Introduction
Cell-cultivated meat is a novel protein source produced by cultivating animal muscle cells in a laboratory setting using tissue culture techniques (Lee et al., 2022). Cell-cultivated meat aims to address critical issues, such as the climate crisis, animal welfare, food security, as well as health and food safety concerns (Hubalek et al., 2022; Lanzoni et al., 2022; Stout et al., 2022). However, successful introduction of cell-cultivated meat into the market requires overcoming numerous challenges, including scalability, high expense, food safety assurance, biomimicry integration, and establishing production systems that are independent of animal-derived components (Post, 2014; Stout et al., 2022). Specifically, culture media that promote the growth and differentiation of muscle satellite cells (SCs), which are essential for the production of cell-cultivated meat products, need to be developed.
Fetal bovine serum (FBS), which contains over 1,800 proteins, hormones, nutrients, and growth factors (GFs), is a key component of growth medium, and contributes significantly to cultured cell growth (Post et al., 2020). FBS is therefore widely used as a supplement in cell culture media to provide essential nutrients and support the growth and development of various cell types in laboratory settings. However, the use of FBS presents ethical issues related to sourcing from unborn bovine fetuses; issues related to batch-to-batch variability, safety and quality; as well as cost issues, accounting for 55%–95% of the total cost of cell-cultivated meat production. These issues necessitate the development of serum-free alternatives (Caneparo et al., 2022; Hubalek et al., 2022).
Serum-free media, commonly used in stem cell cultures, contain basal salts, amino acids, vitamins, GFs, cytokines, hormones, and energy sources, such as glucose, pyruvate, glutamine, lipids. GFs and cytokines include epidermal growth factor (EGF), fibroblast growth factor 2 (FGF2), and transforming growth factor-beta (TGF-β; Freshney, 2005; Karnieli et al., 2017). Although the use of serum-free media avoids the ethical and consistency issues posed by FBS, similar to FBS, their high cost limit their use. These issues may be overcome by developing targeted culture media by identifying specific serum-free culture medium components that promote the growth of muscle SCs (Kolkmann et al., 2022).
Cell-cultivated meat production uses primary cells, such as muscle SCs, embryonic stem cells (ESCs), and induced pluripotent stem cells (iPSCs). ESCs have the potential to differentiate into various cell types, which can result in unpredictable and uncontrolled growth, and iPSCs have raised concerns regarding genetic modification (Pasitka et al., 2023; Reiss et al., 2021; Shaikh et al., 2021). On the other hand, myogenic SCs are unipotent stem cells with ability to proliferate only to a certain level in vitro, do not differentiate into other cell types, and promote muscle regeneration and muscle fiber formation in vitro (Ding et al., 2021; Reiss et al., 2021). Therefore, SCs are commonly used for cell-cultivated meat production. Despite the diverse origins of muscle SCs, culture media commonly consist of base media such as Ham’s F-10 Nutrient Mix, DMEM/F-12, DMEM, or DMEM/EMEM, supplemented with either FBS or horse serum (Burton et al., 2000; Zygmunt et al., 2023). As of now, a standardized media composition for myoblast proliferation has not been established, with research primarily focusing on differentiation media (Zygmunt et al., 2023).
The purpose of this study was therefore to analyze serum-free media that stimulate muscle SC growth, and identify the key components and signaling pathways responsible for promoting cell proliferation, which may allow development of a growth medium for cell-cultivated meat. The cells used in this study were Hanwoo satellite cells (HWSCs).
Materials and Methods
Cells were collected from the buttocks of Hanwoo steer at 30-month-old Hanwoo and HWSCs were isolated as previously described (Park et al., 2022). Briefly, cells were separated from debris and dead cells by staining with Hoechst 33342 solution (#62249, Thermo Fisher Scientific, Waltham, MA, USA). Then, cells were stained with PE-CyTM7 anti-human CD56 (BD Biosciences, San Jose, CA, USA), FITC anti-sheep CD45 (Bio-Rad, Hercules, CA, USA), APC anti-human CD29 Antibody (Biolegend, London, UK), and FITC anti-sheep CD31 (Bio-Rad) on ice. HWSCs were purified by a SH800S cell sorter (SH800S, Sony, Tokyo, Japan). The final isolated cells are CD45-CD31-CD29+CD56+ cells. These cells were considered passage 0, and only cells from passages 4 to 9 were used in all experiments.
Sorted HWSCs were plated on dishes coated with collagen I (rat tail; Corning, NY, USA) and cultured using Ham’s F-10 nutrient mixture (F10; Gibco, Grand Island, NY, USA) with 20% FBS (Gibco), and 1% penicillin/streptomycin (P/S; Gibco) within a humidified 5% CO2 atmosphere at 37?°C. The medium was replaced with fresh medium every 2 days.
