Introduction
The practice of hot-boning carcasses arose because it produces meat without the time and expense associated with the completion of rigor during extended refrigeration (Ge et al., 2021; Toohey and Hopkins, 2006). Hot-boned fresh meat is essentially pre-rigor muscle, which has a high pH and water holding capacity, making it potentially more tender and flavorful than chilled meat, and so hot-boning has become a common practice for lamb processing in many Asian countries (Ge et al., 2021; Xiao et al., 2020).
During the early postmortem refrigeration, a series of biochemical and physiological changes occured in the muscle conversion to meat and affects meat quality characteristics (Chauhan and England, 2018; Scheffler and Gerrard, 2007). The rate and extent of pH decline reflects that of glycolysis and adenosine triphosphate (ATP) generation, which was directly related to muscle extensibility and drives the process of rigor-mortis (Ramanathan et al., 2020). In addition, hot-boned meat was easily susceptible to cold shortening because a high concentration of calcium ions (Ca2+) was released from the sarcoplasmic reticulum into sarcoplasm in response to the muscle temperature decreasing rapidly (Li et al., 2012), at a time when intramuscular levels of ATP and pH were still close to physiological levels (Cornforth et al., 1980). The meat toughness and the ability to retain moisture were driven by muscle pH as well as by Ca2+-induced shrinkage of myofibrils during rigor development and cold shortening (Kim et al., 2013; Marsh and Leet, 1996; Paredi et al., 2012). Other processes such as protein oxidation (Bekhit et al., 2013; Kim et al., 2012; Ma et al., 2017) and proteolysis (Dutson et al., 1980; Lewis et al., 1991; Nishimura, 2015; Spanier et al., 1997) also significantly affected the integrity of the myofibril structures and thereby the meat quality (Huff Lonergan et al., 2010).
As Olsson et al. (1994) reported, intervening in the process of rigor-mortis has the potential to be more effective at increasing meat ultimate tenderness than postmortem aging. This was shown by the combination of muscle hot boning and wrapping suggested by Toohey and Hopkins (2006). When hot-boned meat was wrapped tightly by its packaging, it prevented cold shortening in previous studies (Devine et al., 1999; Devine et al., 2014; Rosenvold et al., 2008).
Vacuum packaging (VP) technology allows the packaging film to firmly envelope meat under the effect of negative pressure (Lawrence and Kropf, 2018), having a similar effect to wrapping in preventing cold shortening. Meanwhile, the use of VP technology provided an anaerobic environment for meat, which is generally considered to be an important way of extending shelf life of fresh meat (Ząbek et al., 2021). However, an anaerobic environment simultaneously may reduce the degree of oxidation and play an important role in improving tenderness and reducing water evaporation, which are often overlooked because deoxygenated myoglobin is detrimental to the color of meat at the point of sale (Delles and Xiong, 2014; Maqsood et al., 2016; Ząbek et al., 2021). Thus, we hypothesized that the meat quality of hot-boned lamb during the early postmortem period could be maintained under VP.
At present, there are few studies on the effect of VP on meat quality of hot-boned lamb and the process of glycolysis, protein oxidation, and proteolysis of the early postmortem muscles, especially for those before and after cold shortening, although the technologies of preventing the cold shortening are prevalent. Because the many biochemical reactions of postmortem muscle can alter meat quality during processing and postmortem storage, this warrants exploration. In this study, hot-boned Longissimus thoracis et lumborum (LTL) of lamb was vacuum-packaged in the early postmortem, and the meat quality characteristics including purge loss, pH, muscle temperature, shear force, glycogen and lactate concentrations, free thiol group contents, and the activity of calpain-1 were profiled with lamb wrapped in plastic [aerobic packaging (AP)] as the control. This research provides new insights into pre-rigor lamb and broadens our cognition of the regulation of meat quality with VP technology in hot-boned lamb muscles during postmortem storage.
Materials and Methods
Twelve crossbred Small Tail Han-Mongolian male lambs (average age of 7 mon with hot-carcasses weight of 27.98±2.26 kg) were slaughtered in a random order at a commercial abattoir (Inner Mongolia Mei Yangyang Food, Bayannaoer, China). Both the left and right LTL muscles of the hot carcasses were removed from anterior to posterior within 45 min postmortem. After that, each LTL muscle was trimmed of epimysium and visible external fat and was fabricated into chops (average weight 146.00±16.55 g) with a thickness of 2.54 cm at an ambient temperature of 13°C–15°C, which were stored at 2±2°C with AP until assigned to VP treatments at 1, 6, 12, 24, and 48 h postmortem. The chops from the first three carcasses were considered as the AP control group, which were measured at 1 h as initial values, and then aerobic packaged with O2-permeable polyethylene film (O2 transmission rate 18,500 mL/m2/24 h) at 1 h postmortem for each postmortem storage time (6, 12, 24, 48, 96, and 168 h). The remaining chops were vacuum packaged at different postmortem times with a machine (Ultravac® 2100, UltraSource LLC, Kansas City, MO, USA) in polyamide/ethylene vinyl alcohol copolymer/polyethylene pouches (thickness 150 μm, O2 transmission rate of 4 mL/m2/24 h, water vapor transmission rate of 4.5 g/m2/24 h, Sunrise Material, Jiangsu, China). Specifically, the chops from the next three carcasses were vacuum packaged at 1 h postmortem (VP-1h) for the determination at 6, 12, 24, 48, 96, and 168 h postmortem storage time. Meat chops from another three carcasses were vacuum packaged at 6 h postmortem (VP-6h) with measurement at 12, 24, 48, 96, and 168 h postmortem storage time, and packaged at 48 h postmortem (VP-48h) thereafter measured at 96 and 168 h postmortem storage time. While meat chops from the last three carcasses were vacuum packaged at 12 h postmortem (VP-12h) with measurement at 24, 48, 96, and 168 h postmortem storage time, and packaged at 24 h postmortem (VP-24h) thereafter measured at 48, 96, and 168 h postmortem storage time. During this sample collection, the order of slaughter was random, and n=3 muscle chops were assigned to each postmortem collection time, with each of the 3 chops originating from different carcasses, resulting a total of 78 chops were randomly assigned to the 26 treatment combinations (packaging time×postmortem storage time). All packages were stored and collected for analysis at 2±2°C for the whole of the experiment period.
