than that of the control (p<0.05). Myofibrillar proteins play an important role in meat product manufacturing by improving the emulsion stability and texture of meat products. The addition of dietary fiber to meat products provides many benefits, such as improved water retention, gel formation, and fat-binding ability (Mishra et al., 2023). In one study by Zhao et al. (2023), dietary fiber was added to the water of emulsion gels by exposing the hydrophobic groups inside myofibrillar proteins that act on a three-dimensional structure. This was observed to improve emulsion stability, by improved the heating by allowing water molecules to easily adhere to the interior between the densely formed gel structures in the mushroom mycelium. The protein structure of meat is prone to water loss owing to denaturation by heating during the cooking process; however, polysaccharides have a molecular structure containing groups, which have high water content (Han et al., 2019; Zhuang et al., 2020). In addition, Zhou et al. (2022) reported that polysaccharides from Ramaria flava mushrooms maintain a stable structure, even at temperatures that result in water loss. This suggests that during the heating process, polysaccharides contained in the mushroom mycelium can maintain higher structural stability than that in meat proteins, which may explain why the MM50 and MM75 treatments resulted in higher cooking yields than the control in the present study.
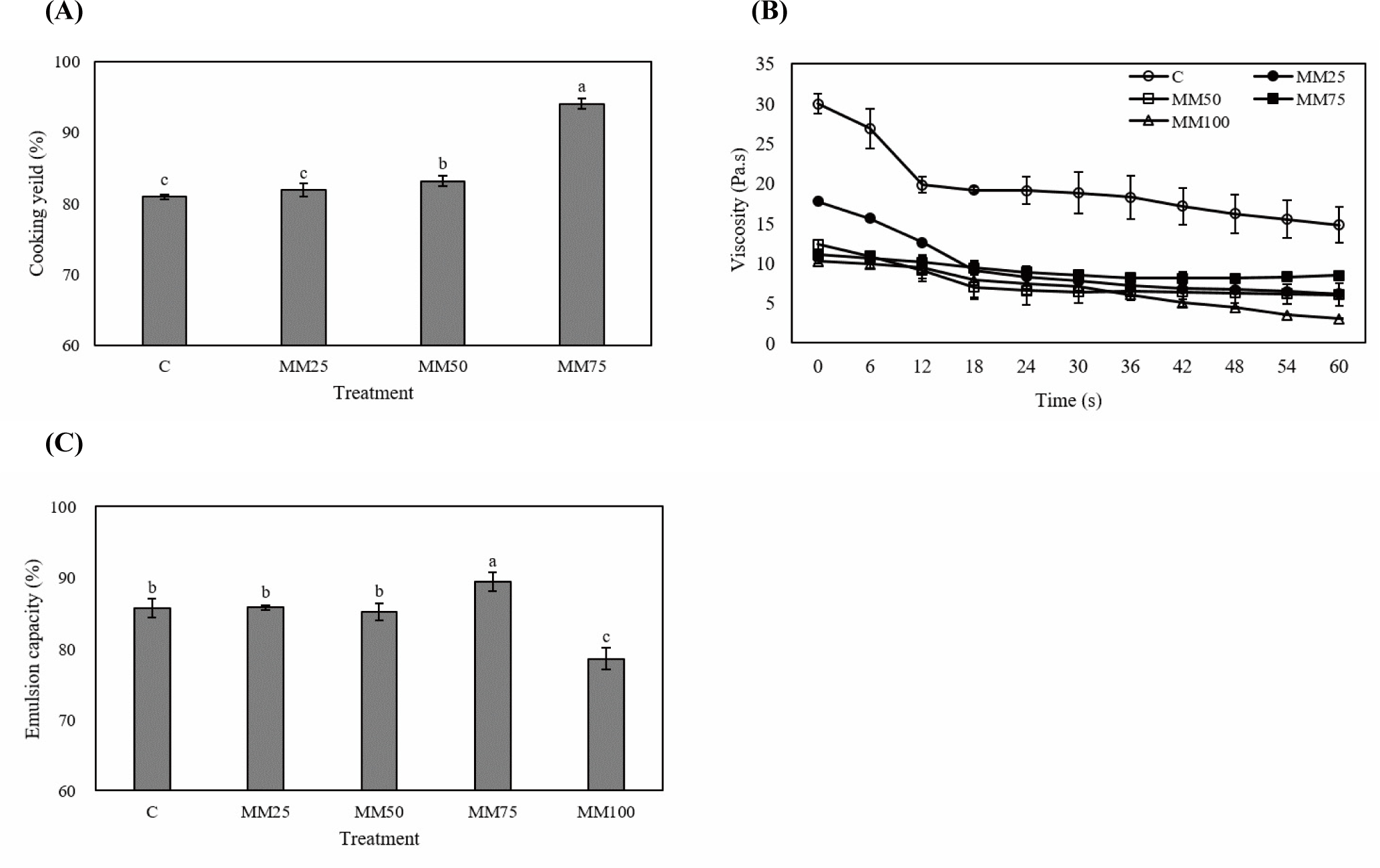
Changes in the viscosity of the mushroom mycelium sausages are shown in Fig. 2B. As the mushroom mycelium content increased, the viscosity of the control and all treatments decreased; the control shows the highest viscosity of all treatments. Although the MM25, MM50, and MM75 treatments had similar values for viscosity, the viscosity of the MM100 treatment was the lowest of all treatments. Viscosity in the MM25 treatment decreased significantly over time (up to 18 s) and was lower than that in the MM50 and MM75 treatments from 42 s onwards. Underlying fiber proteins, such as actin and myosin account for ~60% of meat protein and improve meat product cohesion and gel structure during heating (Ma et al., 2022; Zhao et al., 2023). As vegetable proteins have a different structures and functions than those of meat proteins, gelling agents and emulsifiers are added during vegetable analog meat production to improve the shape, texture, and