Introduction
Nitrites and nitrates are extensively used as curing agents in the meat industry and play essential roles in processed meat products. These compounds are responsible for the development of the characteristic pinkish-red color and distinctive flavor of cured meat products while preserving product quality by inhibiting lipid oxidation and suppressing the growth of pathogenic microorganisms, particularly Clostridium botulinum (Ferysiuk and Wójciak, 2020; Shakil et al., 2022; Yong et al., 2021). However, concerns regarding the potential health risks associated with N-nitrosamines and the carcinogenic compounds formed when nitrites or nitrates react with amines under specific conditions, such as high temperatures or acidic environments, have led to the re-evaluation of their use in meat processing (Asioli et al., 2017; Niklas et al., 2023; Sebranek and Bacus, 2007). This has resulted in increased consumer and regulatory demands for the reduction or elimination of nitrite and nitrate during meat processing (Hur et al., 2018; Hur et al., 2019).
To address these concerns, researchers and the food industry have been investigating natural alternatives to achieve the desired color, flavor, and safety attributes of cured meat products. A promising approach involves the use of Zinc protoporphyrin IX (ZnPP), a naturally occurring red pigment identified in certain dry-cured meat products such as Parma ham (Asaduzzaman et al., 2020; Wakamatsu et al., 2020; Wang et al., 2021). ZnPP provides a stable and visually red color without the need for synthetic nitrites. Structurally, ZnPP is a metalloporphyrin analogous to heme which incorporates zinc into the porphyrin ring instead of iron (Møller et al., 2007; Wakamatsu, 2022; Wakamatsu et al., 2004a). It functions as the primary red pigment in nitrate/nitrite-free cured meat products, imparting a characteristic bright red appearance (Wakamatsu, 2022; Zhai et al., 2023).
ZnPP formation in meat and meat products involves three primary pathways: endogenous enzymatic (Abril et al., 2021; Khozroughi et al., 2017; Llauger et al., 2023), non-enzymatic (Becker et al., 2012; Wang et al., 2021; Zhai et al., 2023), and bacterial enzymatic pathways (Asaduzzaman et al., 2020; Kauser-Ul-Alam et al., 2021; Wu et al., 2023). Although these mechanisms have not been fully elucidated yet, they are influenced by various intrinsic and extrinsic factors, such as pH, salt concentration, muscle fiber type, oxygen availability, and processing parameters, including temperature and time (De Maere et al., 2016; Parolari et al., 2016; Wakamatsu et al., 2009; Wakamatsu et al., 2010; Wakamatsu et al., 2015). For instance, optimal pH conditions and specific microorganisms can facilitate ZnPP formation, thereby enabling the production of nitrite-free fermented meat products with a desirable color (Kauser-Ul-Alam et al., 2020; Wakamatsu et al., 2019). In contrast, nitrite inhibits ZnPP formation by suppressing protoporphyrin IX (PPIX) production (Wakamatsu et al., 2010). Furthermore, variations in ZnPP formation have been observed among different meat types, with horsemeat and liver exhibiting the highest production levels (De Maere et al., 2017; De Maere et al., 2018).
The significance of ZnPP extends beyond its capacity to maintain the red coloration of meat products. It addresses health concerns associated with nitrite use, particularly the formation of carcinogenic N-nitrosamines (Chau et al., 2011; Skibsted, 2011). Consumer demand for clean-label products continues to increase, with ZnPP representing a natural and potentially safe alternative for producers seeking to reduce or eliminate nitrite additives (Parolari et al., 2016; Wakamatsu, 2022). However, practical challenges persist, including the necessity to optimize processing conditions, regulate pH, control oxygen exposure, and ensure cost-effectiveness. Despite extensive research on ZnPP formation, standardized industrial applications remain insufficiently explored. Addressing these challenges is crucial for the successful industrial application of ZnPP and development of standardized practices.
ZnPP exhibits potential for use in traditionally cured meat products. Emerging applications encompass their incorporation into cultured meat and plant-based meat analogs, which may benefit from their natural red coloration and low health risks. Therefore, this review aimed to (1) elucidate the chemical structure and properties of ZnPP and its role in meat coloration, (2) delineate the mechanisms of ZnPP formation, (3) assess its applications and benefits in the meat industry, and (4) examine the current challenges and future prospects for its use as a natural colorant.
Chemical Structure and Spectral Characteristics of Zinc Protoporphyrin IX in Meat and Meat products
ZnPP is a metalloporphyrin with a unique chemical structure that distinguishes it from other pigments such as nitrosylhemochrome and nitrosomyoglobin (Wakamatsu et al., 2009). In contrast to these pigments, which involve iron coordination with nitric oxide (NO), ZnPP is characterized by zinc coordination with PPIX. This distinctive feature enables ZnPP to impart a vibrant red coloration, particularly in nitrate- and nitrite-free cured products such as Parma ham and Iberian ham (Bou et al., 2018; De Maere et al., 2014; Wakamatsu et al., 2009).
As shown in Fig. 1, ZnPP formation involves substitution of the central iron atom in PPIX with zinc ions. PPIX is a key precursor in heme biosynthesis and is essential for the function of hemoglobin and myoglobin, which are responsible for oxygen transport and storage in blood and muscle tissues, respectively (Wakamatsu et al., 2010). The transformation of PPIX into ZnPP can occur naturally during meat aging, prolonged storage, or under controlled processing conditions. For instance, enzymatic pathways involving ferrochelatase (FECH) play a critical role in facilitating this substitution, particularly under low-oxygen conditions, which are typical in dry-curing methods (Adamsen et al., 2003; Wakamatsu, 2022).
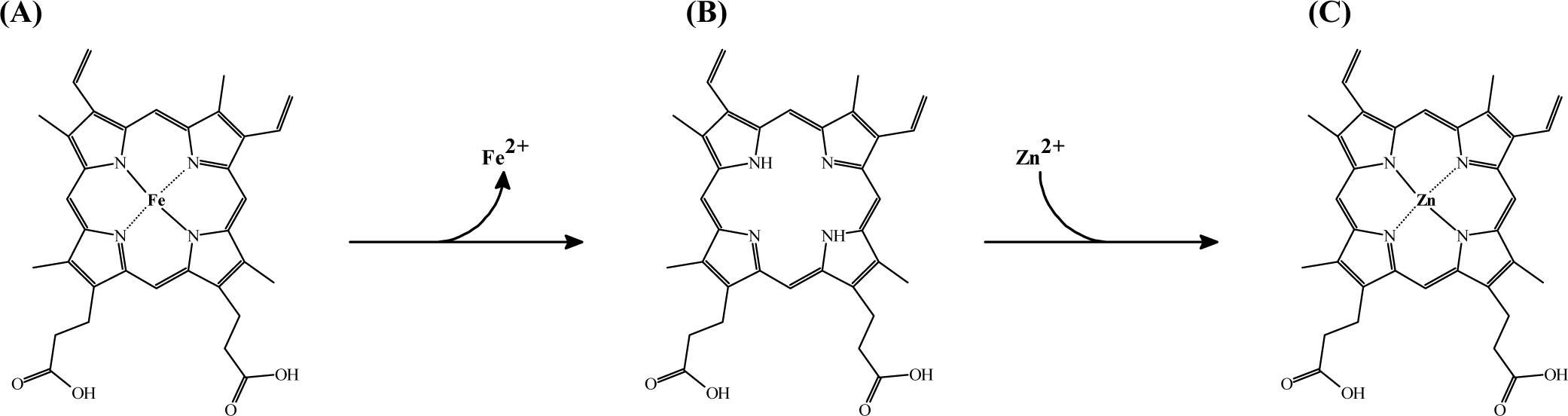
The substitution of iron with zinc not only alters the structural properties of PPIX but also enhances its functional characteristics. Compared to heme, ZnPP exhibits greater stability against oxidative degradation and thermal damage, rendering it particularly suitable for applications in nitrite-free meat processing. For example, studies have demonstrated that ZnPP retains its coloration even under extended curing and storage conditions, thereby maintaining the quality and shelf life of the products (Adamsen et al., 2004; Llauger et al., 2024). These distinctive structural and functional properties of ZnPP also influence its spectroscopic characteristics, thereby enabling precise identification in meat products.
In addition to its structural and functional advantages, ZnPP exhibits distinct spectroscopic and fluorescent characteristics. ZnPP exhibits a distinctive absorption spectrum that plays a crucial role in its identification and quantification in meat products. Its Soret band is observed at approximately 409–418 nm, with Q-band peaks varying according to the meat matrix and curing conditions. For instance, in Parma ham, the Soret band was observed at 409 nm, whereas Q-band peaks occurred at 509, 553, 600, and 637 nm, confirming ZnPP as the primary pigment in these products (Wakamatsu et al., 2004a). Furthermore, studies on acetone/water extracts of matured Parma and Iberian ham identified a Soret band at 418 nm and Q-band peaks at 546 and 584 nm, confirming the role of ZnPP as the dominant pigment in nitrite-free curing processes (Adamsen et al., 2004). These absorption characteristics clearly distinguish ZnPP from myoglobin derivatives such as oxymyoglobin, deoxymyoglobin, and nitrosomyoglobin (Møller et al., 2003).