The purge loss was determined according to the method of Łopacka et al. (2016). The initial weight of each lamb chop from LTL muscle which was fabricated at 1 h postmortem was recorded as m1. After storage for 6, 12, 24, 48, 96, and 168 h postmortem, each chop was removed from the package, blotted dry gently with paper towels, reweighed and was recorded as m2. Then, purge loss was calculated according to the following Eq. (1).
After purge loss measurement, each chop was used for the measurement of muscle pH and temperature. A portable pH meter with a temperature compensated probe (Testo 205, Testo, Lenzkirch, Germany) was used to measure the muscle pH value and temperature simultaneously after calibration with commercial standard buffers of pH 10, 7, and 4. The probe of the pH meter was directly inserted into the center of the meat chop approximately 1.5 cm deep, and the values of pH and temperature were recorded. Three measurements were taken at different insertion sites for each chop and their means were used for statistical analysis.
The Warner-Bratzler shear force was determined according to the method of Hopkins et al. (2010). Each chop was prepared into about 2.0 cm thickness and weighing 65.0±5.0 g with similar shape, sealed in an individual retort pouch without air, and cooked in a preheated 71°C water bath for 35 min. The cooked blocks were cooled in the pouches for 30 min under tap water, then removed from the pouches, blotted dry with paper towels, and transferred to 4°C for storage overnight. The cooked blocks were cut into 1.0 cm×1.0 cm×2.0 cm small cubes along with the muscle fibers direction avoiding thick fat depot and connective tissues, with 6 cubes per cooked meat block. A texture analyzer (TA-XT plus® texture analyzer, Stable Micro Systems, Godalming, UK) was calibrated according to the operating instructions, and then the HDP/WBV probe (Stable Micro Systems) was used to cut the meat cubes perpendicular to the muscle fiber direction at a speed of 1.0 mm/s. The peak force recorded was considered as the shear force and was expressed in Newtons (N). The mean of the peak shear force value of 6 cubes was calculated for each chop and used for statistical analysis.
The concentration of free thiol groups was measured using 5,5′-dithiobis 2-nitro benzoic acid (DTNB) as described by Bao and Ertbjerg (2015). Approximately 0.5 g of frozen meat from each lamb chop were homogenized with 12.5 mL 5% (w/v) sodium dodecyl sulfate in 0.1 M Tris-HCl (pH 8.0) for 30 s on ice. The homogenate was heated for 30 min in an 80°C hot water bath and then filtered through Whatman 40 filter paper (GE Healthcare Life Sciences) after cooling. A 0.5 mL filtrate was mixed with 2 mL of 0.1 M Tris-HCl (pH 8.0) and 0.5 mL 10 mM DTNB in 0.1 M Tris-HCl (pH 8.0) and then incubated for 30 min in a dark room at room temperature. The absorbance was measured at 412 nm and a standard curve was prepared from L-cysteine expressed as nmol/mg protein to quantify the free thiol group, in which the protein concentration of the filtrate was obtained from a standard absorbance curve prepared with 0, 0.025, 0.125, 0.25, 0.50, 0.75, 1.00, 1.50, and 2.00 mg/mL bovine serum albumin in 0.1 M Tris-HCl (pH 8.0). The absorbance values were read at 280 nm.
The activity of calpain-1 was determined by using the casein zymography method according to Du et al. (2017). One gram of frozen meat from each chop was homogenized with 3 mL extraction buffer (100 mM Tris-HCl, 10 mM EDTA, 0.05% β-mercaptoethanol, pH 8.3) in an ice-cold environment and then centrifuged at 10,000×g for 30 min at 4°C. The supernatant was collected, and protein concentration was measured using the bicinchoninic acid assay (Thermo Fisher Scientific, Rockford, IL, USA). Then, the protein supernatant was diluted and mixed with loading buffer [150 mM Tris-HCl (pH 6.8), 20% glycerol, 0.75% β-mercaptoethanol, 0.02% bromophenol blue] in a ratio of 3:2 (v/v) according to the target loading volume and stored at –20°C for further analysis. The casein zymography was performed with 12.5% separation gel [acrylamide/bis-acrylamide ratio=75:1 (w/w), 375 mM Tris-HCl (pH 8.8), 0.05% tetramethylethylenediamine (TEMED; w/v), 0.05% ammonium persulfate (APS; w/v), 2.1 mg/mL casein] and 4% stacking gel [acrylamide/bis-acrylamide ratio=37.5:l (w/w), 125 mM Tris-HCl (pH 6.8), 0.05% TEMED (w/v), 0.05% APS (w/v)], with thickness 0.75 mm. The entire electrophoretic environment was maintained at 4°C. The casein gel was pre-run at 100 V for 15 min in the running buffer (192 mM glycine, 0.05% MCE, 1 mM EDTA, 25 mM Tris-HCl, pH 8.3). The 15 μL sample containing 40 μg proteins was added into each well and the gel was continuously run for 7 h. After electrophoresis, the gel was incubated in the buffer (4 mM CaCl2, 0.05% MCE, 50 mM Tris-HCl, pH 7.5) for 1 h at room temperature with three changes of the incubation buffer, and then was continually incubated for 16 h with the same buffer. Finally, the gel was stained with Coomassie blue (R-250) and de-stained with 20% methanol and 7% acetic acid. The gel was scanned using ChemiDocTM MP System (Bio-rad Laboratories, Hercules, CA, USA) and the image was obtained. The intensity of protein bands was analyzed by Image Lab software (Version 5.1, Bio-rad Laboratories), which relative to that of AP (control) at 1 h postmortem (standard) was used to express the degree of calpain-1 degradation.