mechanical properties (Ghebremedhin et al., 2022; Kyriakopoulou et al., 2021). In this experiment, agent emulsifiers were not added to the mushroom mycelium sausage, which resulted in weaker mycelium structures, and all treatments were lower than the control due to the increased moisture content. In addition, the irregular arrangement of protein molecules caused the initial viscosity of the product to increase with increasing resistance; thus, the viscosity value decreases due to the regular arrangement of protein molecules (Kim et al., 2009). In summary, the overall viscosity tended to decrease with increasing mushroom mycelium content in the control and all treatments.
The emulsion capacity of mushroom mycelium sausages is shown in Fig. 2C. The MM75 treatment shows the highest emulsion capacity, whereas the control, MM25, and MM50 treatments show no significant differences; the emulsion capacity value is significantly lower in the MM100 treatment group than that in all other groups (p<0.05). The addition of dietary fiber during meat manufacturing loosens the protein structure, exposing the hydrophilic groups. These changes play an important role in improving emulsion ability, which in turn improves the cooking yield of meat products (Lee and Kim, 2023). Banerjee et al. (2020) reported that the emulsification stability of nuggets increased due to the improved ability to absorb moisture and oil due to the dietary fiber (32.3%) contained in Flammulina Velutipes. In addition, meat myofibrillar proteins play a role in improving the emulsifying properties of meat products (Xiong, 2018), and polysaccharides derived from plants and fungi can be adsorbed on the oil surface to form a physical barrier, thereby inhibiting oil coagulation and forming a denser interfacial layer, thereby improving the stability of emulsions (Meng et al., 2024). Eliuz and Ayas (2024) reported that when single-cell proteins were produced from L. edodes mycelium and added to emulsion gels, polysaccharides such as β-glucan and chitin from mycelium improved the stability of the gel. Accordingly, it is judged that the control group to the MM75 treatment group showed high emulsifying ability due to the action of myofibrillar proteins and myceliuml polysaccharides. On the other hand, it is judged that the MM100 treatment group showed low emulsifying ability due to the limitation in stably combining water and fat with only mushroom mycelium.