In addition to its distinct absorption spectrum, ZnPP exhibits unique fluorescence properties. Upon excitation at 420 nm, ZnPP emits a characteristic fluorescence peak near 590 nm, which distinguishes it from other heme pigments (Khozroughi et al., 2017; Wakamatsu et al., 2004b). This fluorescence is directly attributed to the zinc-porphyrin complex structure, offering insights into the stability and role of ZnPP in nitrite-free products. Wakamatsu et al. (2004a) provided initial empirical evidence that the stable red color characteristic of traditional Parma ham is attributable to ZnPP, thereby challenging previous assumptions that assigned the color to an unidentified myoglobin derivative (Morita et al., 1996). Moreover, fluorescence analysis revealed that ZnPP is more stable in low-oxygen environments, which are typical of dry-cured meats, facilitating its widespread application in products such as Parma ham (Wakamatsu et al., 2004b; Wakamatsu et al., 2006).
The fluorescent properties of ZnPP have facilitated advancements in meat processing and quality control. Techniques, such as purple LED imaging, have enabled researchers to visualize the distribution of ZnPP in products such as Parma ham, revealing its higher concentration in intermuscular and subcutaneous fat tissues than in lean tissues. These findings have provided critical insights into ZnPP formation and migration during curing, contributing to the consistent coloration of nitrite-free meat products (Wakamatsu et al., 2006). By utilizing these properties, meat processors can monitor and optimize curing processes, ensuring a stable product quality and extended shelf life (Wakamatsu, 2022).
Mechanisms of Zinc Protoporphyrin IX Formation in Meat Products
The formation of ZnPP in meat products involves complex biochemical processes, which are only partially understood. Studies have reported three primary pathways contributing to ZnPP formation: endogenous enzymatic, non-enzymatic, and bacterial enzymatic pathways (Asaduzzaman et al., 2020; De Maere et al., 2016; Wakamatsu et al., 2010). Table 1 summarizes the mechanisms underlying each pathway as described in the literature. However, ZnPP formation is not governed by a single pathway but rather results from the combined influence of enzymatic, non-enzymatic, and bacterial mechanisms. The relative contribution of each pathway varies depending on the meat matrix and environmental factors, necessitating consideration of processing conditions when optimizing ZnPP formation.
Pathway type | Sample description | Processing conditions | Mechanism | Key insights | References |
---|---|---|---|---|---|
Enzymatic | Dry-cured Serrano hams with varied post-mortem pH values | Aging for 12 months at 3°C–25°C, 60%–85% RH | Ferrochelatase (FECH) activity enables Fe removal and Zn insertion into heme, modulated by pH and salt. | ZnPP content was higher in low post-mortem pH (≤5.4) hams; salting time affected salt but not ZnPP content. | Bou et al. (2020) |
Enzymatic | Fresh meat from multiple animal sources (pork, chicken, turkey, lamb, beef, veal, horse, porcine liver) | Anaerobic incubation at 26°C for 7 d | Zinc chelatase activity promotes endogenous ZnPP formation, especially in liver and horsemeat. | ZnPP formation varied by meat type; liver and horsemeat exhibited high potential. | De Maere et al. (2017) |
Enzymatic | Pork meat extracts | Anaerobic incubation at 30°C for 72 h | Fe(II)-Zn(II) substitution in myoglobin drives ZnPP formation without myoglobin degradation. | ZnPP increased during storage; intact myoglobin showed enzymatic substitution potential. | Khozroughi et al. (2017) |
Enzymatic | Ultrasound-treated pork liver extracts | Ultrasound treatment (400 W, 24 kHz) followed by incubation at 37°C | Ultrasonic cavitation enhances FECH activity, promoting ZnPP production. | 33% increase in ZnPP yield observed with ultrasound; prolonged exposure may degrade enzymes. | Abril et al. (2021) |
Enzymatic | Dried pork liver samples | Drying at moderate (10°C–20°C) vs. extreme (−10°C, 70°C) temperatures | Moderate drying preserves enzyme activity for optimal ZnPP formation. | Extreme drying conditions reduced ZnPP yield; moderate drying enabled efficient nitrite-free curing. | Abril et al. (2022) |
Non-enzymatic | Parma ham and experimental pork models | Anaerobic incubation at 35°C, pH 5.5 for 10 d | Weak heme stability in hemoglobin drives ZnPP complex formation with apo-hemoglobin. | Hemoglobin crucial for nitrite-free color development; myoglobin played a minor role. | Zhai et al. (2022) |
Non-enzymatic | Parma ham | Frozen at −20°C, water extraction | ZnPP binds to hemoglobin and myoglobin, forming stable complexes. | Non-enzymatic pathways crucial for color stability in nitrite-free Parma ham. | Wang et al. (2021) |
Non-enzymatic | Pork loin and liver | Anaerobic incubation at 35°C, pH 5.5 | Ferriheme dissociates, reducing to ferroheme; ZnPP forms without nitrites/nitrates. | Stable red color developed naturally; alternative to synthetic colorants. | Zhai et al. (2023) |
Non-enzymatic | Dry cured Parma hams | Aging at low temperature (3°C–4°C) | Enzyme-independent ZnPP formation observed under cold conditions. | Low temperature reduced enzymatic activity but allowed non-enzymatic ZnPP synthesis. | Parolari et al. (2016) |
Non-enzymatic | Nitrite-free dry fermented sausages | Aging at pH>4.9 | Iron-to-zinc substitution in protoporphyrin IX (PPIX) occurs non-enzymatically, influenced by pH and maturation time. | ZnPP formation correlated with product redness in nitrite-free sausages. | De Maere et al. (2016) |
Bacterial | Pork muscle inoculated with Pseudomonas fluorescens | Anaerobic incubation at 30°C for 120 h | Bacterial FECH catalyzes Zn(II) insertion into PPIX, but muscle matrix limits efficacy. | ZnPP concentration in liquid media was 2.5 times higher than in meat muscle. | Khozroughi et al. (2018) |
Bacterial | Parma ham inoculated with Leuconostoc strains and sausage models | Fermented at 20°C for 30 d | Bacterial FECH promotes ZnPP formation by incorporating Zn(II) into PPIX in aqueous systems. | Leuconostoc mesenteroides achieved CIE a* comparable to nitrite-cured sausages. | Wu et al. (2023) |
Bacterial | High ZnPP-forming food-grade lactic acid bacteria (LAB) inoculated in pork | Incubation at 18°C for 14 d | Bacteria-induced ZnPP enhances red color in nitrite-free meat products. | Heat-stable red color achieved, indicating potential for commercial applications. | Asaduzzaman et al. (2020) |
Bacterial | LAB inoculated in minced meat | Anaerobic incubation at 25°C for 7 d | LAB produces ZnPP through Zn insertion into PPIX under salt conditions. | Promising food-grade bacteria for replacing nitrite in meat products. | Kauser-Ul-Alam et al. (2021) |
Bacterial | Bacterial isolates (non-food grade) from homogenate | Anaerobic incubation at 25°C for 5 d | Resident bacteria facilitate ZnPP formation, with optimal pH shifted to 5.5 in their presence. | Serratia liquefaciens showed the highest ZnPP formation; Carnobacterium divergens offered stable ZnPP production. | Wakamatsu et al. (2020) |
The formation of ZnPP in meat products is predominantly driven by the enzymatic activity of FECH (EC 4.99.1.1), a mitochondrial enzyme that catalyzes the substitution of zinc for iron in PPIX (Becker et al., 2012; Wakamatsu et al., 2015). Under typical physiological conditions, FECH exhibits strong specificity for ferrous iron, thereby facilitating heme biosynthesis. However, during the curing of meat products, particularly in low-oxygen environments, FECH preferentially incorporates Zn into PPIX, leading to the formation of ZnPP (Bou et al., 2020; Parolari et al., 2016). This process is influenced by a combination of intrinsic factors, including pH, temperature, and the availability of metal ions. Optimal activity for ZnPP synthesis has been observed within a pH range of 5.5 to 8.0, with slight variations depending on the source of the enzyme and processing conditions (Abril et al., 2021; De Maere et al., 2016). For instance, studies have reported maximum FECH activity in porcine liver extracts at neutral to slightly basic pH levels, while acidic conditions around pH 5.5, have been demonstrated to support efficient ZnPP formation under specific circumstances (Llauger et al., 2023; Parolari et al., 2016).
