Introduction
Bovine milk contains approximately 2.9%–3.3% proteins, which are divided into two main classes, casein and whey proteins, accounting for 78% and 22% of total milk proteins, respectively (Singh, 2011). There are many types of powdered milk protein products, such as sodium caseinate (Na-CN), calcium caseinate (Ca-CN), milk protein concentrate (MPC), and whey protein concentrate (WPC). MPC is a relatively new dairy product compared to Na-CN and WPC, with an increasing demand in the food industry (Meena et al., 2017; Seo and Yoo, 2021). MPC is obtained from skim milk via ultrafiltration that can further concentrate the milk proteins and remove water, lactose, and mineral salts without any heat treatment. Thus, MPC exhibits high-protein to low-fat/low-sugar and is composed of casein and whey proteins in the same proportion as milk (Corredig et al., 2019; Meena et al., 2021). MPC is used as an ingredient in several dairy products, including processed cheese, yogurt, infant milk formula, ice cream, and dairy beverages, because of the advantages of the addition of milk solids and proteins. In particular, MPC can be used to increase the protein content and improve the texture and stability of yogurts (Agarwal et al., 2015; Uluko et al., 2016). Some researchers have reported that milk proteins can lead to the production of volatile aroma compounds in cultured dairy products, such as yogurt (Barakat et al., 2021; Martin et al., 2011).
Sour cream, also known as fermented or cultured cream, is produced by fermenting pasteurized cream using lactic acid bacteria (LAB) that can produce lactic acid (Shepard et al., 2013). Sour creams can be classified into four groups based on their fat content: Full-fat sour cream (fat ≥18%), light sour cream (fat ≤9%), low-fat sour cream (fat ≤6%), and non-fat sour cream (fat ≤1%) groups. Traditional sour cream is popular in some countries, including Mexico, North America, and Eastern Europe, because of its aromatic taste and creamy texture (Kim et al., 2021; Narvhus et al., 2019). Sour cream can be eaten directly with bread and some cookies and can also be added to various food items, such as stews, sauces, salad dressings, and cakes (Gibson, 2018; Yu et al., 2018). However, sour creams exhibit several quality defects, such as low viscosity and whey separation. To overcome these quality defects, food-grade hydrocolloids or milk proteins were added to the cultured dairy products (Goddik, 2012; Narvhus et al., 2019). Several studies have focused in enhancing aroma characteristics, such as diacetyl in sour creams (Akal and Yetişemiyen, 2016; Kim et al., 2021).
Many studies have reported the effects of milk proteins on the rheological and physicochemical properties of cultured dairy products, such as yogurts (Akalın et al., 2012; Atallah et al., 2020; Barakat et al., 2021; Damin et al., 2009). Although Akal and Yetişemiyen (2016) reported the physicochemical properties of fermented creams containing different levels of skim milk powder and demineralized whey powder, little information is available on their rheological, physicochemical, and aroma characteristics. In particular, the specific effects of MPC on sour cream and its underlying mechanism remain unknown. Therefore, in this study, we determined the effects of MPC on the rheological, physicochemical, microbiological, and aroma characteristics of sour creams.
Materials and Methods
MPC (81% protein, 6.5% lactose, 0.7% fat, and 6.7% ash; Ledor MI 85T) was supplied by HOCHDORF Swiss Nutrition (Hochdorf, Switzerland). To prepare the sour cream samples, dairy cream and whole milk were provided by Seoul Dairy Cooperative (Ansan, Korea), and they contain 38% and 4% fat, 2% and 3% protein, and 2.5% and 5% lactose, respectively. The starter culture, freeze dried-direct vat set (FD-DVS) XPL-1 (Chr. Hansen, Hoersholm, Denmark) were used for the fermentation of traditional sour creams, and its microbial composition was Lactococcus lactis subsp. cremoris, Lactococcus lactis subsp. lactis, Lactococcus lactis subsp. lactis biovar. diacetylactis, Streptococcus thermophiles, and Leuconostoc. For microbiological analysis, potato dextrose agar (PDA), deoxycholate lactose agar (DLA), and de Man, Rogosa and Sharp (MRS) agar were purchased from Difco (Detroit, MI, USA).