A total of six serum-free media were selected to culture HWSCs (Table 1). Cells in F10 medium with 20% FBS and 1% P/S were seeded onto the collagen-coated plates. The next day, the culture medium was changed to each serum-free medium and cultured for 6 days with the medium changed every 2 days. F10 medium with 20% FBS was used as the control, and F10 medium without FBS was used as a negative control. After completing the proliferation process for 6 days, differentiation was induced by changing to the medium with F10, 2% FBS, and 1% P/S for 4 days.
Cells were seeded at a density of 3,000 cells per well and cultured for 6 days in serum-free medium with or without 1 μM PD173074 (Tocris, Bristol, UK) and in the control medium with or without FGF2 (0–40 ng/mL). Then, HWSC proliferation was measured using the Cell Counting Kit-8 (CCK-8, Dojindo, Kumamoto, Japan). The optical density was measured using a spectrophotometer (SpectraMax M2e, Molecular Devices, Sunnyvale, CA, USA) at an absorbance at 450 nm. The proliferation rate was calculated as follows:
Cells were stained with 0.4% trypan blue solution (Thermo Fisher Scientific) and total viable cell number was measured using a LUNA-IITM automated cell counter (Logos Biosystems, Anyang, Korea). PDL was calculated using the following formula:
Cells were lysed in RIPA lysis buffer (Biosesang, Seongnam, Korea). Protein samples were separated by SDS-PAGE and transferred to polyvinylidene fluoride membranes (PVDF, Bio-Rad). Membranes were blocked in Tris-buffered saline with 0.05% Tween 20 (TBST, Biosesang), incubated with primary antibodies against β-actin (Cell Signaling Technology, Danvers, MA, USA), Desmin (Thermo Fisher Scientific), p-ERK (Cell Signaling Technology), ERK (Cell Signaling Technology), FGF2 (Abcam, Cambridge, UK), and MyoG (Thermo Fisher Scientific), and stained with anti-rabbit IgG, HRP-linked antibody (Cell Signaling Technology) and anti-mouse IgG, HRP-linked antibody (Cell Signaling Technology) as secondary antibodies. The positive protein bands were detected with enhanced chemiluminescence (ECL) solution (DoGen, Seoul, Korea) and visualized using EZ-Capture MG (ATTO, Tokyo, Japan).
Total cellular RNA was isolated from HWSCs using the NucleoSpin RNA Mini kit (MACHEREY-NAGEL, Düren, Germany) and cDNA was synthesized from 500 ng of RNA using a cDNA synthesis kit (Cosmo Genetech, Seoul, Korea) according to the manufacturer’s instructions. qPCR was performed using SYBR Green Master Mix (Toyobo, Osaka, Japan) with primers shown in Table 2 using the QuantStudio 6 real-time PCR system (Applied Biosystems, Foster City, CA, USA). The targeted genes were FGFR1, FRS2, Raf1, ERK 1/2, p38, Pax7, MyoD, and MyoG. GAPDH was used as the housekeeping gene.
qPCR, quantitative polymerase chain reaction; FGFR1, fibroblast growth factor receptor 1; FRS2, factor receptor substrate 2; Raf1, Raf-1 proto-oncogene, serine/threonine kinase; ERK1/2, extracellular signal-regulated kinases 1/2; Pax7, paired box 7; MyoD, myogenic differentiation; MyoG, myogenin; GAPDH, glyceraldehyde-3-phosphate dehydrogenase.
HWSCs were plated on Geltrex-coated coverslips (Thermo Fisher Scientific) at 3,000 cells per well and cultured in each medium. Then, cells were fixed with 4% paraformaldehyde (Biosesang) and blocked with a blocking solution consisting of 0.3% Triton X-100 (Sigma-Aldrich, St. Louis, MO, USA), 2% horse serum (Abcam) and 2% goat serum (Abcam). Cells were stained with primary antibodies against Pax7 (Santa Cruz Biotechnology, Santa Cruz, CA, USA), MyoD (Thermo Fisher Scientific), MyoG (Thermo Fisher Scientific) and Desmin (Thermo Fisher Scientific) for 12 h at 4°C and secondary antibodies Alexa Fluor 555 (Thermo Fisher Scientific) and Alexa Fluor 488 (Thermo Fisher Scientific). The images were visualized using a Zeiss Axio Imager Z2 (Carl Zeiss, Jena, Germany).