The role of substrate availability is of equal significance, as the relative concentrations of zinc and iron in the meat matrix directly influence the enzyme substrate specificity. Ferrous iron functions as a competitive inhibitor of zinc insertion, with higher zinc availability enhancing ZnPP accumulation (Becker et al., 2012; Bou et al., 2020). These conditions are frequently observed in dry-cured meat products, in which the curing process promotes a low-oxygen environment, thereby reducing the bioavailability of iron and facilitating zinc incorporation (De Maere et al., 2018; Wakamatsu et al., 2010). The interaction between these factors emphasizes the importance of controlled processing conditions for optimizing ZnPP formation.
Recent advancements in enzymatic extraction techniques are highly promising for enhancing ZnPP formation. Ultrasound-assisted extraction methods can significantly increase FECH activity, with studies indicating a 33% improvement in enzymatic efficiency compared with conventional methods (Abril et al., 2021; Abril et al., 2022). These findings suggest that ultrasound treatment not only accelerates the extraction process but also preserves the functionality of the enzyme, rendering it a promising approach for industrial applications. Furthermore, pork liver, a rich source of FECH, has garnered attention for its role in promoting ZnPP synthesis, offering a sustainable and efficient alternative for producing natural pigments in meat products (Abril et al., 2021; Bou et al., 2020).
The presence of myoglobin, a heme protein that is abundant in meat, contributes to an additional level of complexity in ZnPP formation. Although myoglobin degradation is not strictly necessary for ZnPP synthesis, its oxidation state and interaction with FECH significantly influence the enzymatic pathway (Becker et al., 2012; Khozroughi et al., 2017). Myoglobin functions as a precursor for PPIX, and its structural state can modulate the efficiency of zinc incorporation, further emphasizing the importance of elucidating the protein dynamics in the ZnPP formation process (Llauger et al., 2023; Parolari et al., 2016).
Based on these insights, ZnPP synthesis can be optimized to enhance the natural coloration of meat products in the meat industry, particularly in the context of nitrite-free processing. This approach aligns with increasing consumer demand for clean-label products, underscoring the importance of endogenous enzymatic pathways in achieving both functional and aesthetic qualities in meat products (Bou et al., 2020; Parolari et al., 2016).
In addition to the enzymatic formation of ZnPP, non-enzymatic pathways contribute significantly to its production, particularly in traditional dry-cured meat products, where enzymatic activity is limited. The primary mechanism involves the substitution of iron with zinc in the porphyrin ring of heme proteins, notably hemoglobin and myoglobin (Zhai et al., 2022; Zhai et al., 2023). According to previous reports, the predominant substrate for ZnPP formation is ferriheme, which dissociates from oxidized heme proteins. This process requires the reduction of ferriheme to ferroheme, thereby enabling zinc to replace iron within the porphyrin structure (Zhai et al., 2023).
Complexes of ZnPP with hemoglobin (ZnPP-Hb) and myoglobin (ZnPP-Mb) have been observed, with ZnPP-Hb identified as the predominant water-soluble ZnPP complex in Parma ham (Zhai et al., 2022). These findings underscore the importance of heme protein dynamics in non-enzymatic formation of ZnPP. Moreover, the formation of ZnPP through non-enzymatic pathways is influenced by environmental factors including anaerobic conditions, pH, and temperature. For instance, Becker et al. (2012) demonstrated that a non-enzymatic transmetallization reaction occurs in low-oxygen environments wherein the iron in myoglobin is replaced by zinc. Similarly, Wakamatsu et al. (2019) identified mildly acidic pH conditions (4.9–5.5) as optimal for metal exchange in myoglobin to facilitate efficient ZnPP formation.
De Maere et al. (2016) further emphasized the critical role of pH and production time in promoting ZnPP synthesis in nitrite-free meat products. Their findings revealed that ZnPP formation increased significantly during the later stages of curing under appropriate pH conditions. Notably, non-enzymatic formation of ZnPP frequently occurs concurrently with enzymatic pathways, suggesting a synergistic interaction between the two mechanisms (Becker et al., 2012; De Maere et al., 2016; Zhai et al., 2023).
These insights into the non-enzymatic pathways of ZnPP formation not only deepen our understanding of meat coloration processes, but also underscore the potential for optimizing natural pigment production in nitrite-free meat products.
Among lactic acid bacteria (LAB) strains, Lactococcus lactis subsp. cremoris and Leuconostoc mesenteroides produce more intense red colora in salt-added minced meat, with ZnPP autofluorescence serving as a reliable indicator of the presence of ZnPP (Kauser-Ul-Alam et al., 2021). Moreover, LAB strains exhibit optimal ZnPP-forming activity under mildly acidic conditions (pH 5.5–6.5) and anaerobic environments, which closely resemble the conditions present in fermented meat products (Wakamatsu et al., 2020; Wu et al., 2023). Sodium chloride marginally inhibits ZnPP formation, whereas sodium nitrite completely suppresses the process, underscoring the suitability of LAB for nitrite-free curing systems (Kauser-Ul-Alam et al., 2021; Wakamatsu et al., 2020).
Leuconostoc strains have been isolated from fermented meat products and exhibited significantly enhanced ZnPP formation under vacuum-packed and salted conditions, providing a viable alternative to traditional curing agents (Wu et al., 2023). These findings are consistent with studies indicating that LAB are the primary contributors to ZnPP synthesis in various meat matrices, not only improving color but also ensuring safety and stability during fermentation (Asaduzzaman et al., 2020; Wu et al., 2023). Furthermore, LAB-derived ZnPP formation occurs both aerobically and anaerobically, further emphasizing the versatility of these bacteria in diverse processing environments (Wakamatsu et al., 2020).
The application of LAB in nitrite-free meat processing presents a promising approach to address consumer demand for natural clean-label products, while maintaining desirable sensory attributes. These findings underscore the potential of LAB as an effective substitute for nitrites, offering both functional and safety advantages in meat production.
Factors Influencing Zinc Protoporphyrin IX Formation
The formation of ZnPP in meat products is influenced by multiple factors, including pH, muscle fiber type and composition, processing temperature and time, oxygen concentration, presence of nitrites, sodium chloride concentration, and metal ions (Table 2). A comprehensive understanding of these factors is essential for optimizing ZnPP production for commercial applications, thereby facilitating the precise control of meat processing.
Factors | Objectives | Type of sample used | Experimental design | Major results | Insight outcome | References |
---|---|---|---|---|---|---|
pH levels | Determine optimal pH for ZnPP formation in porcine skeletal muscles. | Porcine skeletal muscles | Analyzed at pH 4.75 and 5.5 based on muscle fiber type. | pH 4.75 favored ZnPP formation in type I fibers, while pH 5.5 was better for type II fibers. | pH levels are crucial for optimizing ZnPP formation based on muscle fiber types. | Wakamatsu et al. (2019) |
pH levels | Investigate ZnPP formation in nitrite-free fermented sausages. | Dry fermented sausages | pH adjusted via dextrose addition during fermentation. | ZnPP formation peaked at pH≥4.9 after extended drying (up to 177 d), demonstrating pH and time dependency. | Promoting pH≥4.9 facilitates ZnPP development for natural coloring in nitrite-free products. | De Maere et al. (2016) |
pH levels | Investigate ZnPP formation in porcine heart extract under varying pH conditions. | Porcine heart extract | Anaerobic incubation at pH levels from 4.5 to 5.5. | Optimal zinc-chelatase activity occurred at pH 5.5, enhanced by ATP presence, while anaerobic conditions stabilized ZnPP formation. | pH 5.5 is critical for enzymatic ZnPP synthesis in porcine heart. | Ishikawa et al. (2006) |
pH levels | Optimize ZnPP formation in porcine liver. | Porcine liver homogenates | Incubated at pH 4.2–5.4 with organic acid (ascorbic and acetic). | ZnPP formation peaked at pH 4.8, with 24-h incubation at 45°C enhancing yield. Microbial safety was maintained under these conditions. | Slightly acidic pH (4.8) enhances ZnPP production in porcine liver. | Llauger et al. (2023) |
pH levels | Study post-mortem pH and salting time effects on ZnPP in nitrite-free Serrano dry-cured hams. | Ham slices from Serrano dry-cured hams | Ham slices with pH at 24 h post-mortem categorized into low (≤5.4), medium (5.4–5.9), and high (≤5.9). | Low pH (≤5.4) increased ZnPP formation but decreased heme content. Reduced salting had minimal impact on ZnPP, but fatty acid levels correlated positively with ZnPP. | Acidic conditions improve ZnPP synthesis in cured hams, despite heme reduction. | Bou et al. (2020) |
