Introduction
Whey is a by-product of the cheese making process, containing a lot of whey proteins such as α-lactalbumin, β-lactoglobulin, immunoglobulin and serum albumin (Chen et al., 2019; Xia et al., 2021). Whey also has the characteristics of high protein efficiency ratio, high biological value and high utilization rate (Da Silva et al., 2017). Whey protein contains higher content of branched-chain amino acids (BCAA) than other protein sources, which is crucial for tissue growth and repair (Kerasioti et al., 2019). Goat whey has remarkable antioxidant property at both the molecular and tissue levels because of its ability to neutralize numerous free radicals (Kerasioti et al., 2014). Compared with the milk from cow, buffalo, sheep and camel, the whey protein from goat milk contains the highest levels of proline (53±1.30 mg/g) and eight essential amino acids (Rafiq et al., 2015). However, 47% of the whey is directly discharged around the world, and approximately 1.3 million tons of goat whey is discarded or used as animal feeds every year, causing serious environmental problems (Garay et al., 2021; Papademas and Kotsaki, 2019). Therefore, the high-value reuse of whey has become an urgent problem to be solved. The value of whey can be enhanced by hydrolysis, a process that can mitigate the sensitivity of whey protein by reducing the size of peptides (Bordenave et al., 2000). In addition, BCAA in whey protein hydrolytes have been shown to be able to protect skin cells from inflammation, and low molecular weight peptides usually show strong antioxidant activity after protein hydrolysis (Da Silva et al., 2017; Ranathunga et al., 2006). Goat whey peptides possess biological properties such as immunomodulation, antimicrobial and antihypertension (Osman et al., 2016). Based on the above, high Fischer’s ratio (F ratio) oligopeptides can be prepared from goat whey to increase the value of goat whey. High F ratio oligopeptides refers to the active peptides with the BCAA to aromatic amino acid (AAA) ratio of over 20, and molecular weight (Mw) of 200–1,000 Da, physiological property of it in relieving phenylketonuria and anti-fatigue have been reported (Li et al., 2019). Up to now, the raw materials for the preparation of high F ratio oligopeptides are mostly plant-derived proteins, such as soy and flaxseed protein (Salma et al., 2022; Yan et al., 2021). For animal-derived proteins, only cow casein was reported in 2004 (Pedroche et al., 2004). As an ideal source both in terms of BCAA content and the activity of goat whey peptides, using whey protein for the preparation of high F ratio oligopeptides has not been reported.
In this study, goat whey was hydrolyzed to obtain high F ratio oligopeptides through two-step hydrolysis of pepsin and flavourzyme. The optimal conditions for the double-enzyme stepwise hydrolysis of whey protein and the conditions for activated carbon to adsorb into AAA were discussed. The Mw distribution of high Fischer’s ratio oligopeptides from goat whey (HFO) was defined, and the antioxidant effects of HFO were evaluated by performing various antioxidant assays after the pre-treatment of HFO both in vivo and in vitro. This study not only expanded the high-value processing and utilization of goat whey, but also was of great significance for the diversification and advanced development as well as application of HFO products.
Materials and Methods
Goat milk samples were collected from healthy adult dairy goats raised in AoTe Goat Farm Qingdao Shandong Province, China, and transported to the laboratory by cold chain. After two 10-minute centrifugation processes at 4°C, 2,149×g, the pH value of goat milk was adjusted to 4.1 with 10% acetic acid. Then milk samples were stored at 4°C for 30 min until casein precipitated, and then centrifuged at 2,149×g for 10 min at 4°C to obtain the supernatant, namely the goat whey. After that, the goat whey was filtered with a dialysis bag of 3,500 Da to remove the small molecules, such as salt and lactose. Then the milk whey was freeze-dried to obtain goat whey powder for the enzymatic hydrolysis in the next step.
The two-step enzymatic reaction was performed in a beaker containing a mixture of 100 mL of distilled water and 5 g of goat whey powder. Pepsin hydrolyses were carried out under different conditions where five values for each indicator of time, temperature, pH, and pepsin were chosen. Then the resulting hydrolysate was further hydrolyzed with flavourzyme under the same varied conditions as pepsin. The details of the conditions were shown in Table 1. An orthogonal L9 (34) test design was used to investigate the optimal hydrolysis condition of each enzyme. Four controllable variables, including time (A), temperature (B), pH (C), and the amount of pepsin added (D) were selected for optimization. And three independent trials were conducted at the optimum of enzymatic treatments. The DH of the hydrolysate was determined with the pH-stat method, which was calculated according to the following Eq. (1) (Mat et al., 2020).
where V is the volume of added HCl (mL), N is the normality of the acid, M is the mass of proteins (g), htot is the total number of peptide bonds in whey proteins (8.8 meqv/g), and αCOOH is the mean degree of dissociation of the carboxylic groups produced, which was calculated using the following Eq. (2).
