Introduction
Dry aging is a traditional method to store slaughtered livestock under regulated conditions for a certain period (Kim et al., 2017). This process can be affected by the aging period, temperature, relative humidity and air flow (Dashdorj et al., 2016). It has many positive effects on beef quality, such as increased concentrations of beef flavor and improved tenderness and juiciness (Berger et al., 2018). In addition, dry aging also modifies the microbiological, physical, and chemical characteristics of beef (Smaldone et al., 2019). Previous studies have shown that microbiological changes improve quality, safety, palatability and flavor. Changes in the composition of microorganisms, including bacteria, yeast and fungi, result in alterations in the quality of dry-aged beef. In particular, various Lactobacillus spp. have been detected on the surface of dry-aged beef, including L. sakei and L. plantarum (Ryu et al., 2018).
Lactobacilli have been studied for their association with many diseases, attenuating allergic responses and metabolic disorders. Among the Lactobacillus strains, L. sakei is renowned for its role in the fermentation of meat products (Zagorec and Champomier-Vergès, 2017). In fact, L. sakei is found in various fermented foods, such as sake, sourdough and kimchi, and it has several beneficial effects on host immunity and metabolism. Recently, Rather et al. (2018) reported that L. sakei has effects on the amelioration of skin diseases such as atopic and psoriasis, and Ji et al. (2019) reported that L. sakei could reduce a production of obesity-related biomarkers by increasing the level of short-chain fatty acids. For these reasons, L. sakei has been used as a starter culture in fermented foods and optimized for industrial use; however, only a few studies have been performed on dry-aged beef (Barbieri et al., 2020).
The nematode Caenorhabditiselegans is a widely used model system for featured biological studies because of its transparency, short generation time, ease cultivation, and availability of numerous mutants. C. elegans can also provide insights into the functional aspects of anti-aging and innate immunity (Park et al., 2018). In other studies, C. elegans presented extension of lifespan and increased resistance to pathogenic bacteria and oxidative stresses with a supplementation of probiotics (Clark and Hodgkin, 2014; Grompone et al., 2012). In addition, the structure of human intestinal cells is similar to that of the intestinal cells of C. elegans, allowing estimation of bacterial adhesion to the host gut system (Park et al., 2014). Lifespan and killing assays can be applied to screen L. sakei subspecies to determine whether they have an effect on antiaging or host defense mechanisms, respectively. For these reasons, we used the C. elegans model to evaluate the adhesion ability of bacteria isolated from dry-aged beef.
In the current study, we performed metagenomic analysis and found that dry-aged beef contains the Lactobacillus genus as the major portion of the microbiota. During the process of bacterial isolation, one L. sakei strain and three Enterococcus faecalis strains were isolated. Accordingly, we investigated their capability as probiotics by estimating their acid, bile tolerance and antibiotic sensitivity, antibacterial activity, and adhesion ability. Lastly, we performed C. elegans life-span and killing assays.
Materials and Methods
Longissimus thoracis of first grade Hanwoo cattle were used in this study. Dry aging process was performed at 1°C–4°C and a relative humidity of 80%–90% for 50 days and microbiological samples (approximately 5.0‐cm‐thick) were obtained from the surface of carcass. Samples from the dry‐aged beef were immediately transported to the laboratory at 4°C, without being vacuum packed.
Lactic acid bacteria including L. sakei and E. faecalis strains were isolated from fresh samples of dry-aged beef (without any starter cultures). Each samples were homogenized and serially diluted. The diluted solution was plated on De Man-Rogosa-Sharpe (MRS; BD Difco, Sparks, MD, USA) agar and incubated at 37°C for 48 h (Won et al., 2020). The selected isolates were identified by 16S rRNA sequencing. In every test, all strains were inoculated and sub-cultured in MRS broth at 37°C for 48 h to reach the appropriate growth phase before the experiment.