The texture profiles of the mushroom mycelium sausages are listed in Table 4. The MM75 and MM100 treatments are excluded from the results because the sausages could not be molded, and the texture profiles could not be measured. As the amount of mushroom mycelium added increased, except for the MM75 and MM100 treatment groups, hardness, cohesiveness, gumminess, springiness, and chewiness tended to decrease. Cohesiveness did not show a significant difference between the control group and the MM25 treatment group (p>0.05). The hardness of meat products decreases with increasing moisture content or decreasing protein content (Fu et al., 2023; Wang et al., 2019). Therefore, it is judged that the hardness tends to decrease due to increased moisture content and decreased protein content as the mycelium content increases. In addition, the protein content and cohesiveness of meat products have a linear relationship, whereas springiness decreases as the moisture content increases (Yahya and Ting, 2020; Table 4). As the amount of added mushroom mycelium increased, the moisture content increased and the protein content decreases. Accordingly, springiness tends to decrease in inverse proportion to the increasing moisture content, and cohesiveness decreases with decreasing protein content. Fu et al. (2023) reported the hardness, cohesiveness, springiness, and chewiness replacement of chicken breast content with Agaricus bisporus mushrooms in chicken sausages, which showed similar results to the present study.
Principal component analysis results for the mushroom mycelium sausages and volatile compounds predicted according to the peaks are shown in Fig. 3. The values of PC1 and PC2 are 96.155% and 3.792%, respectively, with differences between treatments mainly distinguishable by PC1. Based on the x-axis, the control and MM25 treatments have positive values, the MM50 treatment has both negative and positive values, and the MM75 and MM100 treatments have negative values. Thus, we conclude that differences in flavor can be attributed to the percentage of mushroom mycelium added. As shown in Fig. 3B, peaks 2, 3, 4, and 6 of the control group show higher values than those of the other treatments. The compound in peak 2 is expected to be propenal, which belongs to the aldehyde group, and is a substance generally produced by lipid oxidation reaction and is known to produce an almond-like aroma (Park et al., 2024). The predicted compound in peak 3 was propan-2-one, a member of the ketone family, which is produced during the oxidative breakdown of fats and amino acids. Propan-2-one has a low odor threshold, which contributes to the overall flavor of meat (Meng et al., 2022). 2,3-Pentanedione (the predicted compound of peak 4) is a ketone compound produced β-oxidationmicrobial metabolism, which is associated with an unpleasant, rancid odor and contributes to the off-odor of meat products (Zhang et al., 2024). Therefore, the control containing the highest amount of meat showed significantly higher values could be resulted from the large amounts of propan-2-one and 2,3-pentanedione produced by fat and protein decomposition during heating. Predicted as the compound in peak 6, hexanal is a product of the oxidation of unsaturated ω-6 series fatty acids derived from animal tissues and commonly found in meat products such as sausage and ham. At high concentrations, hexanal causes rancidity and an unpleasant odor, which makes it a useful indicator of meat freshness (Bu et al., 2023; Park et al., 2024). Therefore, the addition of mushroom mycelium helps mitigate unpleasant odors caused by fat oxidation in meat.