The activated carbon (200 mesh, powder) was used to adsorb AAA in the hydrolysate solution of goat milk whey (Cermakova et al., 2017). The optimum adsorption condition was explored by evaluating the adsorption of the hydrolysate under varying time, pH, and carbon to liquid ratio conditions, the details of which are shown in Table 2. The F ratio of the hydrolysate was measured according to the method of Li et al. (2019). An orthogonal L9 (33) test design was used to investigate the optimal adsorption condition of active carbon. Three controllable variables, including time (A), pH (B) and carbon to liquid ratio (C), were selected for optimization. And three independent trials were conducted at the optimum of adsorption. The absorbance of the hydrolysate was measured at 280 nm and 220 nm using the ultraviolet spectrophotometer. F ratio was defined according to the following Eq. (3), where OD280 and OD220 refer to the absorbance of the hydrolysate at 280 nm and 220 nm, respectively.
The amount of amino acids was determined with a method modified slightly according to the study of Song et al. (2020). The oligopeptide solution was thoroughly mixed with 6 M HCl, where nitrogen was blown for three times, then the obtained substance was hydrolyzed with acid for 22–24 h at 110°C. After that, the hydrolysate was filtered with a double filter paper and diluted with ultrapure water. The filtrate was then spin-evaporated at 50°C to remove HCl and filtered with a 0.22 μm microfiltration membrane filter. The amino acid content in the oligopeptide was determined with an automatic amino acid analyzer (Hitachi High-Tech Science Corporation, Tokyo, Japan).
The Mw distribution of the oligopeptide was determined by gel permeation chromatography (PL_GPC50, Polymer Laboratories, Church Stretton, UK) with two PL aqua gel-OH mixed 8 μm (Agilent, Santa Clara, CA, USA) columns at 30°C, and R1 detector (Waters Corporation, Milford, MA, USA). The mobile phase was 0.1 M sodium nitrate and 500 ppm sodium azide aqueous solution. PEG/PEO was prepared in the mobile phase as a reference standard for determining Mw distribution of the oligopeptide. The oligopeptide solutions were filtered with a 0.45 μm filter before sampling and analysis. The relative Mw and Mw distribution were calculated with the software according to the peak time of the oligopeptide solution.
The DPPH scavenging activity of the HFO was determined according to the method described by Duan et al. (2014). HFO solution was freeze-dried afterwards to obtain HFO powder. 2 mL solutions with different HFO concentrations were added respectively to 2.0 mL ethanol with DPPH (0.1 mM). The mixture was left in dark for 30 min. The absorbances by the HFO solutions were measured using the spectrophotometer (UV756, Shanghai Yoke Instrument, Shanghai, China) at 517 nm. The DPPH RSA was calculated using the following Eq. (4).
In the formula, A0 is the DPPH absorbance by distilled water, and A is the DPPH absorbance by the HFO solution. Analyses of all samples were run in triplicate and averaged.
The ABTS free radicals assay was conducted according to the method reported by Li et al. (2012). 0.3 mL solutions with different HFO concentrations were added to 1.2 mL of ABTSo+ solution. After incubation for 30 min, the absorbance levels at 734 nm were recorded using the spectrophotometer (UV756, Shanghai Yoke Instrument). An equivalent volume with ascorbic acid concentration was used as the control. The initial absorbance at 734 nm was controlled to 0.70±0.02. The ABTS radical scavenging effect was calculated using the following Eq. (5).
In the formula, A0 is the absorbance at 734 nm with ascorbic acid solution, while A is the absorbance at 734 nm by the HFO solution. Analyses of all samples were run in triplicate and averaged.
The hydroxyl RSA was determined according to the method described by Tang and Huang (2018). 1 mL solutions with different HFO concentrations were put into a test tube and then 1 mL 9×10–3 M FeSO4 solution, 1 mL 9×10–3 M salicylic acid ethanol (70%) solution and 1 mL 9×10–3 M H2O2 were added. After reaction in a water bath for 30 minutes at 37°C, the ultraviolet absorbance of the mixture was measured at 510 nm. Ascorbic acid was used as a control. The scavenging effect of hydroxyl radical was calculated using the following Eq. (6).
In the formula, A0 is the absorbance of the mixture by the ascorbic acid solution, and A is the absorbance of the mixture by the HFO solution. Analyses of all samples were run in triplicate and averaged.
Six-week-old male Kunming mice (20±2 g) from Jinan Pengyue Laboratory Animal Breeding (Jinan, China) were kept in polypropylene cages and were maintained at 25±2°C and 55±10% relative humidity with equal 12-h light/dark cycles. All animals were on a standard laboratory feed and water ad libitum. All procedures were approved by the Animal Welfare Ethics Committee of the Shandong Academy of Sciences.
After adaptive feeding for 7 days, the mice were randomly divided into 5 groups. The grouping and administration are as follows (n=6): normal control (NC; Physiological Saline), model group (MG; Physiological Saline), positive control (PC; 10 mg/mL Silymarin) and two groups treated with HFO at a dosage of 300 mg/mL (HDG, high dose group) and 75 mg/mL (LDG, low dose group), respectively. All groups were administered with 0.2 mL of Physiological Saline, Silymarin or HFO with gastrointestinal administration for 10 consecutive days. Except for the NC group, all other groups were injected intraperitoneally (i.p.) with CCl4 (7 mL/kg BW, 0.2% in peanut oil) 2 hours after the last gastrointestinal administration, and mice in the NC group were injected with an equivalent volume of peanut oil (i.p.). All the mice were sacrificed after fasting and continuous water for 24 h. Blood was taken from the eyeballs and the serum was separated.