Metagenomic analysis from dry-aged beef samples were performed by the described methods previously. The bacterial DNA were extracted using a Powerfood Microbial DNA Isolation kit (Mo Bio Laboratories, Carlsbad, CA, USA) according to the manufacturer’s instructions and subjected to PCR according to 16S metagenomic sequencing library protocols (Illumina, San Diego, CA, USA). FASTQ files obtained from MiSeq data were analyzed using Mothur (v. 1.14). In Mothur, reads were combined using the make contig command and were quality-filtered by the screen.seqs command (Ryu et al., 2020). The Mothur pipeline was used to process sequence data for analysis according to the Mothur SOP manual (https://nothur.org/wiki/miseq_sop/).
The preparation of acidic and bile conditions was carried out according to the modified methods previously (Ashraf and Smith, 2016; Park et al., 2014). To make acidic conditions that reflect a stomach, the MRS broth was adjusted to pH 2.5 with 6 N HCl. After autoclaving, pepsin from porcine gastric mucosa (Sigma‐Aldrich, St. Louis, MO, USA) was added to a final concentration of 1,000 units per milliliter and was filter-sterilized using 0.45-μm pore size syringe filters. In the acid tolerance test of L. sakei and E. faecalis strains, 100 μL of isolate was inoculated into 10 mL of acidic solution and incubated at 37°C for 0 h and 3 h. Subsequently, plate counting was performed and compared to 0 h incubated solution as a control.
The bile solution was prepared by suspending oxgall powder (Acumedia, Lansing, MI, USA) in MRS broth to a final concentration of 0.5% (w/v). All isolates were inoculated into 10 mL of bile solution and incubated at 37°C for 24 h. The survival rate was calculated by counting the final viable population after 3 h of incubation compared with the initial viable counts at 0 h, which were immediately treated.
We used the disc diffusion method to examine the antibiotic susceptibility of L. sakei isolates following the modified standard Kirby-Bauer method as used by Sharma et al. (2016). One hundred microliters of each isolate grown for 48 h in MRS broth was spread on MRS agar plates, and the discs with antibiotics were placed on the surface, followed by incubation at 37°C for 24 h. We used ampicillin (10 μg), chloramphenicol (30 μg), kanamycin (30 μg), penicillin (10 μg), tetracycline (30 μg) and vancomycin (30 μg) antibiotic discs. After incubation, inhibition zone diameters were measured, and the degree of inhibition was categorized as resistant (R, zone diameter ≤14 mm), intermediate resistant (IR, zone diameter 15–19 mm) or susceptible (S, zone diameter >20 mm).
To evaluate antibacterial activity, we used 4 pathogenic bacterial strains: Listeria monocytogenes EGD-e, Staphylococcus aureus Newman, Salmonella Typhimurium SL1344 and Escherichia coli ATCC 35150. The pathogenic bacterial strains were inoculated and incubated in LB medium at 37°C for 24 h except L. monocytogenes, which was incubated at 30°C for 48 h. The pathogenic bacteria were spread on LB agar medium, and then 5 μL of isolated bacterial strains from dry-aged beef was inoculated as a spot (Gomes et al., 2012). The antibacterial activity against pathogenic bacteria was categorized into 6 standards. The diameter of the inhibition zone was classified as <10 mm, none (−); >10 mm, weak (+); >15 mm, middle (+ +); >20 mm, strong (+ + +); or >25 mm, very strong (+ + + +) inhibition (Lin et al., 2020).
To perform a mucin adhesion assay, we dissolved type III porcine gastric mucin (Sigma‐Aldrich) to 1% in distilled water and filter-sterilized the solution using a 0.45-μm pore size syringe filter. After filtration, a 100-μL aliquot of mucin solution was added to a 96-well plate and allowed to attach for 24 h at 4°C. Then, we added bacterial strains to the 96-well plate after removing the mucin solution and incubated at 37°C for 2 h. Subsequently, we removed the bacterial solution and washed the plate 5 times. Next, we added 200 μL of 0.1% Triton X-100 (Daejung, Siheung, Korea) to detach the mucin and diluted the solution removed from the plate in 0.85% NaCl. Finally, we dropped 5 μL of the solution into MRS agar medium and incubated it at 37°C for 48 h (Valeriano et al., 2014).