After pH measurement, about 15 g of meat was removed, snap frozen in liquid nitrogen, and stored at –80°C for further biochemical analysis. Both glycogen and lactate contents were measured by using commercial analysis kits (A043-1-1 and A019-2, Nanjing Jiancheng Bioengineering Institute, Nanjing, China) and all chemicals were provided by the manufacturer. For the analysis of glycogen content, approximately 70 mg of minced sample meat was flushed with 0.9% physiological saline and blotted dry gently with filter paper, and then was hydrolyzed with 210 μL alkaline hydrolysis in a boiling water bath for 20 min. After dilution of the hydrolysis solution to 5% with ultrapure water, the optical density was measured spectrophotometrically at 620 nm (Beijing Puxi General Instrument, Beijing, China) and calculated according to the operating instructions in the glycogen kit (A043-1-1). For the analysis of lactate content, about 0.5 g of minced meat sample was homogenized in 4.5 mL 0.9% physiological saline for 3×10 s on ice with 30 s intermediate cooling, and centrifuged at 2,500×g for 10 min at 4°C. Then, 0.02 mL of supernatant was incubated with 1 mL enzyme solution and 0.2 mL chromogenic agent of the kit at 37°C water bath for 10 min. After adding 2.0 mL of the termination reaction solution and mixing well, the optical density was measured at 530 nm and the content of lactate was calculated according to the operating instructions in a lactate kit (A019-2).
Data from purge loss, pH, muscle temperature, shear force, glycogen content, lactate content, and free thiol groups were analyzed using General Linear Model procedures to evaluate the main effects of packaging treatment, postmortem storage time, and their interactions. Differences between means were evaluated by least significant differences at the 5% level with significance at p<0.05. The results were analyzed by SPSS statistical software (Version 22.0, SPSS, Chicago, IL, USA) and expressed as the mean value and SD. Correlation coefficients were generated using Pearson’s correlation coefficient analysis and presented as supplementary results.
Results
As shown in Fig. 1A, the pH value of AP chops decreased from 6 to 24 h (p<0.05) and then was stable over postmortem storage time (p>0.05). The pH values of VP-1h chops decreased significantly and reached the minimum at 96 h of postmortem storage (p<0.05), while there were no significant changes after 48 h of postmortem storage for pH values of VP-6h, VP-12h, VP-24h, and VP-48h chops (p>0.05). Among them, the pH values of VP-1h chops were significantly higher at 12 h postmortem than that of VP-6h chops, followed by AP chops (p<0.05). Likewise, the VP-1h chops at 24 h of postmortem storage also had a higher pH value than the chops in the VP-6h and VP-12h groups, followed by AP chops (p<0.05). No significant differences were observed among all treatments at 48 h of postmortem storage (p>0.05). At 168 h, there were no differences in mean pH values between AP, VP-1h, and VP-6h treatments (p>0.05) but were observed higher than those of VP-12h and VP-24h chops (p<0.05), and the mean pH value of the VP-48h chops was not different from that of other treatments (p>0.05).
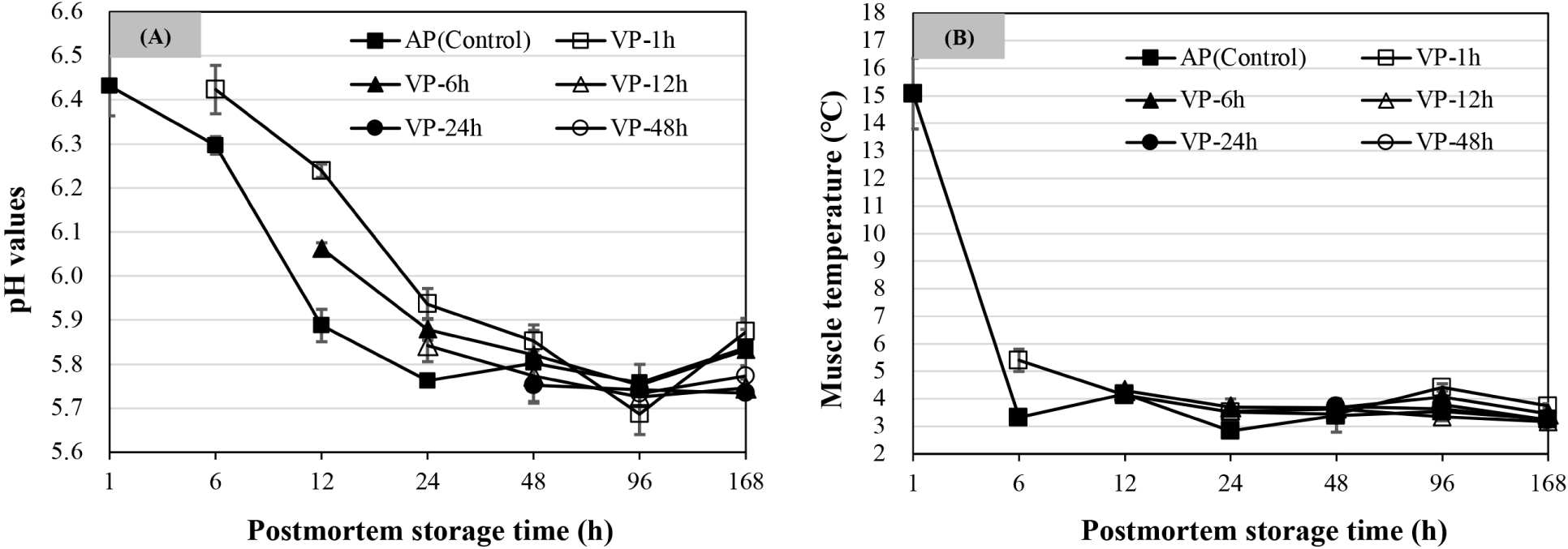
As shown in Fig. 1B, the mean muscle temperature at 6 h of postmortem storage in the VP-1h chops was about 5.40±0.40°C and was significantly higher than that of AP chops (p<0.05). The muscle temperature for each chop was finally maintained at about 3°C–4°C by 12 h of postmortem storage and remained such for the duration of the experiment.