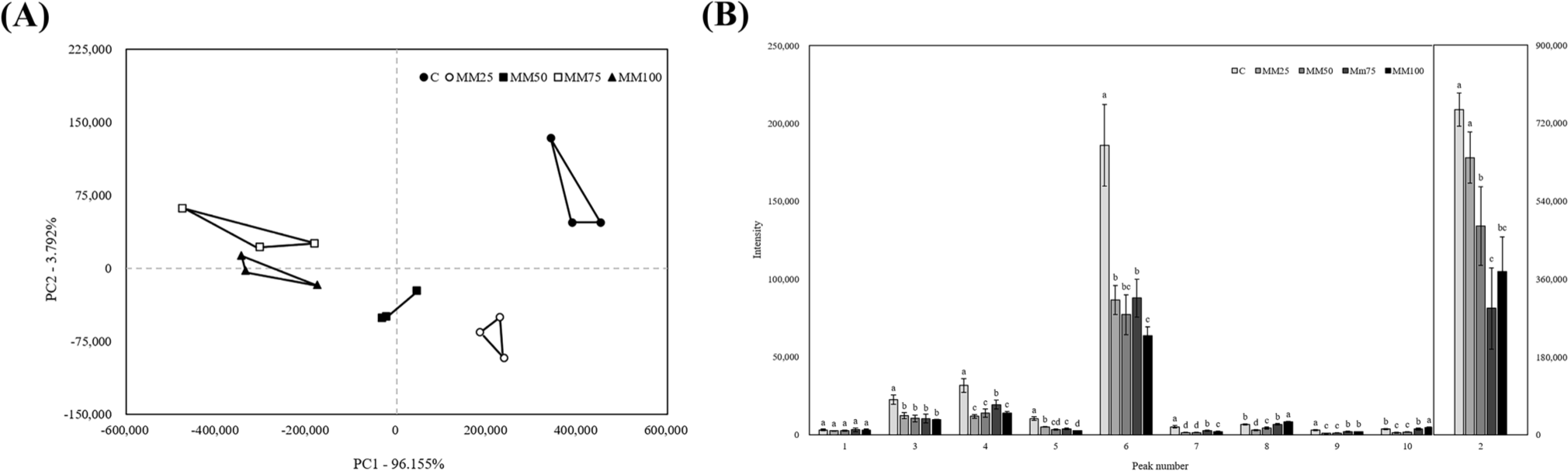
The E-tongue analysis results of the mushroom mycelium sausages are shown in Fig. 4. Among all treatment groups, saltiness and flavor show the lowest values in the control group and the highest values in the MM100 treatment group, which tended to increase with the increasing addition of mycelium. In contrast, umami flavor increased in the order of MM25, MM75, MM50, and MM100 treatment, with the highest value in the control group. Sourness is influenced by pH; that is, the higher the strength of the acid, the sourer the flavor (Clement et al., 2020). In the present study, we observed that the pH of the sausages decreased as the amount of mushroom mycelium increased, and that the sourness of the mushroom mycelium sausages increased as the strength of the acid increased. Umami, which represents a distinct salty taste, enhances the taste of food by suppressing sourness and bitterness, enhancing saltiness, controlling sweetness, and increasing satiety. Edible mushrooms contain compounds such as amino acids (glutamic acid, aspartic acid) and 5'-nucleotides (5'-xanthosine monophosphate, 5'-guanosine monophosphate), which are umami elements (Rangel-Vargas et al., 2021). Go et al. (2022) reported the addition of L. edodes to dumplings and umami flavors, which is similar to the results of the present study.
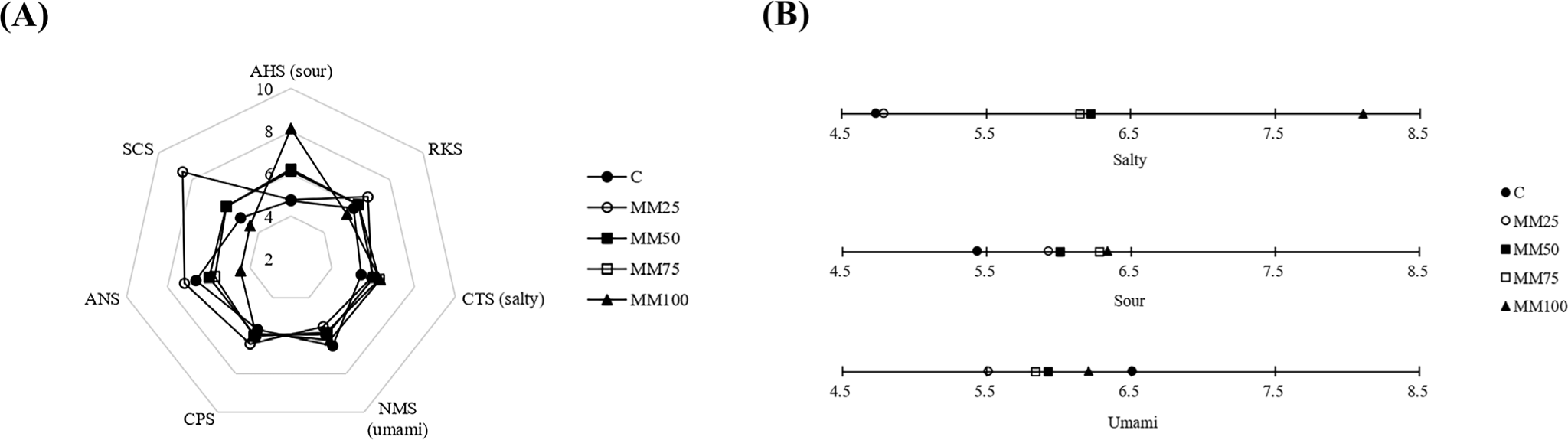
Sensory evaluation results for the mushroom mycelium sausages are shown in Table 4. The color, pork flavor, and overall acceptability tend to decrease with increasing mushroom mycelium addition. Overall acceptability is significantly higher in the control and MM25 treatments than that in the other treatment groups. In contrast, mushroom flavor, tenderness, and juiciness tends to increase as the amount of added mushroom mycelium increased. The moisture content of meat products is an important factor affecting tenderness and juiciness, and maintaining moisture content during the processing is essential to improve these quality attributes (Isleroglu et al., 2015). In addition, Park and Kim (2016) reported that texture profiles are factors affecting the tenderness of meat products, and that an increase in moisture content decreases texture characteristics and increases tenderness and juiciness, which is similar to the present study. Therefore, it is judged that the moisture content of sausages, which increased with the increase in the amount of mushroom mycelium added, improved tenderness and juiciness. On the other hand, although tenderness and juiciness were improved by the addition of mushroom mycelium, the high tenderness and juiciness of the 75 and MM100 treatments were judged to have had a negative effect on the overall acceptability. Wang et