Muscle type, meat source, and meat composition | Investigate ZnPP formation in different muscle fiber types under varying pH conditions. | Porcine skeletal muscles | ZnPP levels analyzed in type I (red) and type II (white) fibers at pH 4.75 and 5.5. | ZnPP formation was optimized in type I fibers at pH 4.75 due to high mitochondrial content, while type II fibers favored pH 5.5 due to distinct enzymatic pathways. | Muscle fiber type and pH are key determinants of ZnPP formation, guiding processing strategies. | Wakamatsu et al. (2019) |
Muscle type, meat source, and meat composition | Evaluate ZnPP formation across various meat sources. | Chicken, turkey, pork, lamb, beef, veal, horse, and porcine liver | Compared ZnPP and Zn-chelatase activity in meat homogenates. | Liver and horsemeat showed highest ZnPP formation, due to high Zn-chelatase activity and heme content. | Zn-chelatase activity is a key factor in ZnPP formation across meat types. | De Maere et al. (2017) |
Muscle type, meat source, and meat composition | Assess proteolysis and marbling impacts on ZnPP in Parma hams. | Parma ham | Analyzed proteolysis and fat content. | Greater marbling facilitated ZnPP stability, while proteolysis enhanced heme transmetallation efficiency. | Fat content and proteolysis enhance ZnPP formation in natural curing. | Bou et al. (2018) |
Muscle type, meat source, and meat composition | Study ZnPP formation in red vs. light muscles in nitrite-free cured hams. | Dry-cured ham | Analysis at different curing stages. | Red muscles (Semitendinosus) showed consistently higher ZnPP levels compared to white muscles. | Muscle type influences ZnPP levels due to differences in enzymatic activity. | Parolari et al. (2009) |
Muscle type, meat source, and meat composition | Explore ZnPP distribution between lean and fat tissues in Parma ham using imaging techniques. | Parma ham | Used autofluorescence imaging to map ZnPP distribution | ZnPP was more concentrated in fat than lean regions, particularly in anaerobic conditions. | ZnPP forms in lean meat but accumulates in fat tissues, affecting visual color. | Wakamatsu et al. (2006) |
Processing temperature and time | Investigate effects of temperature on ZnPP formation in dry-cured hams. | Dry-cured hams | Compared cold (4°C) and warm (16°C) maturation conditions. | Warm temperatures (16°C) enhanced ZnPP formation by increasing enzymatic activity, resulting in a deeper red color. | Warmer conditions promote enzymatic ZnPP synthesis effectively. | Parolari et al. (2016) |
Processing temperature and time | Optimize ZnPP formation in porcine liver under varying conditions. | Porcine liver homogenates | Evaluated ZnPP formation across temperatures (25°C–55°C) and times (up to 30 h) | High ZnPP content achieved at 45°C for 24 h; longer times at lower temperatures also effective. | High-temperature curing optimizes ZnPP formation and maintains microbiological safety. | Llauger et al. (2023) |
Processing temperature and time | Study temperature effects on ZnPP formation in Parma ham. | Porcine muscles and Parma ham | Modified curing model at optimal pH and 35°C. | ZnPP peaked at 35°C, with hemoglobin being a more efficient substrate than myoglobin due to its higher affinity for zinc incorporation. | Optimal temperature maximizes enzymatic and non-enzymatic ZnPP production in natural curing. | Zhai et al. (2022) |
Processing temperature and time | Examine drying temperature impacts on ZnPP formation in pork liver. | Pork liver | Compared drying temperature at –10°C–70°C. | Moderate drying temperatures (10°C–20°C) preserved ZnPP levels and enzymatic activity by minimizing thermal denaturation. | Balancing drying temperature is key for maintaining ZnPP and enzyme functionality. | Abril et al. (2022) |
Processing temperature and time | Evaluate ZnPP content changes at various curing stages (6–20 mon). | Parma ham slices | Sequential sampling during 6–20 mon of curing. | ZnPP levels steadily increased during the first 12 mon, stabilizing thereafter, indicating long-term pigment stability. | Longer curing durations stabilize ZnPP and improve final color in nitrite-free dry-cured meat products. | Parolari et al. (2009) |
Oxygen levels | Investigate ZnPP formation by lactic acid bacteria (LAB) inoculation under aerobic and anaerobic conditions. | Fermented sausages | Tested Lactococcus lactis and other LAB strains under different oxygen conditions. | ZnPP formation was significantly higher under anaerobic conditions. L. lactis subsp. cremoris also formed ZnPP under aerobic conditions. | Highlights the potential of L. lactis as a natural nitrite alternative, effective under both oxygen conditions. | Kauser-Ul-Alam et al. (2020) |
Oxygen levels | Evaluate the impact of oxygen on ZnPP formation in porcine skeletal muscles. | Porcine skeletal muscles | Incubated samples at different oxygen levels and pH conditions. | ZnPP formation was significantly inhibited in the presence of oxygen, with optimal production in anaerobic environments at pH 4.75–5.5. | Confirms oxygen as a major inhibitor of ZnPP formation, supporting the importance of anaerobic environments. | Wakamatsu et al. (2019) |
Oxygen levels | Study the spatial distribution of ZnPP in relation to oxygen exposure. | Parma ham | Fluorescent imaging was applied to compare inner (anaerobic) and outer (aerobic) muscle layers. | ZnPP was concentrated in anaerobic inner layers, with reduced levels in oxygen-exposed outer sections. | Reinforces the role of oxygen exclusion in promoting ZnPP accumulation in Parma ham. | Wakamatsu et al. (2006) |
Oxygen levels | Analyze the effects of oxygen on ZnPP formation using oxymyoglobin as a substrate. | Porcine heart extracts | Compared ZnPP production under aerobic and anaerobic conditions. | Anaerobic conditions doubled ZnPP formation compared to aerobic setups, but oxymyoglobin supported ZnPP formation. | Demonstrates that strict oxygen control enhances ZnPP yield even with oxygen-compatible substrates. | Ishikawa et al. (2006) |
Oxygen levels | Investigate the ways oxygen levels and light exposure affect ZnPP stability in sliced Parma ham. | Sliced Parma ham | Compared storage in high (21%) and low (0.4%) oxygen atmospheres with or without light. | Low-oxygen, dark storage preserved ZnPP content and color stability, while high oxygen and light exposure caused rapid discoloration. | Supports the use of low-oxygen, light-protected storage to enhance product quality and shelf life. | Adamsen et al. (2004) |
Presence of nitrites | Study nitrite’s inhibitory effects on ZnPP and protoporphyrin IX (PPIX) formation. | Pork loin homogenates | Tested nitrite-added and nitrite-free systems. | Nitrite significantly inhibited ZnPP and PPIX synthesis by blocking ferrochelatase (FECH) activity. | Nitrite-free curing allows natural ZnPP formation, supporting clean-label trends. | Wakamatsu et al. (2010) |
Presence of nitrites | Compare ZnPP formation in nitrite-free Parma hams vs. nitrite-cured hams. | Parma ham, Iberian ham, and nitrite-cured hams | Matured hams under identical conditions with and without nitrite. | Higher ZnPP levels observed in nitrite-free hams; nitrite suppressed enzymatic activity required for ZnPP formation. | Supports nitrite-free methods as safer and more natural alternatives for cured meats. | Adamsen et al. (2006) |
Presence of nitrites | Investigate nitrite’s impact on ZnPP formation pathways. | Pork muscle homogenates | Evaluated nitrite’s interaction with zinc-chelatase and PPIX. | Nitrite altered heme enzyme activity, inhibiting ZnPP synthesis while promoting nitrosyl-heme pigments. | Nitrites suppress natural ZnPP production, emphasizing the need for nitrite-free curing methods. | Becker et al. (2012) |
Presence of nitrites | Explore ZnPP formation in nitrite/nitrate-free meat systems using high ZnPP-forming bacteria. | Salted minced pork and meat homogenates | Screened 137 bacterial isolates and analyzed their ZnPP formation rates in nitrite-free environments. | Nitrite-free systems showed enhanced ZnPP formation when inoculated with L. lactis, Leuconostoc mesenteroides, and Enterococcus faecium. | Confirms that nitrites inhibit ZnPP formation, and nitrite-free curing with specific bacteria can mimic nitrite-cured coloration. | Asaduzzaman et al. (2020) |
Presence of nitrites | Explore ZnPP formation in nitrite-free dry sausages. | Dry fermented sausages | Compared curing with and without nitrite across extended drying periods. | Nitrite-free sausages had higher ZnPP levels than nitrite-cured ones, particularly under extended curing periods and optimal pH (>4.9). | Long curing times in nitrite-free systems enhance ZnPP, supporting natural coloration alternatives. | De Maere et al. (2016) |
Sodium chloride concentration | Investigate the effect of post mortem pH and salting time on ZnPP formation in Serrano dry-cured hams | Serrano dry-cured hams | Analyzed different salting times (standard vs. reduced) and measured ZnPP levels | Reduced salting time did not significantly affect ZnPP levels, but free fatty acid content correlated with ZnPP formation. | Optimal salt concentration is critical for ZnPP stability in dry-cured hams. | Bou et al. (2020) |