As shown in Fig. 2A, purge loss increased with extended postmortem storage time. The purge loss of AP chops was significantly higher at 168 h of postmortem storage than that of the chops stored from 48 to 96 h, followed by those stored by 24 h postmortem storage (p<0.05). The VP-1h chops at 168 h of postmortem storage had a significantly lower purge loss than any other postmortem storage times (p<0.05), while the purge loss of other VP treatments showed no differences between 96 and 168 h postmortem storage (p>0.05) and was significantly higher than those stored by 48 h postmortem storage (p<0.05). The purge loss of VP-1h chops was lowest at 6, 48, 96, and 168 h of postmortem storage compared to all other treatments (p<0.05). The purge loss of VP-48h chops was significantly higher than that of other treatments at 168 h of postmortem storage (p<0.05).
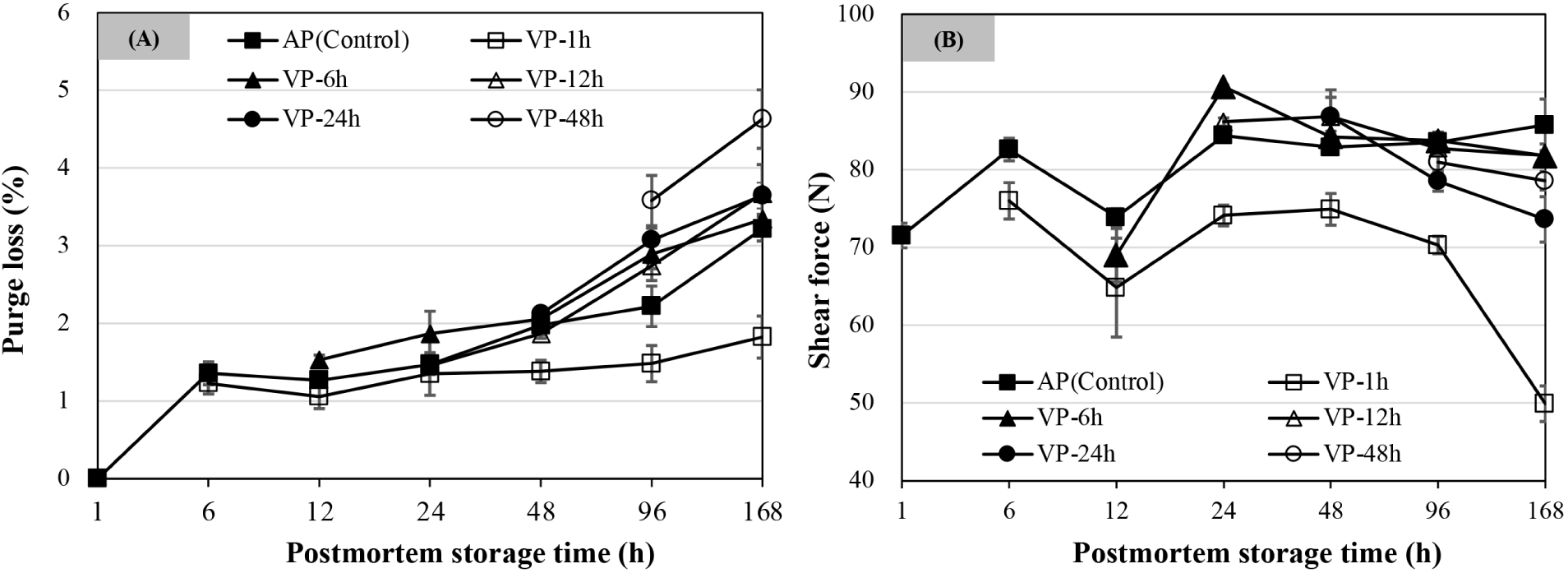
As shown in Fig. 2B, packaging treatment, postmortem storage times, and their interaction had significant effects on shear force of lamb chops. The shear force at 12 h of postmortem storage decreased but increased thereafter and reached a maximum at 24 h of postmortem storage and the value of VP-6h chops was the highest (p<0.05). From 24 h postmortem onwards, the shear force of AP chops had no significant changes over postmortem storage time (p>0.05). The shear force of VP-1h chops showed a lower value than that of other treatments regardless of storage time (p<0.05) and decreased more significantly at 168 h (p<0.05). For the VP-6h and VP-12h chops, no difference for shear force values was observed when compared to the AP group after 48 h of postmortem storage (p>0.05). The shear force values of VP-24h chops showed a decline from 48 to 168 h of postmortem storage and were lower at 168 h than other treatments except that of the VP-1h chops (p<0.05).
As shown in Fig. 3, the free thiol groups of AP chops decreased significantly by 48 h of postmortem storage (p<0.05) and did not change significantly thereafter (p>0.05). The VP-1h had also a significant decrease from 6 to 24 h and at 168 h of postmortem storage (p<0.05). No significant decline in free thiol group was observed when lamb chops were vacuum-packaged at 6, 12, and 24 h postmortem, respectively corresponding to the group of VP-6h, VP-12h, VP-24h (p>0.05), which had higher mean values for this measurement at 12, 24, and 48 h of postmortem storage than AP and VP-1h chops (p<0.05). At 96 h of postmortem storage, the free thiol groups of chops in the AP and VP-1h group were lower than that in the VP-6h and VP-12h chops, and had no differences compared to VP-24h and VP-48h chops. At 168 h postmortem storage, the protein thiol group content of VP-1h chops was lower than that of AP, VP-24h and VP-48h chops, and those of VP-6h and VP-12h chops were the highest (p<0.05).