The blood was collected and centrifuged at 3,500 r/min for 10 min, then the supernatant was taken. Malondialdehyde (MDA), superoxide dismutase (SOD) and glutathione peroxidase (GSH-Px) levels were measured on an automatic biochemical analyzer (7500, Hitachi, Tokyo, Japan) for the antioxidant activity in vitro on an automatic biochemical analyzer (7500, Hitachi), according to the instructions of assay kits (Nanjing Jiancheng Bioengineering Institute, Nanjing, China).
IBM SPSS Statistics 23 software is used for the analysis of the experimental data by using analysis of variance (ANOVA) and the difference analysis of the significance at the 0.05 level between means were determined by Duncan’s multiple range test. The analysis of K and R (the result of extreme analysis) in orthogonal analysis and all the charts were processed using Office Excel 2019.
Results and Discussion
Taking F ratio and DH as indicators, the hydrolysis conditions such as reaction time, the amount of enzyme, temperature and pH were optimized as illustrated in Fig. 1 (pepsin) and Fig. 2 (flavourzyme). When the pH, temperature and amount of enzyme were constant, DH showed a gradual increase. In contrast, F ratio showed an initial increase followed by a decline with the prolongation of hydrolysis time (Figs. 1A and 2A). However, changes to both F ratio and DH were insignificant. This is because the enzyme is more active at the beginning of hydrolysis, and with the progress of hydrolysis, the amount of enzyme and its combination with substrate diminished. The increase of AAA in hydrolyzed liquid resulted in the drop of F ratio. The DH value increased and reached saturation finally due to the increase in the number of active sites of enzymes in the hydrolysis system, and the F ratio reached its maximum at the addition of 1% (w/w) pepsin (Fig. 1B) and 0.5% (w/w) flavourzyme (Fig. 2B). As seen from Figs. 1C and 2C, both DH and F ratio showed a tendency of increasing first and then decreasing with the rise of temperature. Pepsin had the highest DH and F ratio at 