Sour cream samples were prepared according to the method of Seo (2022), with some modifications: 50% (w/w) dairy cream, different levels of MPC (0%, 1%, 2%, and 3% w/w), and 47%–50% (w/w) whole milk. Initially, MPC was added to dairy cream and mixed with whole milk using a Eurostar 20 High-speed digital stirrer (IKA-Werke, Staufen, Germany) for 30 min at 75°C. Next, the creams were homogenized using an APV-1000 homogenizer (Invensys APV; Albertslund, Denmark) at a pressure of 50 bar and then heated at 90°C for 15 min. Immediately, they were cooled to 22°C for inoculation with the FD-DVS XPL-1 (0.1 unit/L), subsequently fermented for 20 h at 22°C, and refrigerated at 5°C after fermentation.
Total solid, fat, protein, and ash contents in sour cream samples were analyzed using oven drying, Gerber, micro-Kjeldahl, and furnace ashing methods, respectively, according to the AOAC Official Methods of Analysis (AOAC, 2005). The pH was determined by a pH-meter (Mettler-Toledo, Columbus, OH, USA), and the acidity was analyzed with 0.1 M NaOH using the titration method (AOAC, 2005).
Microbial analysis was carried out to investigate the hygienic properties and viable LAB count in sour creams. Firstly, sour cream sample was serially diluted 10-fold using sterile saline water, and then 1 mL was poured on PDA (yeast and mold), DLA (Escherichia coli), and MRS agar (LAB), and incubated for 5 d at 25°C, 48 h at 37°C, and 48 h at 30°C, respectively. The results are expressed as colony-forming units per gram (CFU/g).
Aroma compounds in sour creams were analyzed, as described by Martin et al. (2011), with some modifications. An Agilent GC-MS system (Agilent 7890B-5977B-GC/MS; Agilent, Santa Clara, CA, USA) equipped with a multipurpose sampler (MPS; Gerstel, Mülheim, Germany) was used to identify the major aromatic compounds (acetaldehyde, diacetyl, and acetoin) in the sour creams. For chromatographic analysis, 5 g of sour cream sample was weighed in headspace vials and diluted with 5 mL of distilled water. Each sample was equilibrated in an MPS agitator for 60 min at 65°C under strong agitation and injected into a CP-Porabond Q column (length: 25 m; internal diameter: 0.32 mm; film thickness: 5 μm) with helium as the carrier gas. The oven temperature was initially held at 40°C for 5 min and then gradually increased to 165°C at a rate of 4°C/min, followed by holding for 5 min. Then, the temperature was raised to 250°C at 5°C/min and finally held for 10 min. Aromatic compounds were identified via selected ion monitoring mode analysis: m/z 29 and 44 for acetaldehyde, m/z 43 and 86 for diacetyl, and m/z 45 and 88 for acetoin. The concentrations of acetaldehyde, diacetyl, and acetoin were quantified using the standard addition method, and a standard curve was prepared with the relative peak area against various concentrations (0, 5, 10, 20, and 50 mg/kg).
Rheological characteristics of the sour cream were evaluated according to the method of Seo (2022) using a HAKKE Roto Visco-1 rheometer (Thermo Fisher Scientific, Waltham, MA, USA). The steady shear rheological behavior was determined using a power-law model:
where σ is the shear stress (Pa), K is the consistency index (Pa sn), (γ) γ˙ is the shear rate (1/s), and n is the flow behavior index. The apparent viscosity (ηa,50) was calculated at 50 1/s.
Dynamic rheological behavior of the sour creams was investigated using small-amplitude oscillatory measurements. The storage modulus (G′), loss modulus (G″), and loss tangent (tan δ=G′/G″) were used to evaluate the dynamic rheological properties.
WHC and syneresis were evaluated by the method of Seo (2022). The sour cream samples were poured into centrifuge bottles and centrifuged for 10 min at 5,000×g. After centrifugation, the separated whey and the drained gels were weighed, respectively. WHC and syneresis were calculated using the following equations:
Results
Chemical compositions of the sour creams supplemented with different MPC levels are detailed in Table 1. The ranges of total solid, fat, protein, and ash contents in the sour cream samples were 28.3%–30.8%, 21.5%–22.3%, 2.74%–5.13%, and 0.56%–0.74%, respectively. These values were similar to the targeted ranges of total solid (27.3%–29.5%), fat (20.9%), and protein (2.5%–4.9%) levels. The total solid, protein, and ash contents increased with an increase in MPC levels because of the relatively high protein expression of MPC. However, the fat content in sour cream with MPC was not significantly different from that in the control sample without MPC.