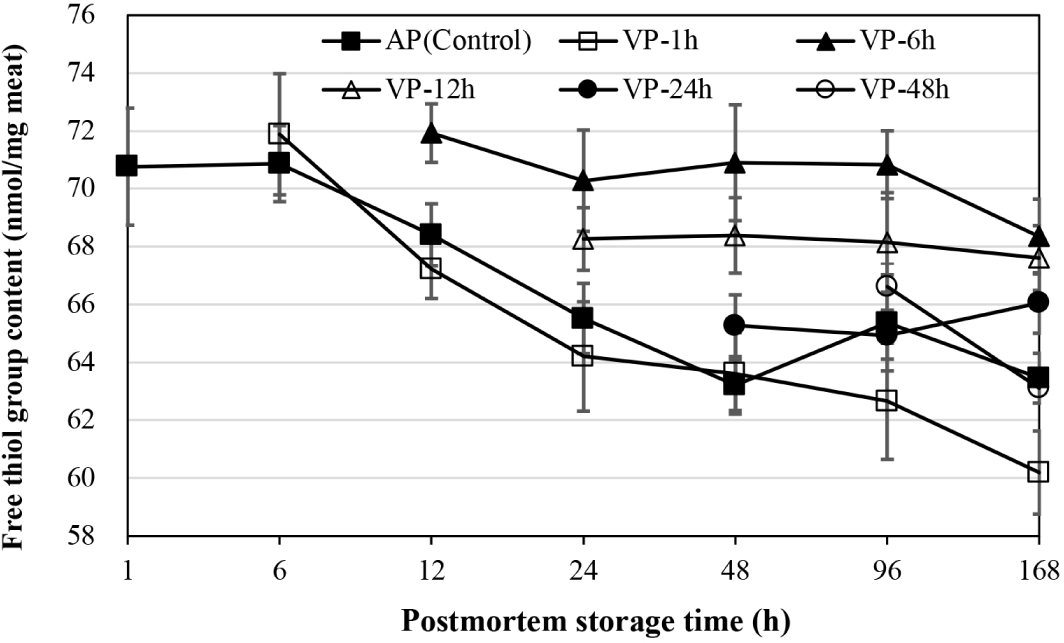
Calpain-1 proteolytic activity (the lightness of degraded casein bands) is shown in Fig. 4A, and the relative degradation rate was calculated based on the activity observed in the AP chops at 1 h postmortem as shown in Fig. 4B. An apparent band was noted at 1 h postmortem on the casein gel. The relative degradation of VP-1h chops was higher than that of AP chops within 12 h of postmortem storage. The bands lightness and the relative degradation of calpain-1 in all treatments decreased with the postmortem storage time extended. The band lightness on casein gel of VP-12h and VP-24h treatments started to decrease after 24 h of postmortem storage accompanied with low values of extracted calpain-1 hydrolytic activity (p<0.05), and a slightly low density was also observed in the VP-48h group at 96 h of postmortem storage compared to that in AP.
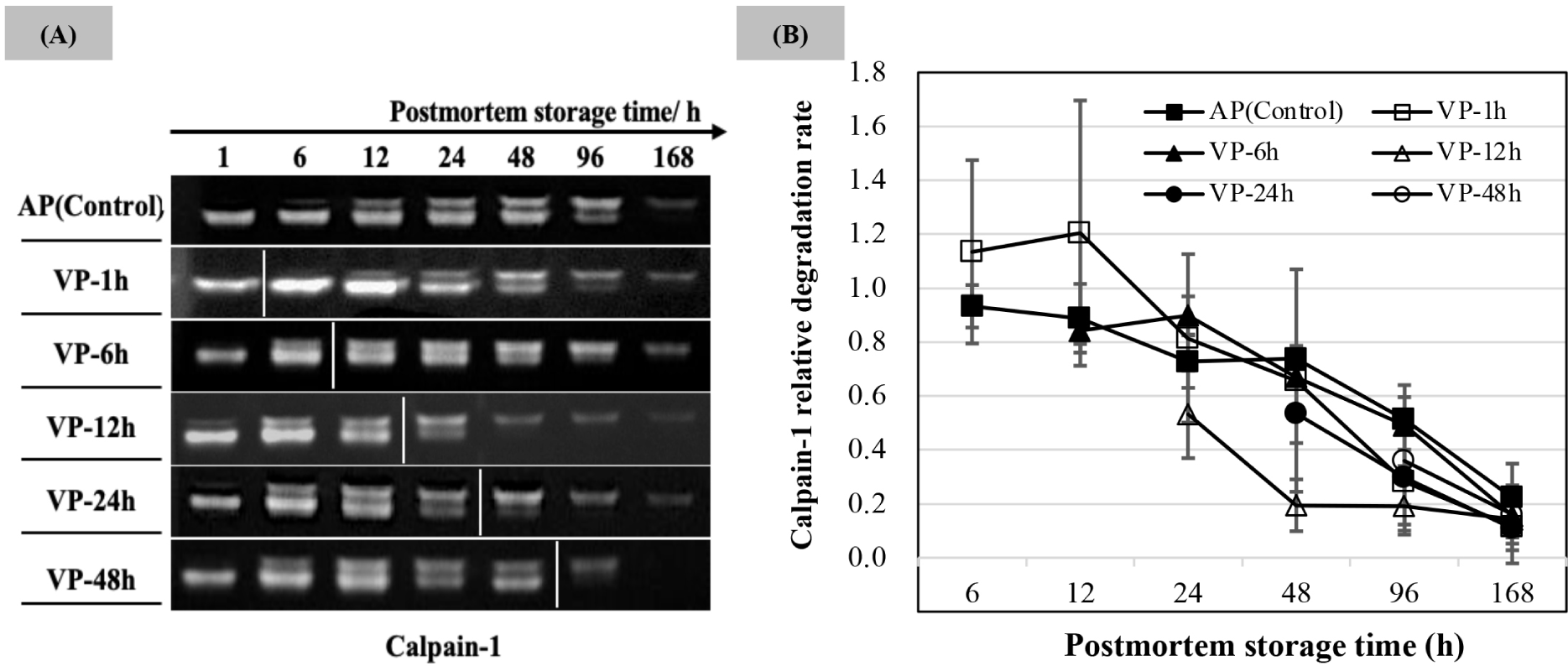
As shown in Fig. 5A, intramuscular glycogen content decreased with postmortem storage time. The glycogen content of AP chops decreased to a minimum level by 24 h of postmortem storage (p<0.05), and did not change significantly at 48, 96, and 168 h of postmortem storage (p>0.05). The VP-1h chops exhibited a continual decrease in glycogen concentration from 6 h to 96 h of postmortem storage (p<0.05) before stabilizing at 168 h (p>0.05). The glycogen content of both VP-6h and VP-12h chops decreased by 48 h of postmortem storage and was stable thereafter with a further decline at 168 h postmortem. There were no significant changes for glycogen content of VP-24h and VP-48h chops after 48 h of postmortem storage (p>0.05). Overall, the glycogen content of VP-1h chops was higher than the other VP-treated groups followed by AP chops within the first 24 h of postmortem storage (p<0.05), while it was non-significant among all treatments at 168 h of postmortem storage (p>0.05).