50°C, while flavourzyme showed maximum values at 45°C. When the range of pH change is not large, the activity of enzymes is affected by the dissociation of the relevant chemical groups at the active site (Li et al., 2019). Both the DH and F ratio of pepsin hydrolysis occur at pH 2 (Fig. 1D) and the maximum ratio of flavourzyme DH and F ratio at pH 7 (Fig. 2D). In view of orthogonal analysis (Tables 3 and 4), the range analysis revealed that the influential extent of the four factors to DH for pepsin and flavourzyme were C>B>D>A, and the optimal conditions were A2B2C2D2 and A2B1C2D1, respectively. The DH of pepsin obtained in three parallel experiments under optimal conditions were 15.93%, 15.52%, and 15.77%, with an average of 15.74%, and that of flavourzyme were 46.64%, 49.86%, and 47.53%, with an average of 48.01%. Based on the above results, the enzymatic conditions in this study were selected as follows. Pepsin (1%, w/w) was used to hydrolyze the goat whey at pH 2 and 50°C for 2 h. Afterwards, flavourzyme (0.5%, w/w) was used to hydrolyze the former hydrolysates at pH 7 and 45°C for 3 h.
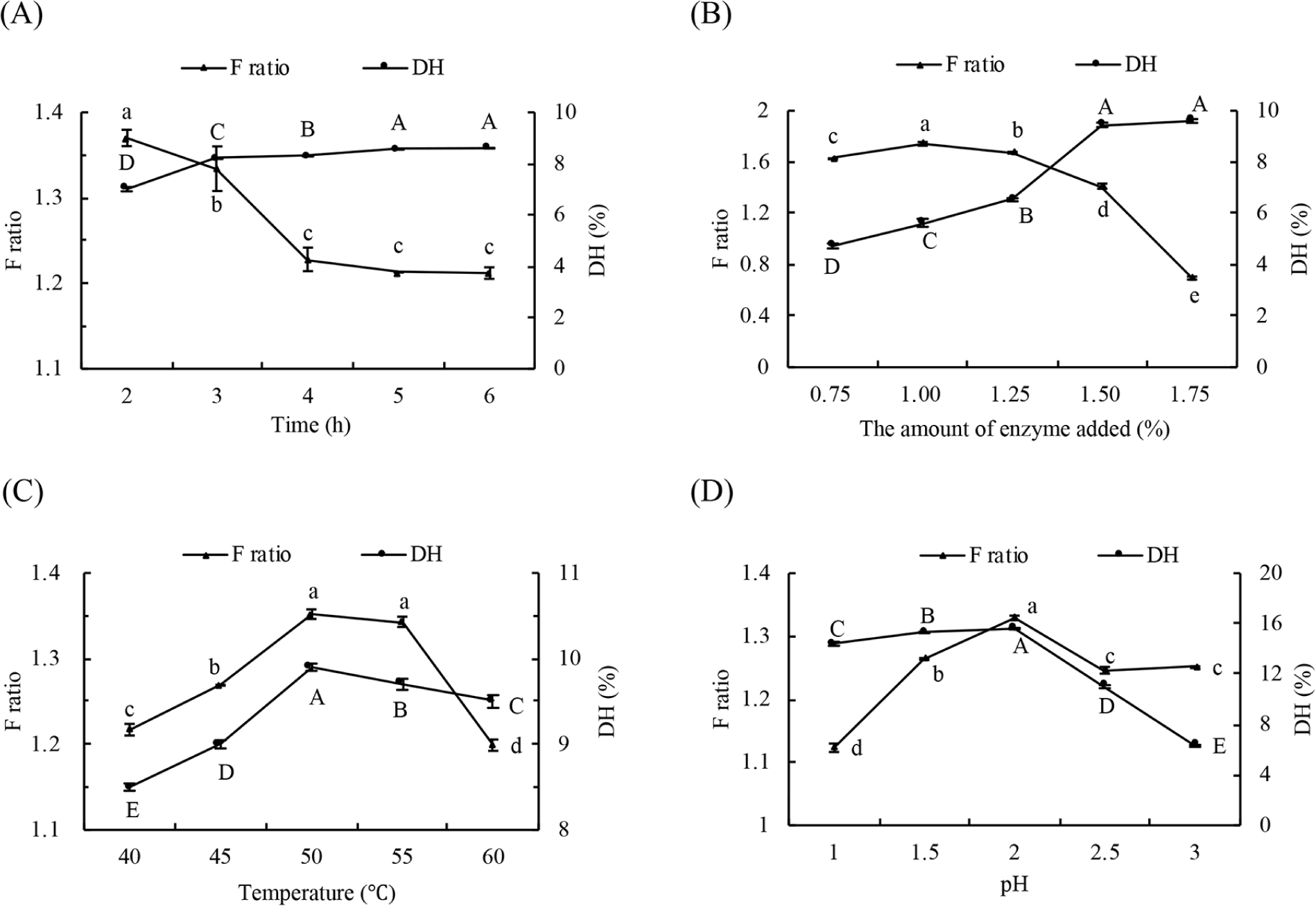
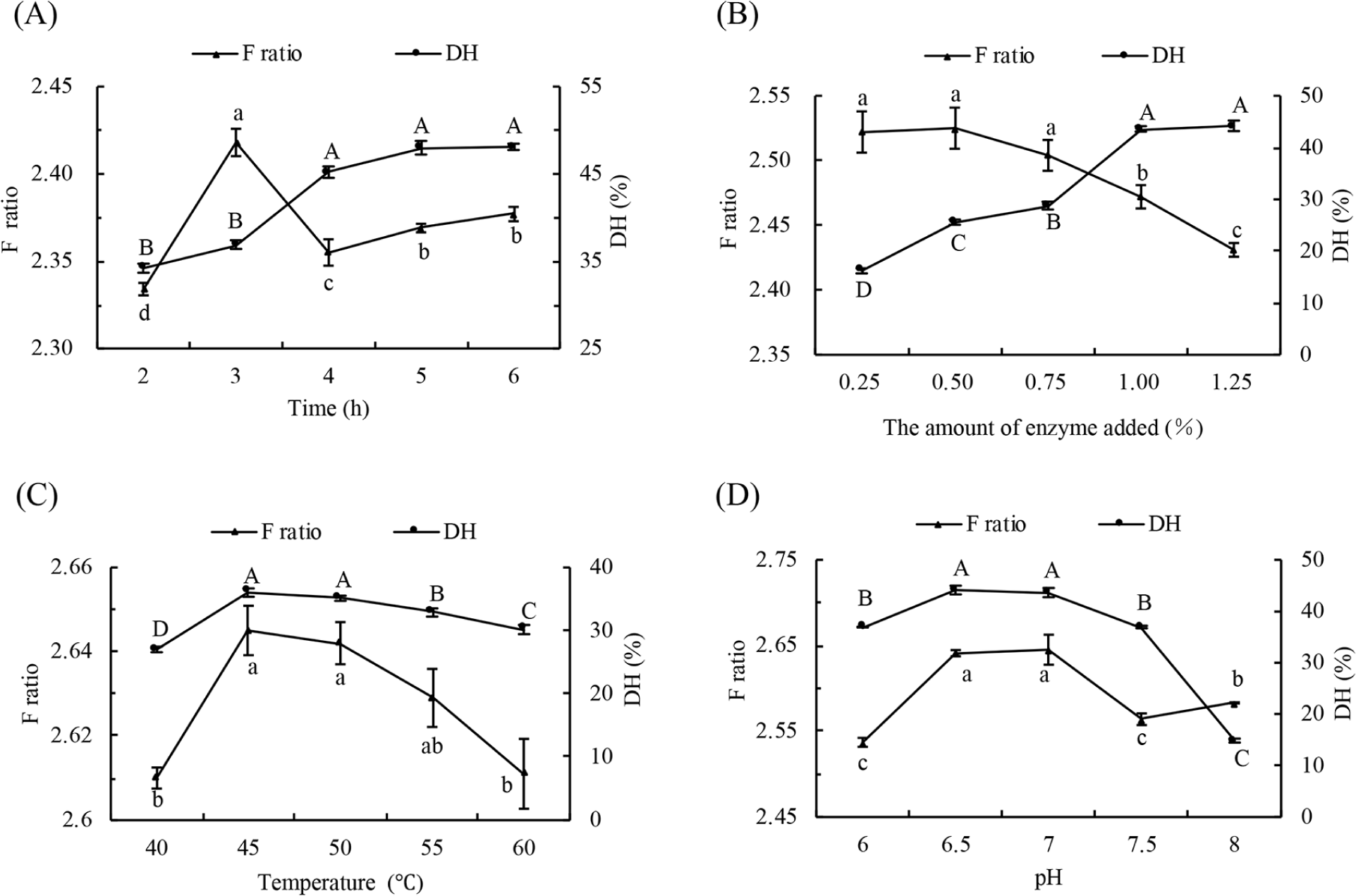
Activated carbon is preferred for AAA adsorption because the benzene ring in AAA is mediated in combination with the graphite microcrystal structure of the activated carbon surface (Majidi and Karami, 2015; Rahmani et al., 2016). As can be seen from Fig. 3A, with the extension of adsorption time, the F ratio of the solution showed the tendency of rises followed by drops, and the F ratio peaked at 2 h of adsorption. The adsorption with activated carbon is better under the acidic conditions, for hydrolytes have a lower solubility at acidic pH, which can facilitate the efficient adsorption of AAA onto activated carbon (Jiang et al., 2017; Li et al., 2019). The results in this study showed that the maximum F ratio was reached at pH 2 (Fig. 3B). The addition of active carbon also affected the F ratio, according to Fig. 3C. The carbon to liquid ratio of 1:5 was