Results and Discussion
HWSCs were cultured for 6 days using six different commercial serum-free media. The proliferation rates of control cultured in the control medium were 0.29±0.04, 0.53±0.08, and 1.01±0.04 on days 1, 3, and 6, respectively (Fig. 1A), indicating a gradual increase in cell proliferation over time. In all media, except the negative control F10, the cell proliferation rates increased as the culture period increased. On day 3, improved cell proliferation was seen in four of the screened media compared with cells cultured in the control medium, with normalized cell proliferation rates of 0.7±0.05 for NP, 0.73±0.11 for SF, 0.76±0.08 for CT, and 0.84±0.13 for MS (p<0.001). On day 6, only cells grown in SF and MS exhibited significantly improved cell proliferation compared to cells cultured in the control medium, with normalized cell proliferation rates of 1.77±0.07 for SF and 1.63±0.11 for MS, respectively (p<0.001). Similarly, higher cell densities were observed in SF and MS than in the control medium after 3 and 6 days (Fig. 1B). The total cell number continued to increase over time in all the media, with significant increases in the total cell number observed from day 3 in MS and from day 5 in SF (p<0.001; Fig. 1C). Furthermore, evaluation of PDL showed that the control medium reached 2.61 doublings at day 6, whereas MS and SF achieved 3.55 doublings at day 6 (Fig. 1D). These results indicate that SF and MS have the best proliferation efficiency among the six screened serum-free media and promote HWSC growth more effectively than FBS-supplemented growth media.
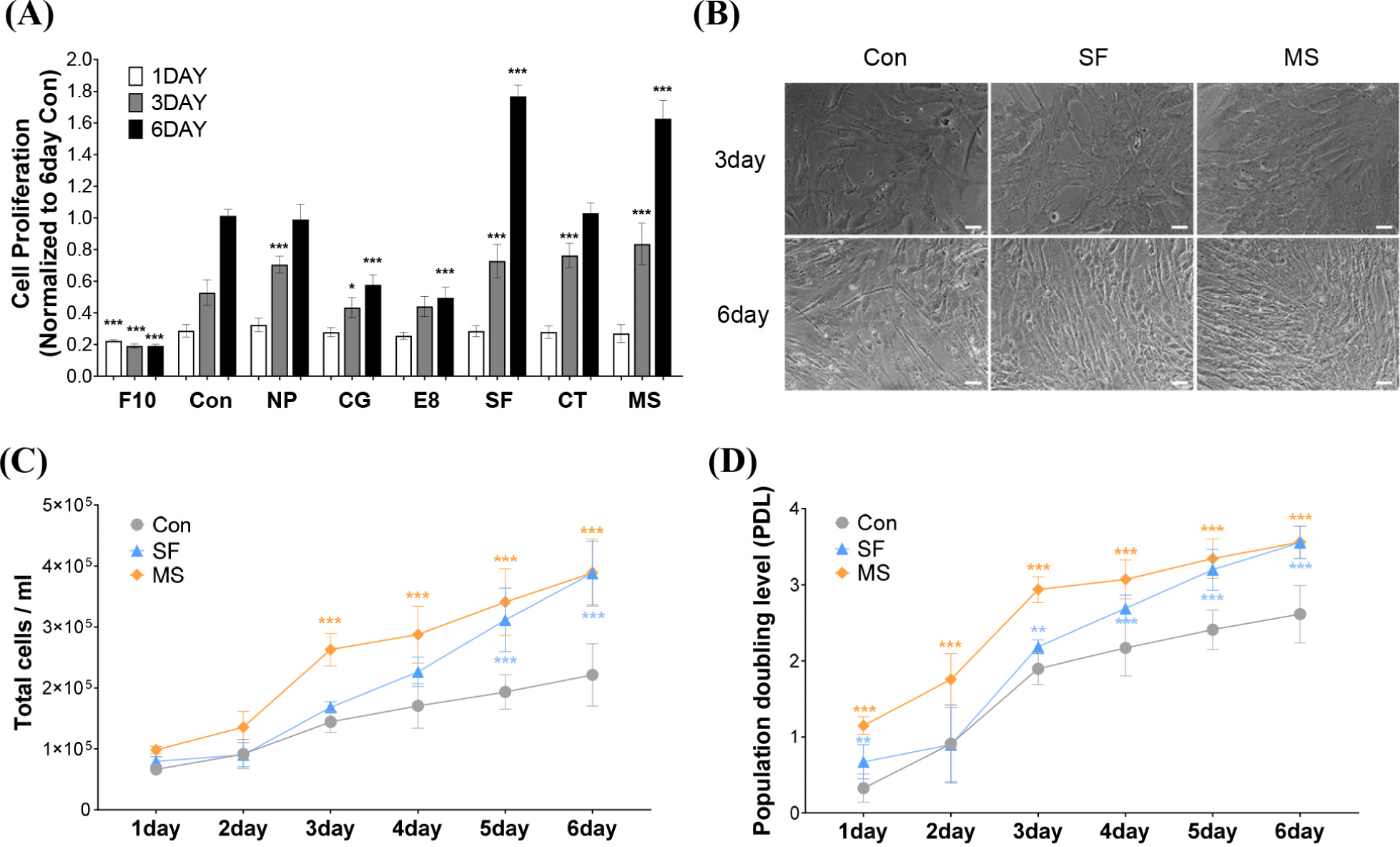
Our results indicated that from day 3, the efficacy of cell proliferation depends on the type of serum-free medium used, which is consistent with results from a previous study. Kolkmann et al. (2020) investigated differences in cell proliferation capacity in various serum-free media. In their study, primary bovine myoblasts were cultured in seven different serum-free media and cell proliferation rates