Sodium chloride concentration | Examine the impact of refined salt vs. sea salt on ZnPP formation in Parma-like ham | Parma-like dry-cured ham | Evaluated ZnPP levels over 76 wk of processing using different salt types | ZnPP increased significantly after 40 wk, unaffected by salt impurities. | The type of salt (refined vs. sea salt) does not impact ZnPP formation, indicating minimal effect of trace minerals. | Wakamatsu et al. (2009) |
Sodium chloride concentration | Analyze the role of LAB in ZnPP formation under different NaCl conditions | Minced pork inoculated with LAB strains | ZnPP formation analyzed in 3%–7% NaCl with LAB inoculation | LAB strains maintained ZnPP formation at 3%, but were inhibited at 5% salt. | Salt-resistant LAB strains could be used to optimize ZnPP formation. | Kauser-Ul-Alam et al. (2021) |
Sodium chloride concentration | Examine ZnPP formation in different cured meat products | Parma ham, Iberian ham, nitrite-cured ham | Measured ZnPP content and correlated with NaCl and Zn levels | ZnPP formation was enhanced in non-nitrite cured dry hams but was inhibited in high nitrite and salt conditions. | High NaCl (≤9%) reduced ZnPP formation due to salt-protein interactions. | Adamsen et al. (2006) |
Sodium chloride concentration | Study NaCl impact on Zn-chelatase activity in pork muscle | Pork muscle extracts | ZnPP enzymatic activity measured with different salt concentrations (0–80 g/L NaCl) | ZnPP-promoting activity increased with salt concentration up to 80 g/L. | Zn-chelatase enzyme remains active under high salt conditions. | Benedini et al. (2008) |
Metal ions | Examine the role of Zn2+ in ZnPP formation | Meat and meat extracts | Evaluated ZnPP formation with Zn2+ supplementation | Zn2+ promotes ZnPP formation through enzymatic and non-enzymatic pathways, but high levels inhibit FECH. | Zn2+ is essential for ZnPP synthesis, but its optimal concentration is critical. | Becker et al. (2012) |
Metal ions | Investigate Fe impact on ZnPP formation | Myoglobin, FECH | Studied Zn-Fe transmetallation | Fe2+ can be replaced by Zn2+ in heme, while Fe2+ inhibits ZnPP formation. | Fe2+ removal is a key step in ZnPP synthesis, whereas Fe2+ presence hinders formation. | Paganelli et al. (2016) |
Metal ions | Investigate FECH inhibition by Zn2+ | Purified FECH | Studied Zn2+ effects on FECH activity | Excess Zn2+ inhibits FECH, reducing ZnPP formation. | Zn2+ serves both as a substrate and an inhibitor at high concentrations. | Hunter et al. (2008) |
Metal ions | Evaluate ZnPP formation in nitrite-free dry-cured ham | Dry-cured ham | Analyzed ZnPP levels across different processing conditions | ZnPP formation is influenced by FeCH activity, total iron and salt content. | Proteolysis and iron availability are major determinants of ZnPP formation. | Schivazappa et al. (2024) |
The pH of meat during curing significantly influences the formation of ZnPP, with distinct mechanisms exhibiting different optimal pH levels. For instance, pH 4.75, promotes ZnPP formation through myoglobin degradation, while pH 5.5, facilitates direct zinc incorporation without significant myoglobin breakdown (Wakamatsu et al., 2019). Furthermore, the processing temperature and time significantly affect ZnPP formation depending on the pH level. De Maere et al. (2016) observed that ZnPP formation increased significantly at pH>4.9 in nitrite-free dry fermented sausages, achieving optimal ZnPP levels during prolonged fermentation under these conditions. Similarly, Ishikawa et al. (2006) reported maximum zinc chelatase activity in porcine heart extracts at pH 5.5, exhibiting comparable effectiveness under both anaerobic and aerobic conditions. This versatility demonstrates the potential of ZnPP formation across diverse processing environments.
Benedini et al. (2008) demonstrated that ZnPP formation in pork muscle was significantly enhanced by the presence of NaCl and ATP, with the zinc chelatase activity being most efficient at pH 5.5–6.0. This suggests that the inclusion of NaCl and ATP in curing formulations can synergistically optimize ZnPP synthesis. Consequently, understanding these pH-dependent mechanisms is essential for optimizing ZnPP formation in nitrite/nitrate-free dry-cured meat products. Such optimization ensures desirable color characteristics and improved product stability and consumer acceptance.
Muscle type, meat source, and meat composition are among the most critical factors that influence ZnPP formation. These factors determine the biochemical and enzymatic environments that regulate ZnPP synthesis. Slow-twitch fibers (type I), which are rich in mitochondria, produce higher ZnPP levels at pH 4.75, whereas fast-twitch fibers (type II) exhibit reduced ZnPP formation under the same conditions. These findings underscore the role of mitochondrial activity in ZnPP synthesis (Wakamatsu et al., 2019).
In vitro studies have demonstrated that the source of meat significantly affects ZnPP formation, with horsemeat exhibiting the highest rates under high-pH conditions (approximately pH 5.3–6.0). This was attributed to its elevated zinc chelatase activity and superior heme content and zinc availability compared to other meats (De Maere et al., 2017; De Maere et al., 2018). Furthermore, pork and chicken were found to exhibit lower ZnPP formation rates than horsemeat, highlighting the variability in ZnPP synthesis across different meat sources (De Maere et al., 2018).
Wakamatsu et al. (2006) investigated ZnPP formation across various animal by-products, with porcine liver exhibiting the highest ZnPP-forming capacity. This phenomenon was linked to the mitochondria-rich environment of the hepatocytes, which facilitated ZnPP synthesis without requiring anaerobic conditions. ZnPP distribution studies in Parma ham revealed that intermuscular and subcutaneous fat had significantly higher ZnPP concentrations than lean tissues. This suggests that ZnPP is initially synthesized in lean muscle and subsequently migrates to adipose tissues during curing, where it accumulates due to the lower metabolic activity of fat (Wakamatsu et al., 2006).
Bou et al. (2018) demonstrated a positive correlation between the ZnPP content, proteolysis index, and intramuscular fat content in Parma ham. Proteolysis releases peptides and amino acids that facilitate iron-to-zinc transmetallation, whereas intramuscular fat enhances the retention and distribution of ZnPP. These findings were further corroborated by studies on nitrite-free dried hams, which emphasized the critical roles of marbling and proteolysis in ZnPP formation and their impact on color development (Parolari et al., 2009). These results highlight the significance of meat source, muscle fiber type, and composition in optimizing ZnPP formation, providing valuable insights into nitrite-free, clean-label meat products.
ZnPP formation is a critical factor determining the visual quality and consumer acceptance of nitrite-free meat products and is primarily influenced by the processing conditions, particularly temperature and time. The processing temperature directly modulates the enzymatic activity of FECH at moderate temperatures (10°C–20°C), achieving optimal catalytic efficiency. Both low and high temperatures inhibit enzymatic function, thereby affecting the efficiency and stability of ZnPP synthesis (Abril et al., 2022). Zhai et al. (2022) demonstrated that moderate temperatures not only promote stable ZnPP formation but also ensure the structural integrity of ZnPP-apo-hemoglobin complexes, which are predominant in cured products such as Parma ham. This underscores the dual role of temperature in facilitating pigment formation and ensuring pigment stability in nitrite-free systems.
The enzymatic pathways exhibited significantly reduced activity at lower curing temperatures (3°C–4°C), leading to the predominance of non-enzymatic pathways. However, slower reaction rates increase curing durations by approximately three months compared to conventional methods, posing challenges for industrial scalability and efficiency (Parolari et al., 2016; Zhai et al., 2022). This temperature range, while decelerating the curing process, enhances the stability of non-enzymatically formed ZnPP, particularly in cured products, such as Parma ham, where ZnPP predominantly exists as apo-hemoglobin-bound complexes (Zhai et al., 2022).
The processing duration is equally crucial as it determines the balance between enzymatic and non-enzymatic pathways in ZnPP formation. At moderate curing temperatures, ZnPP accumulation reaches its maximum during the initial and intermediate stages of processing, when the enzymatic activity is robust (Llauger et al., 2023). However, extended durations diminish enzymatic activity due to enzyme degradation, thus reducing ZnPP synthesis (Abril et al., 2022). At low curing temperatures, non-enzymatic mechanisms predominate, necessitating extended processing times to achieve comparable ZnPP levels (Parolari et al., 2009; Parolari et al., 2016).
The combined effects of temperature and time introduce additional complexity into the formation of ZnPP. Llauger et al. (2023) employed a response surface methodology to optimize the parameters for ZnPP synthesis, revealing that anaerobic conditions at 25°C–55°C over 3–30 h yielded high pigment production while maintaining microbiological safety. These findings emphasize the importance of precisely managing processing temperature and time to achieve optimal ZnPP formation in nitrite-free meat products.