shown to be the most ideal. Under the condition of constant pH and duration, the adsorption of active carbons onto AAA may be inadequate when the amount of active carbon is not enough. As the activation of excessive carbon can cause BCAA to be adsorbed, both cases would result in a decrease in F ratio. The results of the orthogonal analysis are shown in Table 5, the range analysis revealed that the influential extent of the three factors to F ratio for active carbon was B>A>C and the optimal conditions were A2B2C2. And the F ratio of the solution obtained from three parallel experiments under optimal conditions were 24.43, 27.35, and 27.18, with an average of 26.32. To sum up, the optimized condition is pH 2 and carbon to liquid ratio of 1:5 for 2 h.
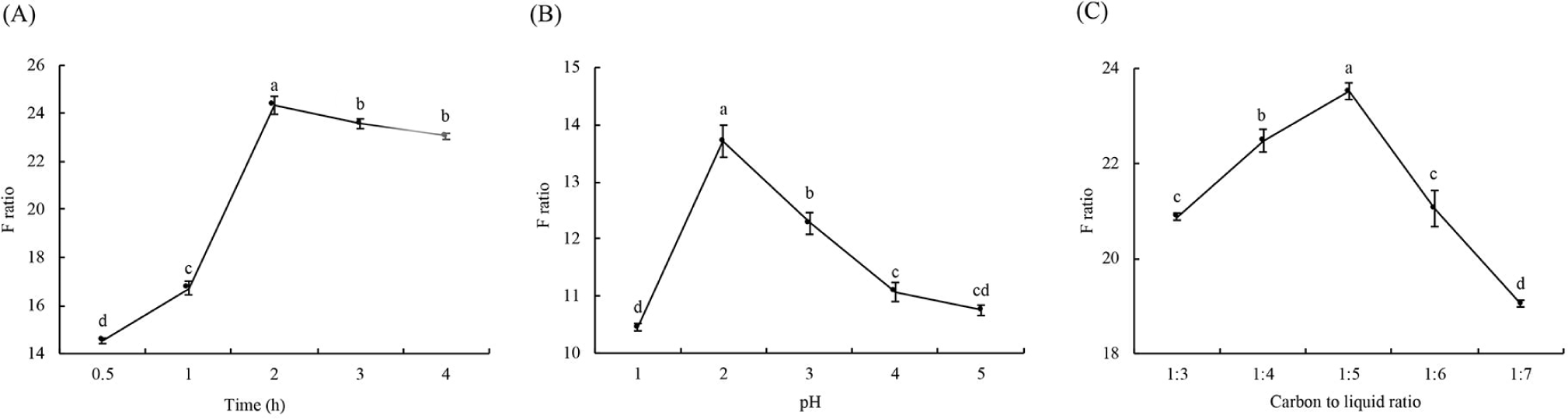
The amino acid profile of the HFO mixture was listed in Fig. 4A and Table 6. The BCAA content in the solution was 101.568 ng/mL and the phenylalanine content was only 3.752 ng/mL. BCAA accounted for 23.32% of total amino acids while AAA accounted for only 0.86%. The F ratio was calculated at 27.070, which is very close to the value of 26.32 calculated with absorbance values in 2.2.2. The content of Lys was 50.201 ng/mL, which can make up for the deficiency of lysine content in edible grain food. His, Tyr, Met, and Cys have been reported to have antioxidant activity (Xie et al., 2008). The results showed that when the amino acids have a high BCAA content and low AAA content, HFO mixture may have such functions as anti-fatigue and liver protection (Kawaguchi and Torimura, 2019; Mikulski et al., 2015; Park et al., 2020). HFO might be a good active ingredient to be added to the diet of patients with Phenylketonuria to reduce the intake of phenylalanine (Lichter-Konecki and Vockley, 2019). In addition, a total of 171.736 ng/mL of human essential amino acids were identified in this mixture. It might be reasonable to infer that HFO mixture obtained in this study was a hydrolysate possessing many physiological functions and nutritional value.