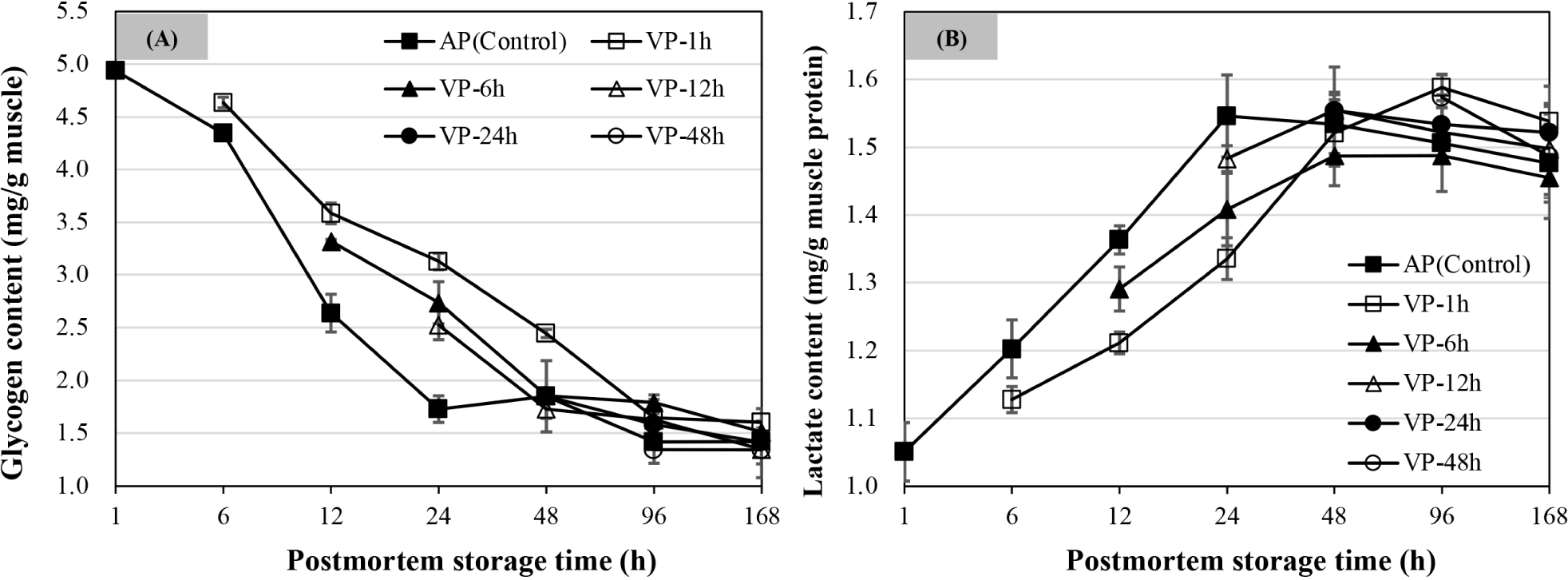
Fig. 5B shows that the lactate contents of lamb chops that were vacuum packaged at different postmortem times compared to the AP (control) group. The lactate contents increased dramatically within the first 24 h of postmortem storage as shown in AP, VP-1h, and VP-6h chops (p<0.05). The lactate content of AP chops reached a maximum at 24 h postmortem. Compared to the AP chops, the lactate contents of VP-1h and VP-6h chops were lower by 24 h of postmortem storage (p<0.05). At 48 h of postmortem storage, no difference was observed for lactate content among all treatments, and the VP-1h chops had a higher lactate content at 96 h of postmortem storage than AP and VP-6h chops (p<0.05), while it was non-significant at 168 h of postmortem storage among all treatments (p>0.05).
Discussion
Cold toughening of hot-boned meat is usually considered to be limited to the phenomenon of cold shortening, although the effects of cold on meat toughness and other quality characteristics may extend beyond the shortening of sarcomeres (Hwang et al., 2004). Cold shortening is likely unavoidable in muscle removed from the carcass pre-rigor, primarily because of the low temperatures this tissue encounters almost immediately after removal. During the experiment period, LTL muscles were fabricated at 13°C–15°C before rigor onset and the average weights of packaged chops were approximately 146.00±16.55 g/each, after which they were stored thereafter in a room chilled at 2±2°C, easily resulting in a rapid chill (Geesink et al., 2000) and a muscle temperature of about 15°C at 1 h postmortem. Unexpectedly, the chops vacuum-packaged at 1 h postmortem had a significantly increased mean temperature compared to all other treatments at 6 h storage, with the mean muscle temperature of VP-1h chops at 5.40°C while that of the AP chops was at 3.32°C, while cold shortening generally occurs if muscle temperature is below 12°C before the intramuscular pH value reached to 6.2 (Chrystall et al., 1980). In this study, the muscle temperature decreased rapidly and was far below 12°C at around 2.3–2.5 h postmortem, at which time the pH value was higher than 6.2 and was outside of the optimum “pH/temperature window” for tenderness development (Hopkins et al., 2014), thereafter likely leading to cold shortening. The VP-1h chops were relatively warmer possibly because of the reduced heat exchanged with the external air environment due to the barrier property of the VP film (Kondjoyan, 2006). From 1 to 6 h postmortem the liner (time, temperature) slope diminished by VP-1h treatment, of which the muscle temperature would be constantly higher than that of AP chops within the first 6 h of postmortem storage if the same initial temperature as AP treatment was postulated.