Viable LAB counts, pH, and acidity of sour cream samples supplemented with different MPC concentrations are listed in Table 2. Contaminant microorganisms, such as E. coli, yeasts, and molds, were not detected in any of the sour cream samples (data not shown), indicating the safety of the sour cream samples analyzed in this study under hygienic conditions. As shown in Table 2, the viable counts of LAB in the sour cream samples ranged from 7.86 to 8.64 Log CFU/g. Supplementation with MPC enhanced the proliferation of LAB, and 3% MPC increased the number of LAB to 8.64 Log cycles. After fermentation, the acidity and pH values of sour cream samples ranged from 0.71% to 0.93% and 4.51 to 4.60, respectively, which further increased with increasing MPC levels.
Effect of different MPC levels on the production of three major aroma compounds by LAB was investigated. Acetaldehyde, diacetyl, and acetoin levels in sour creams with different MPC levels are listed in Table 3. The three aroma compounds were detected in all sour cream samples. As shown in Table 3, the levels of acetaldehyde, diacetyl, and acetoin were in range of 2.09–2.32, 8.51–8.56, and 2.45–2.72 mg/kg, respectively. Diacetyl levels were much higher than those of acetaldehyde and acetoin in all sour cream samples, and the levels of the three aroma compounds in sour cream samples with MPC were similar to those in the control sample without MPC. These results suggest that diacetyl is the major aroma compound in sour cream.
Experimental results of σ and (γ) γ˙ data were well fitted with the power-law model with a high r2 (0.94–0.99), as presented in Table 4. All sour cream samples showed lower flow behavior index (n=0.41–0.50) than 1 in the range of shear rate (0.4–100 1/s), indicating the shear-thinning behavior of sour creams. Apparent viscosity (ηa,50) and consistency index (K) values of the sour creams with MPC were much higher than those of the control sample without MPC, which further increased with an increase in MPC levels (Table 4).