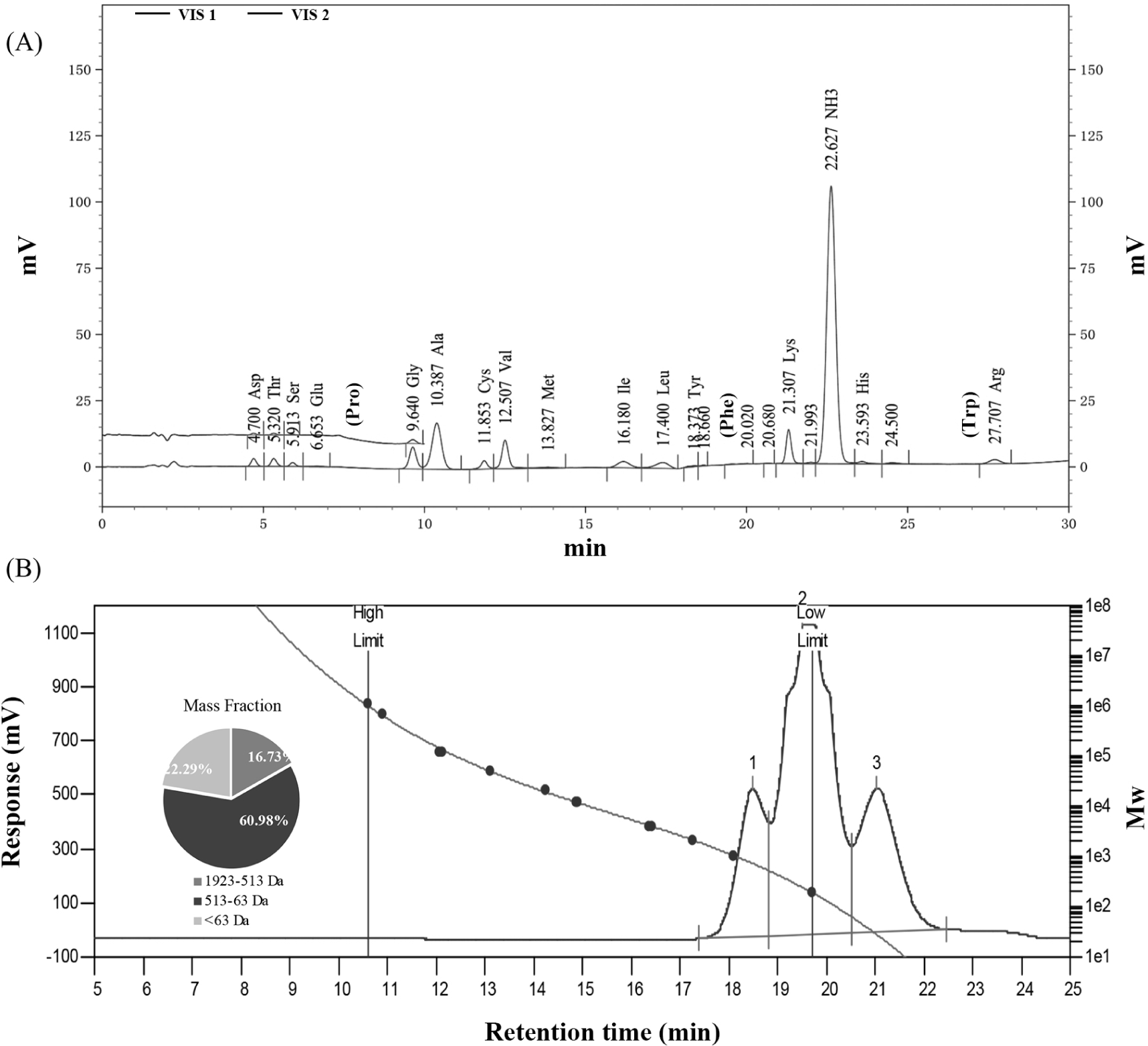
As illustrated in Fig. 4B, the hydrolysates showed three peaks: Peak 1, from 17.38 to 18.83 min, with the average Mw of 790, including about 4 to 7 amino acid residues of the medium peptide segment, accounting for 16.73%; Peak 2, from 18.83 to 20.51 min, with the average Mw of 236, dipeptides and tripeptides accounting for 60.98% of the total amino acids; Peak 3, from 20.51 to 22.47 min, with the average Mw of 29, which may be residual salts, accounting for 22.29%. Based on the result of amino acid composition, it can be concluded that HFO were obtained from goat whey after hydrolysis and adsorption. It is easier for small-molecular peptides such as HFO to be absorbed by human body than proteins and free amino acids. In addition, previous studies have reported that α-leaf protein with a Mw of below 1,000 Da has antioxidant properties (Xie et al., 2008), the hydrolysate with the strongest hydroxyl RSA in soybean protein components is mainly the peptides of 0.7–0.8 kDa (Moure et al., 2006). The Mw of HFO obtained in this study was similar to that of the peptides reported previously. Therefore, it was inferred that HFO might show good oxidation resistance due to its small Mw.