were measured on days 1, 4, and 6. Cell proliferation increased over time in most serum-free media, but significance was only observed on days 4 and 6 in Fibroblast basal medium with the FGM-CD SingleQuots KitTM (FBM), Essential8TM Medium (E8), and TeSR-E8 (Kolkmann et al., 2020). The response tendency to the same medium, E8, was also similar to our results.
The varying cell proliferation rates across different cell culture media can be attributed to differences in media composition. Although the composition of commercially available serum-free media is not disclosed, the components remain constant. Serum-free media contain varying GFs, which are essential for cell growth and maintenance (Choi et al., 2021; Passipieri and Christ, 2016). SCs, responsible for muscle regeneration, are activated, proliferate, and differentiate in response to stimulation by various factors, such as FGFs, TGF-β, Wnt7a, brain-derived neurotrophic factor, and insulin-like growth factor 1 (Hannon et al., 1996; Yeh et al., 2023). FGF2 influences cell proliferation, survival, migration, and differentiation, promoting myoblast proliferation and inhibiting myoblast differentiation (Clegg et al., 1987; Hannon et al., 1996; Sheehan and Allen, 1999). Our western blot results indicated that FGF2 is abundant in SF and MS but was not detected in the control FBS-supplemented medium (Fig. 2A). Proliferating myoblasts express FGF1, FGF2, FGF6, and FGF7, and our results indicated that, unlike in the control medium, HWSCs cultured in SF or MS exhibit high FGF2 expression (Hannon et al., 1996; Yeh et al., 2023). Furthermore, p-ERK, which plays a crucial role in cell survival and proliferation, was exclusively expressed in HWSCs cultured in SF and MS, which suggests that signal transduction pathways related to FGF2 may promote HWSC proliferation (Lavoie et al., 2020; Wang and Rudnicki, 2012).
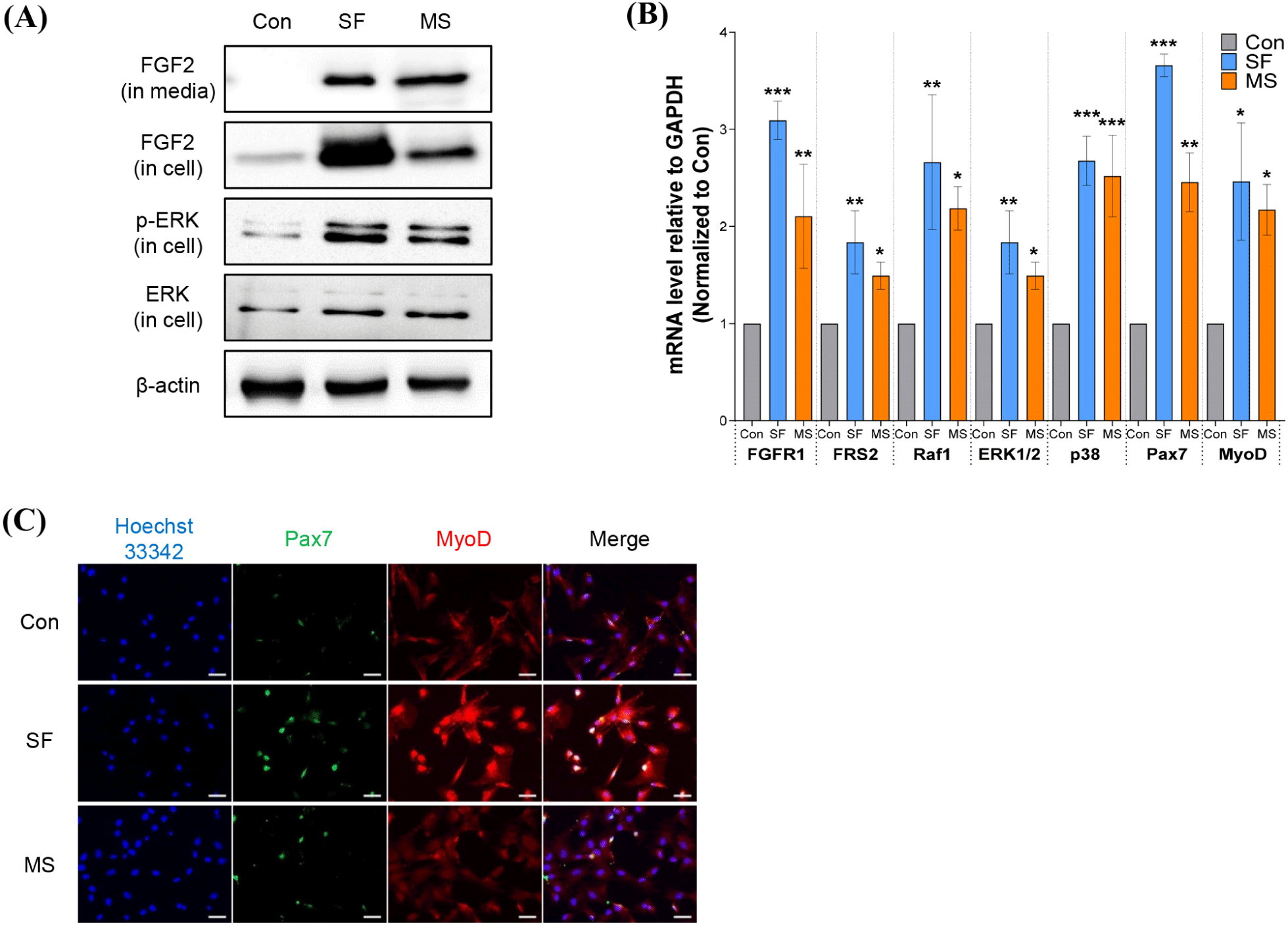