Storage modulus (G′) and loss modulus (G″) of the sour creams supplemented with different MPC levels are shown in Fig. 1. Both the G′ and G″ values increased with an increase in ω, and sour creams with MPC showed higher G′ and G″ values than the control sample without MPC. Moreover, the G′ and G″ values increased with increasing MPC levels (Table 5). This result indicates that supplementation with MPC enhances the viscoelastic properties of sour creams. Tan δ (G′/ G″) values were also used to determine the viscoelastic properties of sour creams. All sour cream samples exhibited lower tan δ (0.44–0.54) values than 1, indicating a higher elastic gel property. In particular, the sour cream sample with 3% MPC exhibited the lowest tan δ value, suggesting that MPC supplementation enhances the viscoelastic characteristics of sour creams.
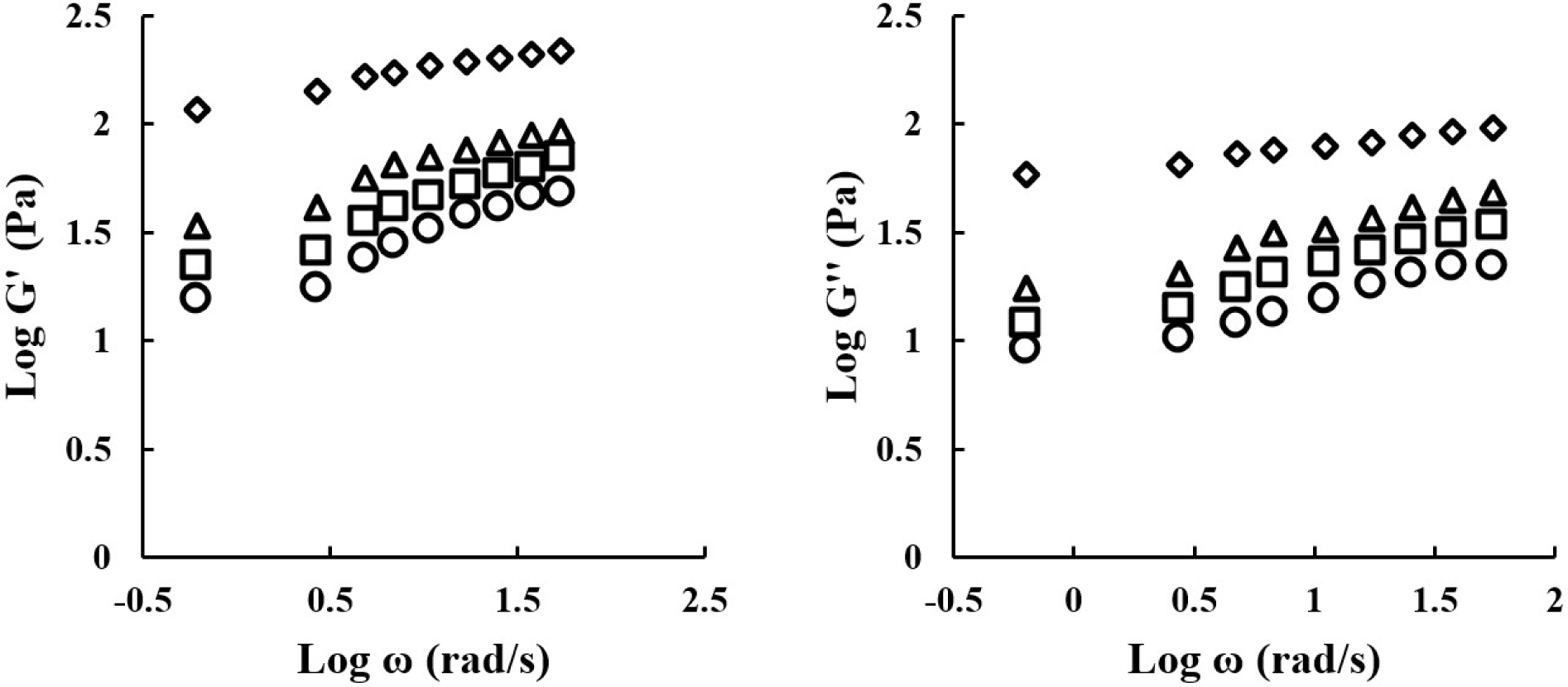
Sample | G′ (Pa) | G″ (Pa) | tan δ |
---|---|---|---|
Control | 27.4±0.99a | 14.7±0.67a | 0.54±0.02b |
MPC 1% | 55.1±1.74b | 29.5±1.85b | 0.54±0.02b |
MPC 2% | 97.2±3.95c | 51.2±1.84c | 0.53±0.04b |
MPC 3% | 183±15.4d | 79.9±5.45d | 0.44±0.01a |
WHC and syneresis are widely used as quality indicators for fermented dairy products, and they are measured by weighing the serum released due to the centrifugal force. As shown in Fig. 2, the WHC of sour creams with MPC was much higher than that of the control sample without MPC, which further increased with increasing MPC levels because of the increase in total solid content. In contrast, syneresis of sour creams was significantly reduced by supplementation with MPC. These results suggest that MPC supplementation improves the textural qualities of sour cream.