In conclusion, the temperature and time are critical determinants of ZnPP formation. Moderate temperatures and shorter durations favor enzymatic pathways, whereas extended periods at lower temperatures promote non-enzymatic mechanisms. Understanding these interactions provides valuable insights into enhancing the quality and visual characteristics of nitrite-free meat products. This knowledge provides a foundation for developing efficient and scalable processing strategies tailored to meet consumer demand for natural clean-label products in the competitive meat industry.
Oxygen availability plays a pivotal role in regulating ZnPP synthesis, directly influencing the balance between enzymatic and non-enzymatic mechanisms. In oxygen-rich environments, ZnPP formation is impeded by oxidation of myoglobin to metmyoglobin, which lacks the capacity to facilitate ZnPP synthesis. For the process to resume, metmyoglobin must be reduced back to its active myoglobin form, a reaction that is inhibited by oxidative stress (Ishikawa et al., 2006; Wakamatsu et al., 2006). Anaerobic conditions are essential for maintaining the enzymatic activities, particularly FECH, a key enzyme in ZnPP synthesis within skeletal muscles (Ishikawa et al., 2006; Wakamatsu et al., 2019).
Porcine skeletal muscle extracts demonstrate significantly higher ZnPP production under low-oxygen conditions because oxygen exposure destabilizes intermediates and oxidizes myoglobin, limiting its capacity to support ZnPP synthesis (Wakamatsu et al., 2004b; Wakamatsu et al., 2019). In contrast, porcine heart tissue exhibits robust ZnPP formation under both aerobic and anaerobic conditions because of its superior enzymatic systems and high mitochondrial activity, which confers resistance to oxidative stress (Ishikawa et al., 2006).
Certain LAB strains, such as L. lactis subsp. cremoris, exhibit the unique ability to facilitate ZnPP synthesis under oxygenated conditions by employing alternative metabolic pathways. This capability renders LAB particularly advantageous in fermented meat products where oxygen exclusion is challenging (Kauser-Ul-Alam et al., 2020; Wakamatsu et al., 2020).
Traditional dry-curing methods, such as those utilized for Parma ham, rely on oxygen-restricted conditions to enhance the accumulation of ZnPP. Fluorescence imaging studies revealed that ZnPP was predominantly localized in the inner layers of Parma ham, where oxygen exposure was minimal. This spatial distribution highlights the critical role of oxygen diffusion in the formation of ZnPP during curing (Wakamatsu et al., 2006).
These findings underscore the significance of oxygen availability in ZnPP synthesis, providing practical insights into nitrite-free meat processing techniques with controlled oxygen levels.
Nitrites and NO significantly impede ZnPP formation via various biochemical pathways (Adamsen et al., 2006; Wakamatsu et al., 2010). Nitrites interact with myoglobin to produce nitrosylmyoglobin, the primary pigment responsible for the characteristic pink color of cured meat products. This reaction reduces the availability of PPIX, which is a precursor required for the synthesis of ZnPP. Studies have demonstrated that nitrites elevate the redox potential of the meat environment, thereby hindering the chelation of zinc into PPIX (Adamsen et al., 2006; Wakamatsu et al., 2010).
In nitrite-free cured products, such as Parma ham, ZnPP forms naturally and contributes to a stable red color. Conversely, nitrite-cured products exhibit negligible ZnPP levels, underscoring the significant inhibitory effects of nitrites (Adamsen et al., 2006; Wakamatsu et al., 2007). Although less reactive than nitrites, nitrates undergo bacterial reduction to form nitrites during the processing. This conversion indirectly inhibits ZnPP formation by acting as a continuous source of NO, albeit at a slower rate than that of direct nitrite addition (Adamsen et al., 2006; Furukawa et al., 1995).
NO inhibits ZnPP synthesis by interacting with the iron-sulfur cluster within FECH, which is a critical enzymatic component responsible for inserting zinc into PPIX. This interaction destabilizes the structure of the enzyme and effectively deactivates its catalytic function (Furukawa et al., 1995). Furthermore, NO reduces PPIX availability, further limiting ZnPP synthesis via enzymatic and non-enzymatic pathways (Wakamatsu et al., 2010).
Innovative nitrite-free curing methods have been developed to counteract the inhibitory effects of nitrite. The use of LAB, including Lactobacillus fermentum, L. lactis, and L. mesenteroides, has demonstrated significant potential for replicating the visual characteristics of nitrosylmyoglobin (Asaduzzaman et al., 2020; Zhang et al., 2007). For instance, L. fermentum enhances ZnPP formation by increasing PPIX availability and facilitating zinc chelation under anaerobic conditions (Asaduzzaman et al., 2020). These findings underscore the potential of LAB as substitutes for nitrites to produce visually appealing and nitrite-free cured meat products.
In conclusion, nitrites and NO inhibit ZnPP formation by reducing PPIX availability and disrupting enzymatic pathways. Nitrite-free curing methods that leverage ZnPP-forming bacteria provide sustainable and health-conscious alternatives for the meat industry. Such methods not only afford the desired coloration of cured products, but also align with the growing consumer demand for clean-label and nitrite-free food options, presenting significant potential for market expansion.
Sodium chloride (NaCl) is a critical factor that influences the formation of ZnPP in nitrite-free meat products. Although moderate salt concentrations enhance ZnPP formation, excessive levels may alter ZnPP synthesis pathways and influence enzymatic activity (Becker et al., 2012; Bou et al., 2020).
Studies have demonstrated that NaCl serves as a catalyst for ZnPP formation by facilitating iron displacement from myoglobin, thereby enabling zinc to bind to PPIX (Becker et al., 2012). In dry-cured ham, moderate salt concentrations have been observed to enhance ZnPP formation by promoting enzymatic reactions and stabilizing ZnPP pigments (Adamsen et al., 2006; Becker et al., 2012). Moreover, research on Parma-like hams indicates that the ZnPP content increases significantly after 40 weeks of processing, with no significant difference between refined salt and sea salt, suggesting that ZnPP formation is primarily influenced by aging duration rather than salt impurities (Wakamatsu et al., 2009).
While moderate salt concentrations facilitate ZnPP formation, excessively high concentrations can inhibit enzymatic activity by reducing water activity and destabilizing ZnPP-related enzymes (Becker et al., 2012). Studies on Serrano ham have demonstrated that ZnPP content is positively correlated with salt content when measured on a dry-weight basis, but inversely correlated when measured on a desalted basis, suggesting that salt content exerts a complex influence on ZnPP formation (Bou et al., 2020). The effect of NaCl on ZnPP is further modulated by pH and enzymatic activity. Research indicates that as the salt concentration increases, the final pH of meat decreases, potentially influencing ZnPP synthesis (Bou et al., 2020).
In addition, ZnPP formation is influenced by microbial activity. Studies have demonstrated that LAB, including Lactobacillus plantarum, L. lactis subsp. cremoris, and Leuconostoc lactis, can sustain ZnPP synthesis even in environments with a 3% salt concentration (Kauser-Ul-Alam et al., 2021). However, at salt concentrations exceeding 5%, ZnPP formation decreased as LAB metabolism and enzymatic activity were inhibited. This observation indicates that while LAB can be beneficial for ZnPP formation, elevated salt concentrations may adversely affect their efficacy (Kauser-Ul-Alam et al., 2021).
The formation of ZnPP in meat products is influenced by the availability of metal ions, particularly Fe and Zn. FECH plays a central role in this process by inserting Fe2+ into PPIX to form heme under normal conditions (Becker et al., 2012; Hunter et al., 2008; Taketani and Tokunaga, 1982). However, under certain conditions, FECH can also remove Fe2+ and insert Zn2+, resulting in ZnPP formation (Becker et al., 2012; Paganelli et al., 2016; Taketani and Tokunaga, 1982).
Although Fe2+ is the preferred substrate for FECH, the enzyme exhibits the capacity to insert Zn2+, Co2+, and other metal ions into PPIX. However, elevated Fe2+ concentrations may inhibit ZnPP formation by competitive binding at the FECH active site (Hunter et al., 2008). The efficacy of metal insertion follows the order of Fe>Zn>Co>Ni, suggesting that Zn2+ may serve as a substitute for Fe2+ under conditions of low Fe availability (Taketani and Tokunaga, 1982).
Zn2+ is essential for ZnPP formation and has been demonstrated to significantly enhance ZnPP synthesis in vitro (Becker et al., 2012; Schivazappa et al., 2024). However, excessive Zn2+ concentration can inhibit FECH activity by binding to an additional inhibitory site on the enzyme (Hunter et al., 2008). Environmental factors such as phosphate concentration can facilitate ZnPP formation by increasing Zn availability in the system (Becker et al., 2012).
ZnPP formation in meat products is regulated by the interplay between Fe and Zn availability, FECH activity, and environmental conditions. Although Fe2+ generally inhibits ZnPP formation by competing with Zn for FECH binding, Zn2+ functions as a crucial substrate for ZnPP synthesis when Fe levels are low. Elucidation of these factors can facilitate optimization of ZnPP formation during meat processing, thereby enhancing product quality.