DPPH free radical is characterized by stability at room temperature, which is different from most other free radicals that are highly active but short-lived. The ability of HFO to scavenge DPPH was verified using ascorbic acid as the PC, as represented in Fig. 5A. There was an increase in the scavenging rate of DPPH with the increase of HFO concentration. A similar result has been reported for α-leaf protein hydrolysate (Xie et al., 2008). When the concentration reached 1.6 mg/mL, the scavenging rate tended to stabilize, and remained at about 77% (p>0.05), which indicated that the maximum activity of HFO in scavenging DPPH was observed at the concentration of 1.6 mg/mL. At the same concentration, the ascorbic acid showed a DPPH scavenging rate of over 95% (p>0.05).
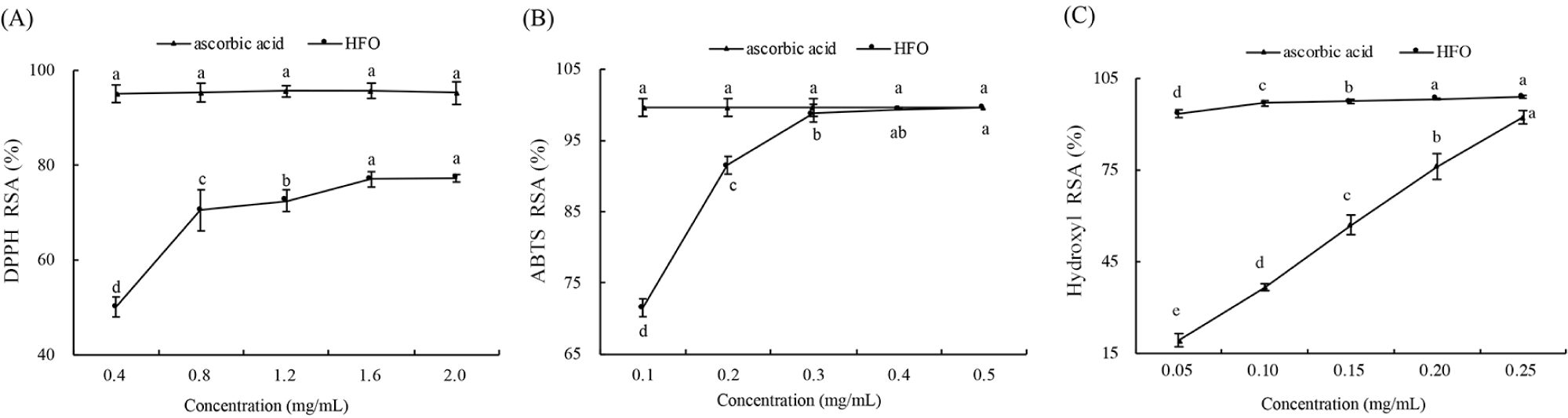
Antioxidants inhibit lipid peroxidation through blocking free radical chain reactions and chelating metal ions that promote lipid oxidation (Zheng et al., 2016). As is shown in Fig. 5B, the RSA of ABTS free radicals increased with the rise of HFO concentration. At the concentration of 0.5 mg/mL, the RSA of ABTS free radicals reached 99.63%, which was the same as the scavenging rate of ascorbic acid. It indicated that HFO had a strong ability of scavenging ABTS free radicals, namely strong antioxidant activity. The ABTS scavenging rate of HFO was much higher than that of the feather keratin antioxidant peptides reported previously (Fontoura et al., 2019). In the amino acid sequencing results obtained in this study, the concentration of Cys was 27.063 ng/mL, consistent with the finding that Cys plays a major role in the scavenging of ABTS (Huo et al., 2020).
Hydroxyl free radicals are the most reactive in comparison with other oxygen free radicals and have been proven to be highly damaging species in free radical pathology. It can react with almost all substances in cells and cause serious damage (Zhang et al., 2012). Therefore, the effective removal of hydroxyl free radicals may be a valid defense for the living body against various diseases. As is shown in Fig. 5C, the scavenging ability of HFO solutions against hydroxyl free radicals varied with the HFO concentration (p<0.05). When the HFO concentration was at 0.25 mg/mL, high hydroxyl scavenging rate was observed (92.31%), which was slightly lower than that of the same ascorbic acid concentration (98.53%). This result was much higher than the scavenging rate of soybean protein hydrolysate (69.75%). Soybean protein hydrolytes with the strongest ability of scavenging hydroxyl free radicals mainly consist of peptides from 0.7 to 0.8 kDa, which also indicated that the antioxidant activity of HFO was correlated with the low molecular weight. The high hydroxyl radical scavenging ability of HFO also may be due to their susceptibility to oxidation by extremely reactive hydroxyl radicals, enhancing its antioxidant activity and protecting the body’s ability of preventing hydroxyl free radical damage (Moure et al., 2006). The results of HFO about the hydroxyl RSA can provide useful information for antioxidant activity (Kitts, 2005).