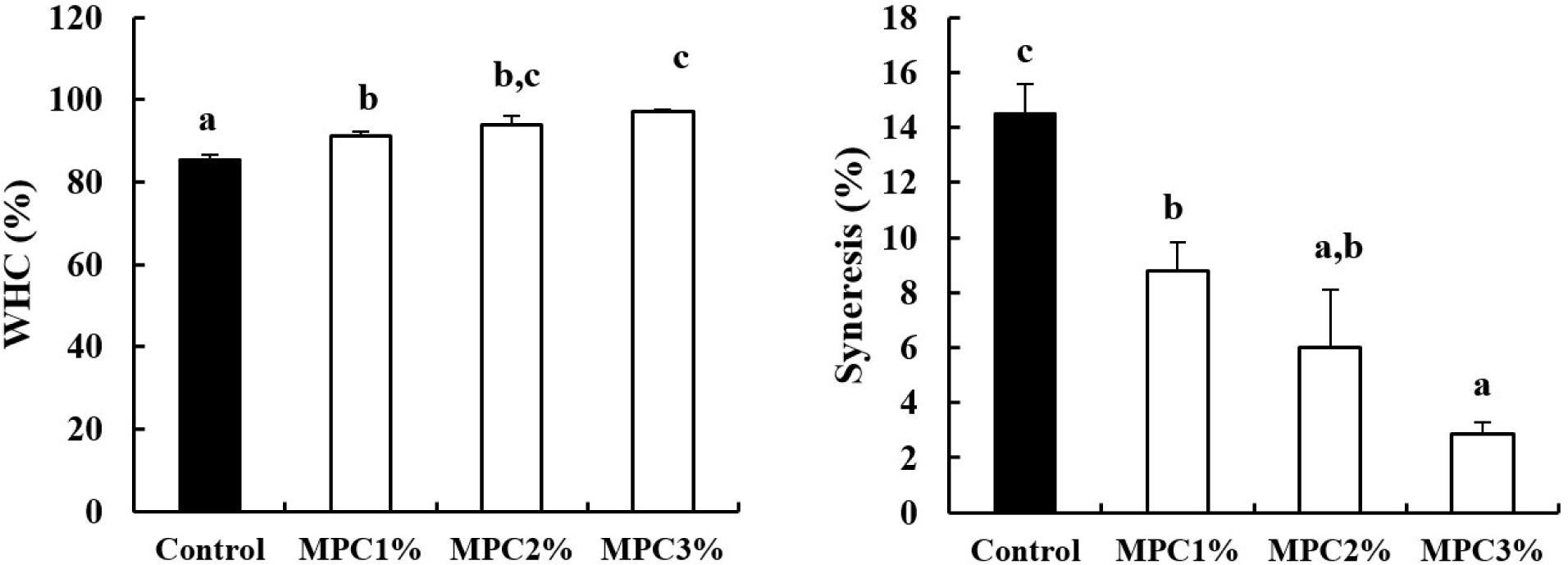
Discussions
In this study, supplementation of sour creams with MPC promoted the growth of LAB from 7.86 to 8.64 Log CFU/g, as shown in Table 2. Several studies have reported the growth-promoting activity of milk proteins on LAB in cultured dairy products. Atallah et al. (2020) reported that the addition of WPC and Ca-CN accelerates LAB growth in low-fat yogurts. Recently, Barakat et al. (2021) also reported that buffalo milk yogurts enriched with WPC and Ca-CN exhibited a higher viable count of Lactobacillus delbrueckii ssp. bulgaricus and S. thermophiles than the control yogurt sample. In general, LAB have complex amino acid requirements, and the proteolytic system of LAB can break down milk proteins into small peptides and amino acids to meet their nutritional requirements (Ardö et al., 2017). According to Zhang et al. (2020), small peptides are the main nitrogen source for LAB, and the growth of Lactobacillus lactis is dependent on these small peptides. In addition, the casein contains essential amino acids with high nutritional value, and two main whey proteins, α-lactalbumin and β-lactoglobulin, act as bifidobacterial growth promoters (Antunes et al., 2005). However, the protein content of milk is too low to increase the growth of LAB in high cell populations. Therefore, supplementation of sour creams with MPC can provide the required nitrogen sources to LAB to stimulate their growth.
In this study, a mesophilic mixed-strain starter culture consisting of Lactococcus lactis subsp. cremoris, Lactococcus lactis subsp. lactis, Lactococcus lactis subsp. lactis biovar. diacetylactis, S. thermophiles, and Leuconostoc were used to produce sour creams. Although their exact composition is unknown, Lactococcus lactis subsp. cremoris and other lactococci may be responsible for most of the production of lactic acid, and Lactococcus lactis subsp. lactis biovar. diacetylactis and Leuconostoc have been reported to produce volatile compounds, such as diacetyl and acetoin, respectively (Narvhus et al., 2019). As the growth of LAB was promoted by MPC supplementation, the acidity of sour creams increased from 0.71% to 0.93% (Table 2). These values were much higher than the U.S. Food and Drug Administration-defined minimum acidity standard (0.5%) for sour cream (Goddik, 2012). The higher acidity of sour creams supplemented with MPC can be explained by the accumulation of lactic acid produced by LAB. Supplementation with MPC may stimulate the growth of LAB and produce more metabolites, such as lactic acid, compared to those in the control sample (de Souza et al., 2021; Shori et al., 2022). In general, pH is inversely proportional to acidity, but the opposite trend was observed in our study. Both the pH value and acidity of sour creams increased with an increase in MPC levels (Table 2). A similar tendency has been reported in buffalo milk yogurts enriched with WPC (Barakat et al., 2021). Frederico et al. (2016) revealed that WPC improved the buffering capacity owing to its high levels of proteins and phosphates, resulting in a slight decrease in the pH value despite the high acidity. Akalın et al. (2012) reported that casein have a higher buffering capacity than whey proteins. Therefore, the high pH values of sour creams supplemented with MPC can be explained by the buffering capacity of MPC.