Applications and Limitations of Zinc Protoporphyrin IX in Meat Products
ZnPP has revolutionized nitrite-free meat processing by functioning as a natural pigment that eliminates the need for synthetic additives. It forms naturally through enzymatic and microbial pathways, providing a vibrant red color in nitrite-free products and addressing consumer demand for clean-label options (Bou et al., 2020; Wakamatsu, 2022). ZnPP mitigates the health risks associated with synthetic nitrites, such as N-nitrosamine formation during cooking, while maintaining color vibrancy and stability during extended storage and processing (Wu et al., 2024). As shown in Table 3, its role is particularly evident in traditional products such as Parma ham, where ZnPP forms under low-oxygen conditions and optimal pH levels without the addition of nitrites or nitrates (De Maere et al., 2016; Wakamatsu, 2022; Wakamatsu et al., 2019). In dry-cured fermented sausages, controlled pH, temperature, and processing time facilitate ZnPP synthesis, resulting in a rich and stable red color (De Maere et al., 2016; De Maere et al., 2018). Similarly, ZnPP-rich pork liver homogenates were effective in preserving the color of nitrite-free liver pâtés even under thermal processing conditions, rendering ZnPP a viable solution for clean-label trends, aligning with consumer preferences for minimally processed natural products (Llauger et al., 2024).
Sample description | Mechanism of formation | Major results and potential applications | Practical challenges | Future research recommendations | References |
---|---|---|---|---|---|
Parma hams | Proteolysis enhances ZnPP formation by increasing zinc availability for insertion into heme structures. | Proteolysis significantly correlates with ZnPP levels, promoting stable red coloration. | Variability in proteolysis can impact consistency in ZnPP formation. | Standardize curing processes to enhance uniform ZnPP production. | Bou et al. (2018) |
Parma hams and pork muscles | ZnPP-Hb complexes form non-enzymatically, driven by hemoglobin dissociation. | ZnPP-Hb complexes dominated in experimental models of Parma ham, enabling natural coloration. | Strict control of pH levels and temperature is required. | Develop scalable protocols to enhance ZnPP-Hb formation for broader applications in meat processing. | Zhai et al. (2022) |
Dry cured hams | ZnPP forms under low temperature curing conditions. | Red color formation was observed at 3°C–4°C, though slower than at warmer conditions | Slower ZnPP formation compared to warm curing processes. | Investigate low-temperature pathways for industrial scalability | Parolari et al. (2016) |
Parma ham, Iberian ham, and nitrite-cured hams | ZnPP forms through enzymatic activity influenced by salt and nitrite levels. | Lower salt content promotes ZnPP formation, while nitrite inhibits it. | Balancing salt levels for optimal ZnPP without compromising product safety. | Develop alternative curing agents, such as natural acids or plant-derived compounds, that simultaneously enhance ZnPP formation and ensure microbial safety. | Adamsen et al. (2006) |
Nitrite-free dry fermented sausages | ZnPP forms at pH>4.9 and increases significantly during long-term drying. | Natural red color achieved without nitrites; optimal results obtained after extended drying periods of up to 177 d. | Drying up to 177 d limits scalability and increases production costs. | Investigate alternative methods to accelerate ZnPP formation for industrial feasibility. | De Maere et al. (2016) |
Cooked hams | ZnPP and protoporphyrin IX (PPIX) form independently and contribute to reddish color in nitrite-free products. | Demonstrated natural red color development with polyphenols achieving comparable coloration to nitrite-treated controls. | Consistency of pigment formation across production batches. | Optimize processing conditions to stabilize ZnPP and PPIX levels for consistent natural color development. | Giménez-Campillo et al. (2022) |
Longissimus muscles | ZnPP forms through Fe-Zn substitution in myoglobin without significant degradation. | Efficient natural red color formation achieved during short-term storage (72 h). | Variability in ZnPP levels under different storage conditions. | Investigate the role of myoglobin availability in ZnPP formation pathways. | Khozroughi et al. (2017) |
Porcine muscles | ZnPP forms at optimal pH 4.75 and 5.5, varying by muscle fiber type. | ZnPP production optimized using slow-twitch (type I) fibers in acidic pH conditions. | pH variability requires precise adjustments for consistency. | Develop methods to enhance consistent ZnPP production across different muscle types. | Wakamatsu et al. (2019) |
Pork homogenates | ZnPP forms through parallel enzymatic and non-enzymatic pathways, inhibited by nitrite. | Highlighted key enzymatic and non-enzymatic pathways for ZnPP formation, favoring nitrite-free processing. | Inhibition by nitrite poses challenges for industrial adaptation. | Identify alternative additives to enhance ZnPP formation while ensuring product safety. | Becker et al. (2012) |
Minced pork muscles | ZnPP formation driven by high-ZnPP forming lactic acid bacteria (LAB) under controlled fermentation. | LAB strains enhanced natural color, serving as a substitute for conventional curing agents such as nitrites. | Optimization required for diverse meat products to ensure consistent color improvement. | Evaluate LAB strains across various meat matrices to enhance scalability of ZnPP-based color enhancement. | Kauser-Ul-Alam et al. (2021) |
Porcine and chicken organs | ZnPP forms through zinc-chelatase activity influenced by organ type and pH conditions. | Porcine liver demonstrated the highest ZnPP formation, while chicken organs showed limited capacity. | Limited ZnPP formation in specific organs such as chicken liver and spleen. | Optimization of pH, temperature, and enzyme activity for broader organ application. | Wakamatsu et al. (2015) |
Ultrasound-treated porcine liver | Ultrasound treatment intensifies ferrochelatase (FECH) extraction, promoting ZnPP formation in liver tissues. | ZnPP production increased by 33% with ultrasound treatment compared to conventional methods. | Precise control over ultrasound intensity is required to avoid enzyme degradation. | Optimize ultrasound parameters for large-scale applications, balancing enzyme stability and process efficiency. | Abril et al. (2021) |
Porcine liver homogenates | ZnPP formation promoted by ascorbic and acetic acids under controlled pH and temperature. | ZnPP levels significantly increased under optimal conditions (pH 4.8, 45°C, 24 h), with microbial safety maintained. | Requires precise pH and temperature control for consistent results. | Scale natural acid-based methods for commercial applications. | Llauger et al. (2023) |
Microbial pathways play a crucial role in ZnPP synthesis, with bacteria such as L. lactis, L. mesenteroides, and Enterococcus faecium facilitating ZnPP production in fermented meat products (Asaduzzaman et al., 2020; Wu et al., 2024). These microorganisms ensure vibrant and stable pigmentation, even after thermal processing, rendering ZnPP suitable for diverse product applications.
Technological advancements have significantly enhanced the efficiency and scalability of ZnPP production. Ultrasound-assisted extraction methods increased the FECH activity by up to 33%, reduced processing times by 50%, and achieved higher ZnPP yields (Abril et al., 2021; Abril et al., 2023b). In addition, moderate drying temperatures ranging from 10°C to 20°C, preserved the enzymatic activity and ensured consistent ZnPP yields during processing, while minimizing degradation (Abril et al., 2022). Such technological innovations render large-scale ZnPP production feasible, affording stable meat coloration and extending the shelf life of the product.
Optimized fermentation techniques utilizing engineered Escherichia coli strains, such as HAEM7, have resulted in production yields exceeding 2.2 g/L, minimizing by-products and reducing costs for industrial applications (Choi et al., 2022). Furthermore, system-level metabolic engineering approaches, including feedback regulation elimination and transporter optimization, have further enhanced ZnPP production efficiency, rendering it a sustainable and cost-effective alternative to synthetic curing agents (Choi and Lee, 2023).
Thus, the integration of ZnPP into meat processing is highly promising. However, substantial challenges and limitations persist (Table 3), including variability in ZnPP formation, technological impediments, and incomplete mechanistic understanding, constraining the widespread industrial application of ZnPP.
A major challenge in ZnPP research is the variability in its formation across different muscle types, which complicates standardization of meat processing. The type of muscle fiber significantly influences ZnPP synthesis, with type I fibers requiring acidic pH (approximately 4.75) and type II fibers requiring near-neutral pH. This variability in optimal conditions poses challenges for achieving consistent coloration across different meat matrices (Wakamatsu et al., 2007; Wakamatsu et al., 2019). Moreover, factors such as heme concentration, myoglobin levels, and the presence of metal ions introduce additional complexity, necessitating tailored optimization for each product type (Bou et al., 2020; De Maere et al., 2016).
The commercial adoption of ZnPP-enhancing methodologies has been constrained by technological and economic barriers. Advanced techniques, such as ultrasound-assisted extraction and controlled fermentation, have demonstrated efficacy in enhancing the ZnPP yields. However, these methods require significant investment in specialized equipment and process optimization, limiting their scalability and cost-effectiveness for industrial use (Abril et al., 2021; Abril et al., 2023a; Abril et al., 2023b). For instance, although ultrasound treatment can increase FECH activity and reduce processing time by up to 50%, its high energy requirements and operational costs prohibit its widespread implementation (Abril et al., 2021). Similarly, fermentation technologies involving engineered bacterial strains require precise environmental control, which further increases production costs (Choi et al., 2022).