FGF2 binds to FGF receptors (FGFRs), which leads to phosphorylation of tyrosine residues in the intracellular domain of the receptor, which docks with fibroblast growth factor receptor substrate 1 (FRS1) and FRS2, thereby stimulating downstream signaling cascades, including the RAS-MAPK pathway, transcriptional regulation, and etc (Akl et al., 2016). As FGFR1 and FGFR4 are expressed on the cell membrane of SCs (Pawlikowski et al., 2017), we focused on the impact of FGF2 on downstream signaling via stimulation of FGFR1 and FRS2 (Fig. 2B). mRNA expression analysis showed that both FGFR1 and FRS2 were significantly increased in cells grown in SF and MS compared to cells grown in control medium. Expression of biomarkers Raf1, ERK1/2, p38, Pax7, and MyoD, related to sub-signals of FGFR1–FRS2, was also upregulated in HWSCs grown in SF and MS compared to cells grown in control medium (* p<0.05, ** p<0.01, *** p<0.001). ICC revealed high Pax7 and MyoD expression levels in HWSCs cultured in SF and MS (Fig. 2C), which is consistent with the mRNA expression results.
FGF2 activates the RAS–MAPK pathway, which promotes muscle cell proliferation, differentiation, and survival. Two main MAPK families, ERK1/2 and p38 MAPK (α, β, γ, and δ), are involved in myogenesis (Lavoie et al., 2020; Wang and Rudnicki, 2012). Although the Ras/Raf/MEK/ERK and p38α/β MAPK pathways are involved in myogenesis, the role of MAPK signaling cascades is controversial as it is influenced by variations in the source of cell lines used and culture conditions (Gredinger et al., 1998). Activation of the MKK1/2–ERK1/2 pathway stimulates proliferation and myogenic differentiation in C3H10T1/2 and MM14 muscle SC lines (Jones et al., 2001), but suppresses myogenic differentiation in 23A2, L6A1, and C2C12 cells (Wyzykowski et al., 2002). The p38α/β MAPKs are activated in proliferating SCs but is required for late stages of myogenic differentiation in C2C12 and L6 myoblasts (Wu et al., 2000). Our results showed that in proliferating HWSCs, both the Ras/Raf/MEK/ERK and p38α/β MAPK pathways are activated, which suggests that both signaling cascades may promote cell proliferation.