Aroma compounds are considered to be very important characteristics that determine the quality of cultured dairy products. According to Kaneko et al. (2014), carbonyl compounds (mainly acetaldehyde, diacetyl, and acetoin) are the most potent flavor compounds in cultured dairy products. Acetaldehyde provides fresh and fruity flavors to yogurts. Although acetaldehyde is the main aromatic compound in yogurt products, diacetyl is a more important compound than acetaldehyde because it contributes to a buttery and creamy flavor in sour creams. Acetoin has a mild creamy similar to diacetyl, but its butter-like flavor is considerably weaker than that of diacetyl (Cheng, 2010; Yüksel and Bakırcı, 2015). The results of this study also showed that diacetyl is a major aromatic compound in sour creams (Table 3). In sour cream products, diacetyl is produced by LAB, such as Lactococcus lactis subsp. lactis biovar. diacetylactis and Leuconostoc, via the metabolic pathway of citrate in milk. Citrate is first broken down into oxaloacetic acid and acetic acid, and the former is then decarboxylated to pyruvate. Then, pyruvate is condensed with acetaldehyde-thiamine pyrophosphate to form α-acetolactate, and the unstable α-acetolactate is spontaneously decomposed into diacetyl via oxidative decarboxylation (Martin et al., 2011; Narvhus et al., 2019). Barakat et al. (2021) reported that the enrichment of yogurts with WPC and Ca-CN can lead to the production of volatile aroma compounds due to the proteolytic activities of yogurt starters. However, yogurt was fermented by a mixed starter culture of two LAB, Lactobacillus bulgaricus and Streptococcus thermophiles, and the composition of the yogurt starter culture was different from that of the traditional sour cream starter culture containing multiple strains of Lactococcus lactis subsp. cremoris, Lactococcus lactis subsp. lactis, and Lactococcus lactis subsp. lactis biovar. diacetylactis, and Leuconostoc. Narvhus et al. (2019) revealed that diacetyl production is influenced by the availability of oxygen and redox potentials, and high fermentation temperature (approximately 30°C) can reduce the activity of Leuconostoc strains, thereby decreasing the diacetyl and increasing the acetaldehyde levels. Therefore, aromatic compounds in sour cream products may be influenced by the fermentation conditions and composition of the starter culture rather than the supplementation of milk proteins.
Rheological and textural properties of dairy products are determined by the interactions among major milk components, such as casein, whey protein, and fat globules (Atallah et al., 2020). As shown in Table 3, supplementation with MPC significantly affected the rheological behavior of sour creams in this study. Aggregates of casein micelles can develop with an increase in MPC levels, and these aggregates can enhance the apparent viscosity (ηa,50) and consistency index (K) values (Damin et al., 2009). The shear-thinning behavior of sour creams can be explained by the breaking effect of the clustered fat droplets. In sour cream production, clustered fat droplets are formed after a single homogenization process, which can increase viscosity and affect textural properties of sour creams (Narvhus et al., 2019; Seo et al., 2018). However, these clustered fat droplets can be easily broken at high shear rate and reduce viscosity, resulting in shear-thinning behavior (Bourriot et al., 1999).