Reactive oxygen species (ROS) are usually produced upon liver injury induced by CCl4, resulting in lipid peroxidation and oxidative stress (Yuan et al., 2005; Zelko et al., 2002). So in this study, CCl4 was selected to induce oxidative stress in mice (Fig. 6). Compared with the NC group, there was significant increase in MDA level in the MG (p<0.05; Fig. 6A), which indicated that oxidative stress was generated in mice after CCl4 induction and this model can be used to evaluate the antioxidant capacity of HFO in vivo. Compared with the MG group, HDG and LDG group showed significant declines in the level of MDA (p<0.05). As a product of membrane reaction between free radicals and unsaturated fatty acids, MDA reflects the sensitivity of lipid peroxidation and the degree of cell damage (Cemek et al., 2010; Meng et al., 2019). The results of this study showed that the intervention of HFO could delay the accumulation of lipid peroxides in vivo. Oxidative damage induced by CCl4 can not only cause changes in MDA, but also detect changes in oxidative stress parameters such as SOD and GSH-Px (Hamid et al., 2017; Lin et al., 2008). Antioxidant enzymes (SOD and GSH-Px) can convert peroxides into small or harmless substances (Zhang et al., 2003). The antioxidant enzymes are considered the main defense agent against oxidative damage of biological macromolecules, inhibiting the chain reaction of lipid peroxidation by effectively removing the production of ROS (Liu et al., 2018). As is shown in Fig. 6B, a decrease in SOD and GSH-Px was observed in MG group, indicating that excessive ROS may inhibit the development of antioxidant enzymes. Compared with MG group, there were significant increases in SOD and GSH-Px for HDG group and SOD for LDG (p<0.05), but GSH-Px of LDG showed no significant differences from that of MG. This observation may be due to the scavenging impact on free radicals by antioxidant enzymes. The results showed that HFO could alleviate oxidative stress induced by CCl4. This may be related to the amino acid composition of HFO and the molecular weight distribution. Leu and Ala could release protons easily to electron-deficient free radicals, exhibiting excellent free radical scavenging ability and metal ion chelation ability. Moreover, oligopeptides can be easily absorbed by the intestine to exert an excellent antioxidant effect in the body (Jiang et al., 2020; Li et al., 2008; Wu et al., 2013). A research has shown that oral administration of small peptides can improve the antioxidant activity in ultraviolet-induced photoaging mice, which is consistent with the results of this study.
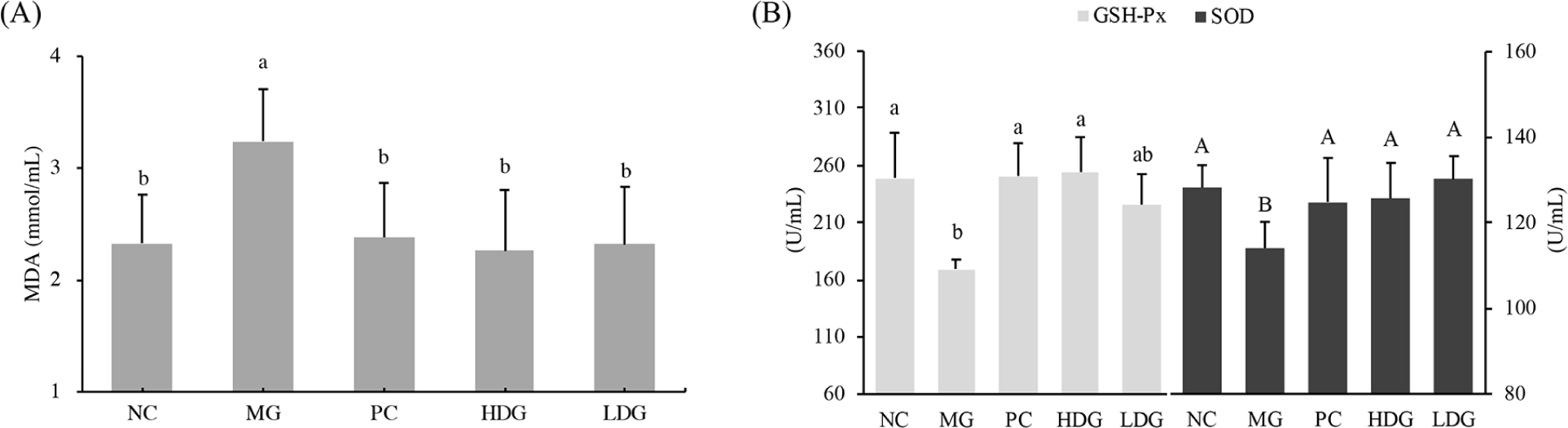
Conclusion
In this study, goat whey was used to prepare high F ratio oligopeptides with a F ratio of 27.070, and the optimized enzymatic hydrolysis and adsorption condition was determined. The HFO have a high BCAA concentration and low molecular weight of less than 1,000 Da. Free radical scavenging results showed that HFO has the ability of scavenging DPPH, ABTS and hydroxyl free radicals, and the characteristic of concentration-dependence. Animal experiment shows that HFO could reduce the MDA produced by oxidative stress in mice and elevate the level of SOD and GSH-Px in vivo. This work can not only offer an important basis for the high-value processing of goat whey, but also for the diversification of HFO sources, expanding the source of antioxidant peptides, while providing a theoretical basis for the development of HFO for special medical foods.