Pax7 and MyoD are representative transcriptional regulators of SC proliferation and differentiation, playing vital roles in regulating myogenesis, muscle development, and muscle regeneration. Quiescent SCs express Pax7, whereas activated SCs co-express Pax7 and MyoD (Wang et al., 2022). Pax7 expression is partially controlled by FGF2 via the FGFR1–ERK signaling pathway (Wei, 2020), whereas MyoD expression requires p38α/β MAPKs (Jones et al., 2005). The elevated ERK1/2 and p38 expression in HWSCs cultured in SF and MS may therefore increase Pax7 and MyoD expression, thereby promoting cell proliferation.
Promotion of cell proliferation by SF and MS is likely to occur via activation of the FGFR1-mediated signaling cascade by FGF2. Although the proliferation-promoting effect of FGF2 has been shown using muscle SCs and muscle cells (Hawke and Garry, 2001), the effect of FGF2 on HWSCs has not been previously reported. To determine whether FGF2 promotes cell proliferation, HWSCs were treated with varying concentrations of FGF2 in the control medium. As shown in Fig. 3A, the HWSC proliferation rate increased in a concentration-dependent manner and was significant at FGF2 concentrations above 2.5 ng/mL, which corresponds to previous a study that found that cow SCs treated with FGF2 concentrations above 2 ng/mL exhibit an increased number of nuclei (*** p<0.001; Skrivergaard et al., 2023).
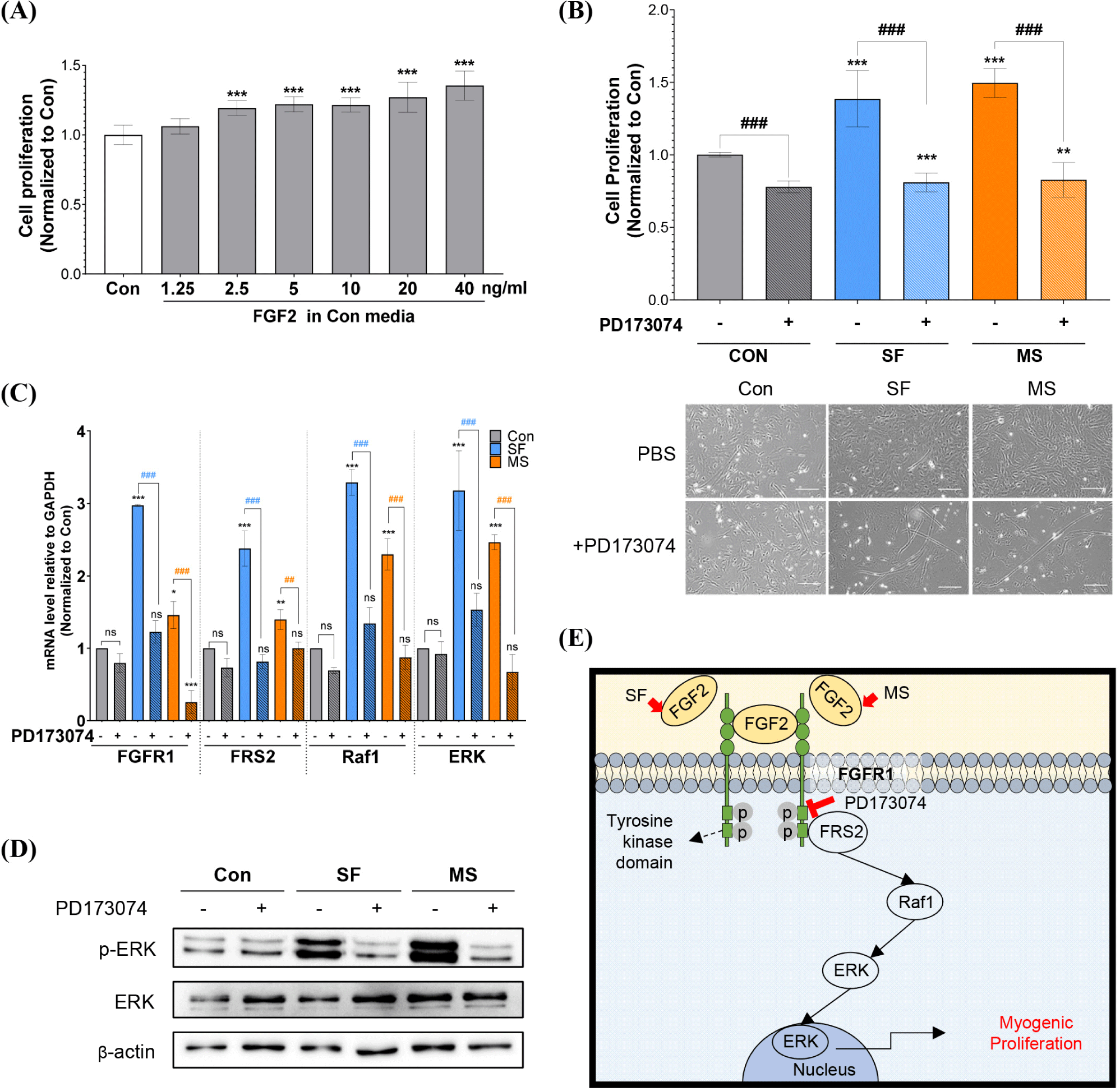
To confirm the cell proliferation effect of FGF2 present in SF and MS, HWSCs were cultured in medium containing 1 μM PD173074, an FGFR1 inhibitor associated with the regulation of SCs and muscle cells (Zhang et al., 2020). As shown in Fig. 3B, PD173074 decreased the normalized proliferation rates of cells grown in the control medium from 1.00±0.01 to 0.77±0.03, and the normalized proliferation rates of cells grown in SF and MS were significantly decreased from 1.39±0.19 to 0.81±0.06 and from 1.51±0.08 to 0.83±0.11, respectively. These results were also confirmed by cell morphology analyses. The effect of PD173074 on the FGFR1-mediated pathway was investigated by evaluating the mRNA expression levels of FGFR1, FRS2, Raf1, and ERK (Fig. 3C). PD173074 did not change the mRNA expression levels of FGFR1, FRS2, Raf1, or ERK in HWSCs grown in the control medium, whereas the mRNA expression levels of FGFR1, FRS2, Raf1, and ERK were significantly downregulated in HWSCs cultured in SF and MS. Consistent with the mRNA expression results, PD173074 decreased the protein level of p-ERK to that of the control group without it (Fig. 3D). These results indicate that FGFR1 inhibition suppresses the enhanced cell proliferation observed in SF and MS by inhibiting the FRS2-Raf1-ERK pathway.