Purge loss reflects a migration of the mobile water to the meat surface, which increases slowly in the first stage as water exchange between intra- and extra-cellular spaces, and then becomes evident later in the postmortem period (Pearce et al., 2011; Zhu et al., 2017). The purge loss was affected by temperature similar with the findings of Geesink et al. (2000) and Li et al. (2012), where the purge loss increased when conditioning temperature decreased within the range of 0°C–15°C, so a reduced maximum value of purge loss of VP-1h chops within 6 h of postmortem storage was associated with its early warmer temperature. In addition, the early postmortem pH has the potential to be used for predicting the purge loss of samples in VP stored a period (Zhu et al., 2017). Retarding the rate of pH decline benefited water retention as reported in Pearce et al. (2011), and a moderate relationship between pH value and purge loss (r=–0.584, p<0.01; Table 1) was found in this study, thus the delayed pH decline of VP chops might also contribute to the reduced purge loss. During the post-rigor storage, the purge likely collected in the extracellular space and was then released by the compression associated with VP (Huff-Lonergan and Lonergan, 2005), leading to an increased purge loss of VP-48h lamb chops compared to that of AP chops. This aligned with previous findings of Doherty et al. (1996) and Li and Ai (2016), who suggested that VP could result in a higher purge loss than the other packaging methods.
The shear force values of meat generally increase during the rigor-mortis onset and then decrease from 24 to 48 h postmortem, which makes the tenderness of pre- and post- rigor meat greater than that of peri-rigor meat (Devine et al., 2014; Ge et al., 2021). However, a temperature below 10°C–15°C in early postmortem muscle is responsible for the improper functioning of the sarcoplasmic reticulum membranes, leading to the release and accumulation of Ca2+ in the sarcoplasm (Denur, 2021). The most rapid shortening starts with cooling especially during the first 1–2 h postmortem, and then the shortening would slow, with finally the shortening associated with rigor shortening making the most substantial contribution (Locker, 1985). Thus, the decrease in shear force values between 6–12 h postmortem observed most likely indicated ATP remained in early postmortem muscles, allowing the myosin and actin myofilaments to still dissociate and slide past one another (Savell et al., 2005), while the increase in shear force after 12 h was interpreted to signal rigor development. The tenderness would not be improved if cold shortening happened, even after subsequent aging during storage (Simmons et al., 2008). Consistent with this hypothesis, no decrease in shear force for AP chops was observed after reaching a maximum at 24 h of postmortem storage, whereas the decreased shear force values by 168 h of the VP chops suggested that VP was beneficial for meat tenderization. This result agrees with that of Fu et al. (2015), who reported an improved meat tenderness in meat from VP than that from AP that associated with a high degree of proteolysis and enzyme activation and lower oxidation, and the results of the present study further clarified that the positive effect of VP on tenderization is also suitable for cold-shortened muscles. As Hwang et al. (2004) reported, tenderization would be the main factor influencing the toughness of cold shortening meat rather than shortened sarcomere length. Obviously, the shear force of VP-1h chops was the lowest compared to the other treatments over postmortem storage periods. Tightly packaging using VP might make sense because plastic-wrapped has already been shown by previous research to immobilize early postmortem muscle, preventing cold shortening (Devine et al., 2014; Marsh and Leet, 1966; Rosenvold et al., 2008). The relatively higher muscle temperature of VP-1h chops at 6 h postmortem might be another factor in sustaining lower shear force values during storage, as Geesink et al. (2000) and Olsson et al. (1994) reported that the shear force would decrease with increasing temperature within the range of 1°C–15°C. There was an obvious detrimental effect of hot boning at low postmortem storage temperature on the meat quality characteristics of lamb, whereas the early warmer temperature exhibited by lamb chops packaging in VP at 1 h postmortem was associated with a lower mean shear force over postmortem storage and a reduced maximum value of purge loss compared to those without VP before rigor onset.
Protein oxidation and proteolysis are important factors affecting meat tenderness and water holding capacity and are used to characterize the meat during postmortem aging development. The thiol groups are the most reactive among protein residues extensively existed in myofibrillar proteins myosin, actin, and tropomyosin, with the reduction of free thiol groups and cross-link generation implying that protein oxidation occurred (Bao and Ertbjerg, 2018; Fu et al., 2017; Zakrys-Waliwander et al., 2012). The free thiol groups of lamb chops had no obvious decline after vacuum-packaged except for that of the VP-1h chops, and were higher than that of AP chops, illustrating that VP can inhibit protein oxidation due to the anaerobic conditions it creates, which was aligned with previous studies where they found no cross-link protein polymers in VP samples (Lund et al., 2007; Zakrys-Waliwander et al., 2012). Interestingly, VP-1h lamb chops had the lowest free thiol groups similar to that of AP chops throughout postmortem storage when compared to other VP-treatments. The very early postmortem muscle temperature might be another factor that influences protein oxidation in addition to aerobic/anaerobic conditions, as Lu et al. (2020) reported that the protein oxidation is temperature-dependent and accompanied by a greater degree when the temperature is higher. Reid et al. (2017) found that the muscle under anaerobic conditions at 7°C would be more accessible to spoilage-impact than that at 2°C. Moreover, at 1 h postmortem before packaging, the postmortem muscle was in pre-rigor or partially began to shorten with chilling stress and would still be extensible and more likely to have exposed oxidization sites than if in a rigor state (Gross and Lehman, 2013): Therefore vacuum-packaged at 1 h postmortem might promote the reactive oxygen species generation and increased oxidation degree for postmortem lamb. Residual oxygen was not measured in our study, but oxidative stress possibly occurred in VP-1h treatment according to previous research (Bao and Ertbjerg, 2018; Berruga et al., 2005). Unpublished data from our laboratory also demonstrates that oxidative reactions existed in those immediately vacuum packaged postmortem lamb as the a* of VP-1h chops are temporarily increased by 6 h of postmortem storage compared to that packaged in AP. Despite this, the reason for an equal level of thiol groups to be found in VP-1h and AP chops should be further confirmed. Further confounding was that the extent of protein oxidation was not fully reflected in the shear force values, because the final content of thiol groups of VP-1h chops was lower than that of other VP treatments but accompanied by a lower shear force. These results indicate that protein oxidation was not the determining factor for shear force in this experiment.