al. (2019) reported that as the amount of L. edodes added to sausages in which pork was replaced by L. edodes at 0%, 25%, 50%, 75%, and 100%, the tenderness increased and had a negative effect on consumers, which was similar to our results. Vegetable protein raw materials for expressing the texture of meat proteins include mushrooms, rice, soybeans, and peas, and microbial enzymes can be used to improve gelling characteristics (dos Santos et al., 2023; Mazumder et al., 2023). In order to increase the consumer acceptance of mushroom mycelium sausages, it is believed that follow-up research is needed to improve the gelation properties and texture by adding heterologous proteins such as rice, soybeans, and peas.
Conclusion
This study compared the physical and chemical properties of cultured mushroom mycelium before and after water rinsing, in addition to the physical and chemical properties of sausages with different proportions (C, MM25, MM50, MM75, and MM100) of added mycelium. After rinsing, mycelium pH and color CIE L* increased, whereas CIE a* and CIE b* decreased (p<0.05). The physicochemical differences before and after rinsing were confirmed by principal component analysis (PC1 and PC2). Ethanol, 2-Methyl-1-propanol, acetoin, and hexanal contents were significantly lower after rinsing than those before rinsing (p<0.05). The salty flavor was higher before water rinsing, whereas the sour and umami flavor was higher after water rinsing. The results of the general composition analysis of mushroom mycelium sausages showed that the moisture content increased and the protein and fat content decreased as the mushroom mycelium content increased (p<0.05). The pH decreased as the mycelium content increased (p<0.05). The color analysis results showed that CIE L* and CIE a* decreased, and CIE b* increased as the mycelium content increased. Cooking yield increased in the MM50 and MM75 treatments (p<0.05). Viscosity and physical properties decreased with increasing mycelium addition, and the emulsion capacity was significantly higher in the MM75 treatment group than that in the other treatment groups (p<0.05). E-nose and e-tongue analysis results showed that the addition of mushroom mycelium tended to alleviate unpleasant odors caused by lipid oxidation and increased saltiness and umami tastes. Sensory evaluation showed that the color, pork flavor, and overall acceptability decreased as the amount of mushroom mycelium increased, while the mushroom flavor, tenderness, juiciness to increase with increasing mushroom mycelium content. Therefore, the addition of up to 50% mycelium is deemed the most suitable for plant-based analog meat sausages. However, further research on improving the texture by adding heterologous materials is necessary.