Previous studies showed that PD173074 inhibits the growth of human RD cells isolated from human muscle tissue (Gizaw et al., 2022), and downregulates FGFR1-mediated p38 phosphorylation induced by Lycium barbarum extract in C2C12 cells (Meng et al., 2023). Similar to these studies, our results indicated that PD173074 inhibits HWSC proliferation via FGFR1-dependent signaling, especially the FRS2/Raf1/ERK pathway (Fig. 3E), which suggests that FGF2 may be a key component in SF and MS for enhancing HWSC proliferation. It may therefore be beneficial to develop a proliferation medium containing a substance that stimulates FGFR1.
Culture media developed for cell-cultivated meat production should promote muscle SC proliferation but should not interfere with differentiation or change the cell properties. Although SCs usually differentiate into muscle cells, transdifferentiation may also occur depending on the culture medium composition. To evaluate the risk of transdifferentiation, we therefore cultured HWSCs in the three different proliferation media (control, SF, and MS) for 6 days, followed by 4 days in the same differentiation medium, and investigated changes in morphology and mRNA and protein expression. All HWSCs cultured in the proliferation media were successfully differentiated, and cells cultured in SF and MS displayed a more differentiated phenotype with fused and long tubular shapes (Fig. 4A). Because some condition can induce transdifferentiation, ORO staining indicated that no phenotypic change to adipocyte-like cells occurred in SF and MS, confirming that transdifferentiation did not occur. Furthermore, the mRNA expression levels of the differentiation markers p38, MyoD, and MyoG in HWSCs incubated in SF and MS were significantly upregulated compared to those incubated in the control medium (Fig. 4B). The protein expression levels of Desmin and MyoG were also elevated in SF and MS compared to the control medium (Fig. 4C), and ICC indicated higher expression of MyoD and MyoG in cells grown in SF and MS compared to those in the control medium (Fig. 4D).