Calpains are the main enzymes responsible for proteolysis and highly depends on Ca2+ accumulation in the sarcoplasm, as it is under this condition which calpains are activated and autolyzed, and the brighter band on the casein gels indicates the extractable calpain-1 is not autolyzed in native postmortem muscles (Biswas and Tandon, 2019). The extractable calpain-1 activity of lamb chops declined during postmortem storage time illustrating that the autolysis proceeded in all treatments during postmortem storage (Du et al., 2017). The extractable calpain-1 activity was highest in the VP-1h chops by 6 h postmortem storage, reflecting that the calpain-1 in other treatments could be prematurely activated and autolyzed before the muscle starts rigor while that of VP-1h chops was activated later (Hopkins and Thompson, 2001; Hwang and Thompson, 2001). Because the calpain-1 may be activated in the early postmortem as the result of a temperature below 10°C–15°C in the early postmortem muscle, where a temperature below this range would predispose the Ca2+ - Mg2+ ATPase pump in the sarcoplasmic reticulum membranes to inactivate, leading to Ca2+ release and accumulation in the sarcoplasm (Denur, 2021). Considering this, the warmer condition of VP-1h chops may have slowed Ca2+ accumulation thereby potentially influencing the early shortening and shear force. This result complements the reduced shear force observed in the VP-1h lamb chops but this hypothesis was not completely confirmed in the present study as sarcomere length was not assessed. Previous studies widely emphasized the positive regulation of VP on the activation of calpain-1 (dos Santos et al., 2015; Fu et al., 2015), but the difference of extractable calpain-1 activity between treatments in this work was non-significant, only a slight decrease of extractable calpain-1 activity was evident in VP chops by 96 h postmortem. Although replicate numbers were too low to provide a definitive conclusion to the relationship between calpain activity and shear force, the shear force values of other VP muscles did not decrease much below that of the AP group at the 168 h of postmortem storage, suggesting that muscle temperature very early postmortem was more influential than VP technology.
The glycolysis is the principal pathway providing ATP energy to myofilament movement in postmortem muscles (Paredi et al., 2012; Scheffler and Gerrard, 2007). Generally, the pH values and lactate contents of lamb will be stable after 24 h postmortem (Huang et al., 2011; Li et al., 2019), whereas the pH values of VP treatments in this study were obviously higher than that of AP chops in the first 24 h of postmortem storage, and all the treatments approached almost the same values after 48 h postmortem. This indicated that the rate of pH decline decreased when the lamb was vacuum packaged compared to those continually exposed to the air but the degree of pH decline was not affected. Sikes et al. (2017) mentioned that pH values would decline faster when muscle was exposed to temperatures between 10°C and –3°C, whereas there are also several studies documented that decreasing temperature from 20°C to 0°C did not contribute to the rate of pH decline (Li et al., 2012; Liu et al., 2014) or reduced the rate of glycolysis due to a decrease in enzyme activity (Olsson et al., 1994). The relatively high muscle temperature of VP-1h chops by 6 h postmortem might partly contribute to a slower pH decline, whereas the reasons for delayed pH decline in the VP-6h and VP-12h chops remain questionable. Differences in the rate of pH decline have been attributed to modified atmospheres condition as differentially expressed proteins in the glycolytic pathway were reported (Wang et al., 2019; Yang et al., 2016). However, a slightly faster rate of pH decline for muscles in 100% N2 than that in the air was found by Locker and Hagyard (1963), while no differences in pH values of muscle under air and 100% N2 were reported by Buege and Marsh (1975). Again, low sample replication limited the certainty of the effect of VP in this experiment, and the biological differences due to carcasses within each treatment could not be ignored, because anaerobic glycolysis is a complex process that is affected by a variety of factors including glycolytic potential and residual aerobic respiration by mitochondria (England et al., 2018; Scheffler et al., 2015). An area of research that deserves further investigation is the role of early oxygen concentration in postmortem muscles and its effect on early postmortem metabolism. Despite this limitation, this study did suggest the rate of postmortem glycolysis in hot-deboned muscles had a limited effect on shear force, most likely because a rapid pH decline in postmortem muscle is accompanied by increasing in Ca2+ concentration which promotes calpain activation (Liu et al., 2014; Pomponio et al., 2010; Tornberg et al., 2000), whereas the Ca2+ was already massive accumulated under low postmortem temperature for all lamb chops, so that although pH decline faster did not make sense in increasing of Ca2+ concentration thereafter meat tenderness of AP chops.
For hot-boned muscles early postmortem under chilled condition, the sarcoplasmic reticulum was possibly inactivated early with massive Ca2+ accumulation in the sarcoplasm due to a rapid muscle temperature decline. While the early mitochondria might still have a greater capacity for Ca2+ sequestration if pH is above 6.3–6.5 (Ramos et al., 2020), the increased Ca2+ entering mitochondria for buffering promotes the oxidative phosphorylation to generate ATP (Brinkkoetter et al., 2008; Buege and Marsh, 1975). Under this hypothecation, the Ca2+ dependent processes such as pH decline from glycolysis, as well as the activation of calpains could be delayed, and the level of muscle shortening decreased (Cornforth et al., 1980; Sikes et al., 2017), just as shown by the results of the VP-1h chops in this work, which had higher pH values, increased extractable calpain, and lower shear force compared to that of AP chops. The present results have not unequivocally pointed out the critical role for Ca2+, and this warrants further investigation.
Conclusion
VP played a defining role in purge loss, the retention of free thiol groups, and shear force even in an environment where the muscle likely underwent cold shortening. It unexpectedly assisted muscle with retaining warmth for the first 6 h postmortem when used at 1 h postmortem, which contributed to a continuously low shear force and purge loss at the expense of reduced free thiol groups. Therefore, there is great potential in the maintenance of hot-boned meat quality if VP is used strategically as a barrier that counters the cold shortening that may occur in the early postmortem muscles. However, the specific mechanism of the delayed pH decline, possibly correlated with Ca2+ release remains to be discerned. Further investigation is warranted as there is the potential to affect the shear force, purge loss, and calpain activity of lamb chops.