With the supplementation of MPC, the storage modulus (G′) and loss modulus (G″) increased to 183 and 79.9 Pa, respectively (Table 5). Moreover, sour cream with 3% MPC exhibited greater elasticity (tan δ=0.44) than the control sample (tan δ=0.54). A similar result was reported by Chen et al. (2018), who found that the gel strength of yogurt gradually increased with an increase in the MPC concentration from 1% to 3%. Gel formation can be attributed to casein aggregation by decreasing the pH and disulfide bonding between caseins and denatured whey proteins (Aryana and Olson, 2017). As described by Tribst et al. (2018), it is important to reach a pH value close to 5.0 for acidification, as acid gel formation begins at this pH value because of the solubilization of colloidal calcium phosphate of casein micelles and the reduction of the electrostatic repulsion between casein aggregates. As the pH further decreased to 4.6, a gel network was finally formed by the hydrophobic interactions of casein aggregates. Thus, MPC supplementation can enhance the gel structure via acid gelation, resulting in an increase in the G′ value and a decrease in the tan δ value (Aryana and Olson, 2017; de Souza et al., 2021; Pang et al., 2015). According to Atallah et al. (2020), heat treatment can also affect the rheological behavior of cultured dairy products. In this study, the homogenized cream was heated at 90°C for 15 min to denature the whey proteins before fermentation. Denatured whey protein can interact with casein proteins, leading to the formation of whey protein-coated casein micelles. These casein-whey complexes can result in the formation of elastic gel with high firmness (Akalın et al., 2012; Aryana and Olson, 2017; Damin et al., 2009). These results indicate that the rheological behavior of sour cream products is significantly affected by protein fortification and heat treatment, and MPC supplementation can enhance the gel structure of sour creams.
WHC and syneresis are important physical properties of sour creams. In this study, syneresis was significantly improved by MPC supplementation due to increased WHC (Fig. 2). Similar results were observed in a previous study by Hashim et al. (2021), who reported that WHC gradually increases with whey protein isolate substitution in non-fat yogurts. Pang et al. (2015) revealed that high WHC can be attributed to high total solid levels. As shown in Table 1, the total solid levels in sour creams were increased by the supplementation of MPC in our study, which is consistent with the WHC values of sour creams with MPC (Fig. 2). The interaction between denatured whey protein and casein protein in MPC also enhances the WHC by forming a gel structure. As described above, whey proteins are denatured by heat treatment and exposed to hydrophobic and sulfhydryl groups, leading to the interaction between denatured whey protein and κ-casein protein via disulfide bonds. This interaction forms a three-dimensional structure that can hold more water molecules (Akalın et al., 2012; Hashim et al., 2021; Li et al., 2021). Consequently, the higher WHC of sour creams supplemented with MPC can be attributed to their higher total solid levels and the three-dimensional structure formed by interactions between the denatured whey protein and casein.
In summary, rheological and textural characteristics of sour cream samples were strongly affected by MPC supplementation, which can contribute to the texture formation of sour cream. Since sour cream products are primarily used as salad dressings and sauces, their moderate viscosity and gel-like structure are very important. In addition, whey separation, a major quality defect in sour cream, was effectively reduced by the increase in WHC with MPC supplementation. Therefore, sour cream with high quality can be produced by MPC supplementation. Moreover, MPC can contribute to the manufacture of high-protein dairy products, and this aspect can also be an advantage in sour cream because protein fortification is one of the recent trends in dairy industry. Our findings suggest that MPC can be used as a supplementary protein to improve the rheological and physical characteristics of sour cream.
Conclusion
Cultured dairy products are supplemented with milk proteins, such as WPC and Na-CN, to enhance their flavor and textural characteristics. Recently, MPC has gained increasing attention because it is a relatively new protein ingredient compared to WPC and Na-CN. Therefore, in this study, we determined the effects of MPC supplementation on the rheological, physicochemical, microbiological, and aroma characteristics of sour creams. Supplementation with MPC promoted the growth of LAB in sour creams, increasing the acidity due to lactic acid production by LAB. Three aroma compounds, acetaldehyde, diacetyl, and acetoin, were detected in all sour cream samples. Additionally, diacetyl was found to be the main aroma compound as its levels were higher than those of acetaldehyde and acetoin in all sour cream samples. The rheological parameters (ηa,50, K, G′, and G″) of sour creams increased with an increase in MPC levels. In particular, the sour cream supplemented with 3% MPC exhibited better elastic properties than the control sample due to the interaction between the denatured whey protein and casein. Moreover, these protein interactions contributed to the formation of a gel network, which increased WHC and improved whey separation. Based on these results, we suggest that MPC can be used as a supplementary protein to improve the rheological and physicochemical characteristics of sour cream in the dairy industry.