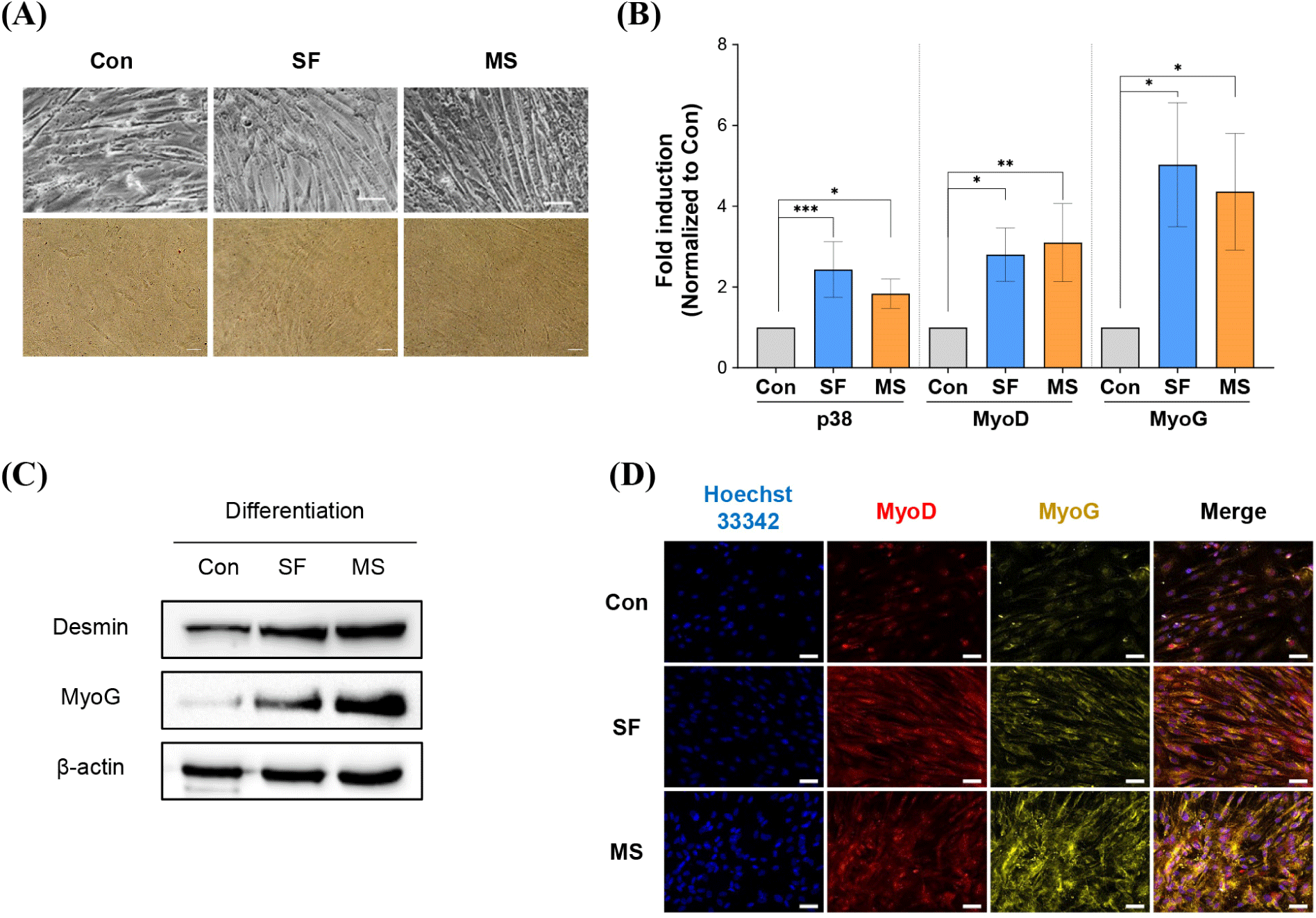
Increased proliferation may affect differentiation capacity, and previous studies have reported that higher initial cell densities of C2C12 cells cultured in serum-free medium lead to dramatically higher cell proliferation and differentiation (Allen et al., 1985). Our results also showed increased differentiation rates and differentiation biomarker expression in cells grown in proliferation-promoting SF and MS. Some substances cause transdifferentiation. Ciglitazone, a potent activator of adipogenesis, switches the differentiation pathway from porcine SCs to adipocytes in vitro (Singh et al., 2007). In addition, MyoD-deficient myoblasts differentiated into brown adipocytes (Wang et al., 2017). However, in the present study, no phenotypic changes were observed in HWSCs upon medium replacement. This suggests that SF and MS media do not contain transdifferentiation inducer, and are therefore suitable for HWSC culture.
Conclusion
Our results suggest that the selected SF and MS media provide an optimal culture environment for HWSCs, promoting both proliferation and differentiation. Furthermore, FGF2 present in SF and MS may be a key factor in promoting proliferation. SF and MS therefore have the potential to improve cell-cultivated meat production by increasing production yield. Further research and optimization of these media may contribute to the development of more sustainable and economic serum-free media for cell-cultivated meat production.