The limited understanding of the ZnPP formation mechanisms impedes the development of efficient production methods. Although enzymatic and non-enzymatic pathways are involved, the precise interactions among variables, such as pH, temperature, salt concentration, and oxygen levels, remain underexplored. For example, high salt concentrations can either promote or inhibit ZnPP formation depending on other environmental factors (Adamsen et al., 2006; Parolari et al., 2016). Additionally, nitrite and NO, which are commonly used in traditional meat curing, inhibit ZnPP synthesis by interfering with key enzymatic processes (Wakamatsu et al., 2010). These gaps in understanding necessitate further research to optimize production parameters for consistent and scalable ZnPP yields.
ZnPP has not yet received approval from the Food and Drug Administration (FDA) as a food colorant, largely because of the absence of well-defined safety specifications and regulatory frameworks governing natural colorants, which are not subject to the same certification process as synthetic colorants (Simon et al., 2017). This regulatory gap presents significant challenges to the approval process. Therefore, a comprehensive toxicological assessment of novel natural colorants is imperative (de Mejia et al., 2020).
Consumer acceptance of such additives is influenced by factors such as perceived naturalness, ingredient transparency, and generational differences (Maruyama et al., 2021). Notably, providing information about ingredient sources improves acceptance, whereas explaining functionality alone has limited impact. The increasing demand for natural colorants and clean-labelled food products suggests a potential market for ZnPP (de Mejia et al., 2020; Nieto et al., 2024). However, the utilization of advanced technologies such as metabolic engineering and chemical extraction may elicit concerns among regulatory bodies and consumers regarding the naturalness of ZnPP as an additive. Addressing these concerns through transparent labeling and regulatory guidance could be essential for market acceptance.
The natural formation of ZnPP in certain meat products, such as Parma ham, indicates its potential cost-effectiveness (Wakamatsu, 2022). However, natural colorants generally face challenges related to stability and vibration, which must be addressed to ensure their commercial viability (de Mejia et al., 2020). The successful integration of ZnPP as a food additive requires overcoming regulatory hurdles, addressing consumer perceptions, and improving stability through continued research and effective communication strategies.
To address these challenges, a multidisciplinary approach is essential. Future research should focus on elucidating the detailed mechanisms underlying ZnPP formation, optimizing cost-effective production methods, and addressing regulatory and consumer concerns. By addressing these barriers, ZnPP could emerge as a transformative solution for nitrite-free meat production, offering both industrial and consumer benefits.
Future Directions and Emerging Perspectives in Zinc Protoporphyrin IX Research
Despite advancements in the elucidation of ZnPP formation, significant knowledge gaps persist regarding its biochemical pathways. A critical area of inquiry is the mechanism by which FECH preferentially incorporates zinc into PPIX. Previous studies utilizing model systems have demonstrated that zinc substitution can spontaneously occur under anaerobic conditions during curing (Wakamatsu et al., 2004b; Wakamatsu et al., 2007). Further investigation of the molecular factors and environmental conditions influencing this substitution could lead to enhanced ZnPP yields.
The role of non-enzymatic mechanisms in ZnPP formation, particularly in dry-cured meat products with limited enzymatic activity, warrants further investigation. For instance, storage and processing conditions influence the balance between enzymatic and non-enzymatic pathways. Furthermore, studies have shown that both endogenous enzymes and curing conditions contribute to ZnPP formation (Wakamatsu et al., 2009). Elucidating the interaction between these pathways will be crucial for optimizing ZnPP production across diverse meat processing systems and for understanding the role of heme destabilization during proteolysis.
Additionally, exploring the potential health implications of ZnPP is essential. Unlike synthetic nitrites, ZnPP may help reduce the formation of harmful N-nitrosamines, potentially offering a safer alternative to meat products. Although ZnPP has been shown to contribute to color stability in meat products and may influence oxidative stability, its direct antioxidant properties have not been conclusively established and require further investigation (Wakamatsu et al., 2020). In addition, the long-term health effects of ZnPP remain unclear, necessitating systematic research to confirm its safety and potential benefits.
Finally, expanding ZnPP applications to non-meat products, such as plant-based alternatives, seafood, and dairy products, is a promising research direction. Studies on the stability and behavior of ZnPP within diverse food matrices are critical for developing new markets and broadening their industrial applications.
Innovative technologies for enhancing ZnPP formation present promising approaches to address the current production challenges in meat processing. A primary strategy involves bioengineering, including genetic modifications, to enhance the activity of FECH, the enzyme responsible for inserting zinc into PPIX (Choi and Lee, 2023; Choi et al., 2022). Recent studies have demonstrated that overexpression of FECH and optimization of associated metabolic pathways can significantly increase ZnPP synthesis in engineered E. coli strains (Choi et al., 2022). For instance, reducing the concentration of iron in the culture medium and fine-tuning zinc levels has been shown to favor zinc insertion over iron during ZnPP production (Choi et al., 2022; Wakamatsu et al., 2007).
In addition to bioengineering, microbial solutions offer alternative approaches. Specific bacterial strains, such as L. lactis subsp. cremoris, have demonstrated the ability to convert myoglobin into ZnPP both aerobically and anaerobically, rendering them suitable candidates for nitrite-free meat processing (Kauser-Ul-Alam et al., 2020). These bacterial strains not only enhance the red coloration of meat products but also provide a sustainable and scalable solution for industrial applications.
Optimizing biochemical conditions is another critical factor for improving ZnPP production. Studies have demonstrated that manipulating the Zn2+/Fe2+ ratio in culture media can significantly enhance zinc insertion into PPIX while minimizing competing pathways (Choi et al., 2022; Wakamatsu et al., 2007).
Such advancements in bioengineering and microbial methodologies have the potential to transform ZnPP production, bridging the gap between innovative technologies and the increasing demand of the meat industry for natural nitrite-free solutions.
The increasing demand for natural and clean-label food products has created new market opportunities for ZnPP as a natural meat colorant. Consumers are seeking alternatives to artificial additives and synthetic nitrites due to growing health and environmental concerns (Delgado-Pando et al., 2021; Inguglia et al., 2023). This trend is particularly evident in the processed meat sector, where the demand for nitrite- and nitrate-free products is increasing. ZnPP constitutes a viable alternative, providing vibrant coloration that meets consumer expectations and potentially reduces nitrosamine formation, although further research is necessary to confirm this effect (Wakamatsu, 2022).
ZnPP retains its vibrant red color even under light or heat exposure, rendering it a durable and natural alternative to synthetic additives in meat products (Adamsen et al., 2004; Wakamatsu et al., 2007). Furthermore, high ZnPP-forming food-grade LAB have emerged as promising candidates for nitrite-free meat processing, aligning with consumer demand for clean and sustainable solutions (Kauser-Ul-Alam et al., 2021).
In addition to traditional cured meat products, the food industry and researchers are investigating new natural colorants in emerging categories such as plant-based and alternative foods (Bakhsh et al., 2023; Ryu et al., 2023), and may explore the application of ZnPP. The vibrant red color of ZnPP can effectively replicate the appearance of traditional meat products, rendering it a suitable option for plant-based meat analogs. These factors position ZnPP as a significant innovation in the development of natural meat colorants, addressing consumer preferences for healthier, sustainable, and visually appealing food products.
Conclusion
ZnPP represents a significant advancement in the pursuit of natural alternatives to synthetic nitrites for the meat industry. This review highlights the complex mechanisms of ZnPP formation, including endogenous enzymatic, non-enzymatic, and bacterial enzymatic pathways. The formation of ZnPP is influenced by various factors, such as pH, muscle fiber type, processing temperature and time, oxygen levels, and the presence of nitrites. ZnPP offers substantial benefits in nitrite-free meat products by providing a stable red coloration without the associated health risks of N-nitrosamines.
ZnPP aligns with consumer demand for clean-label products, with advanced technologies enhancing its scalability and industrial applications. ZnPP has shown promise in traditional cured meat products, such as Parma ham, with its stability under various processing conditions and potential health benefits, rendering it an attractive option for the meat industry.
Nevertheless, challenges persist in standardizing ZnPP production across diverse muscle types and in optimizing its formation mechanisms. Future research should focus on developing innovative technologies to enhance ZnPP formation, investigating emerging markets for natural meat colorants, and elucidating their mechanisms of action and their potential health benefits.
In conclusion, ZnPP represents a promising natural alternative to synthetic nitrites in the meat industry, with the added benefit of enhancing the color of meat products. As ongoing research addresses current limitations and explores novel applications, ZnPP is positioned to play a substantial role in meeting consumer demands for healthier, more natural meat products, while maintaining the quality and organoleptic properties of traditional cured meat products.