For the life span and killing assay experiments, we used the C. elegans fer-15; fem-1 model, which is unable to produce progeny at 25°C without alteration in the phenotype. Worms were maintained on nematode growth medium (NGM) agar and seeded with E. coli strain OP50. Eggs were obtained using sodium hypochlorite-sodium hydroxide solution, and all synchronized L1 worms were grown at room temperature. To identify the degree of bacterial colonization in the C. elegans intestinal tract, we measured the number of bacterial cells in worm intestines. After exposing C. elegans to individual bacterial strains on NGM for 24 h, 10 worms were picked randomly and placed on brain heart infusion agar (Sigma-Aldrich) plates containing gentamycin (25 μg/mL) for 5 min. Next, worms were transferred to a 1.5-mL Eppendorf tube containing M9 buffer with Triton X-100 and were mechanically disrupted using a pestle (Kontes Glass, Vineland, NJ, USA). These diluted worms were plated on MRS agar medium and incubated at 37°C for 48 h. Lactobacillus rhamnosus GG (LGG) and E. coli OP50 were used as positive and negative controls, respectively.
For the C. elegans life span assay, 100-μL volumes of concentrated bacteria were plated on 35-mm-diameter NGM agar plates, and L4 stage C. elegans fer-15; fem-1 worms were individually transferred with a platinum wire onto OP50 or isolated bacterial strain plates. For each life span assay, we assayed 90 worms per bacterial species in three separate plates, and all were incubated at 25°C. Total number of worms was counted daily and determined whether alive or dead by touching with a loop. To perform a C. elegans killing assay, L4 stage worms were placed on conditioning plates with isolated bacterial strains or E. coli OP50 for 24 h. Afterward, pathogenic bacteria, including S. aureus Newman and E. coli O157:H7 EDL933, were prepared and inoculated on NGM agar plates. Prepared nematodes were transferred onto plates with pathogens and incubated at 25°C. Living worms were transferred to fresh pathogen plates every day during the assay periods.
Results
In our previous study, we found that the dry-aging process altered the microbial composition in beef. Alpha- and beta-diversities that indicate diversity of microbes were altered, especially, the abundance of Lactobacillus genus increased until 30 days of dry-aging, and it decreased when it reached at 70 days (Ryu et al., 2020). Based on the findings, we analyzed a 50-day sample to identify certain alterations between 30 and 70 days that are transition points for the microbiota. Samples were aged at 1°C–4°C and a relative humidity of 80°C–90°C for 50 days. Metagenomic analysis revealed that the Lactobacillus genus had the highest abundance in dry-aged beef, followed by Bifidobacterium. Enterococcus existed at an abundance of approximately 3%. These data were in accordance with findings at the phylum and genus levels (Fig. 1). Consequently, we cultured lactic acid bacteria present in dry-aged beef samples on MRS agar plates in order to examine their characteristics. Finally, we isolated one L. sakei sample labeled 20D49 and three E. faecalis samples labeled 12D26, 20D48, and 30D36.
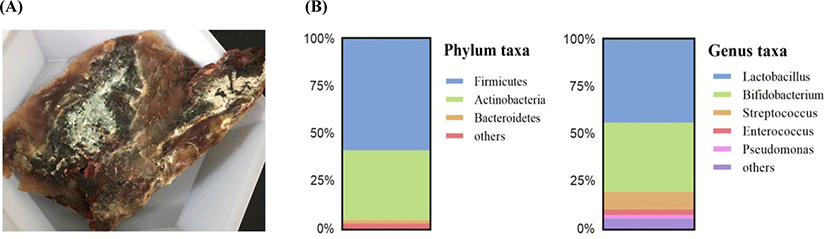
Probiotics must survive in extreme acidic and bile conditions to have a positive impact on host where the gastrointestinal environment is reflected (Mallappa et al., 2019). We compared the isolated bacterial abilities that survive on acid and bile media in a timely manner. LGG has the ability to survive and proliferate under acidic and bile conditions, and thus used as a standard along with the test strains (Capurso, 2019). Four bacterial strains isolated from dry-aged beef survived at acid MRS media after 3 h of exposure (Fig. 2). In particular, the 12D26 isolate showed a remarkable survival rate (88%). Although the 20D48, 20D49 and 30D36 isolates had lower survival rates than LGG in terms of acid tolerance, they had survival rates greater than 70%, suggesting that they have tolerance in acid.
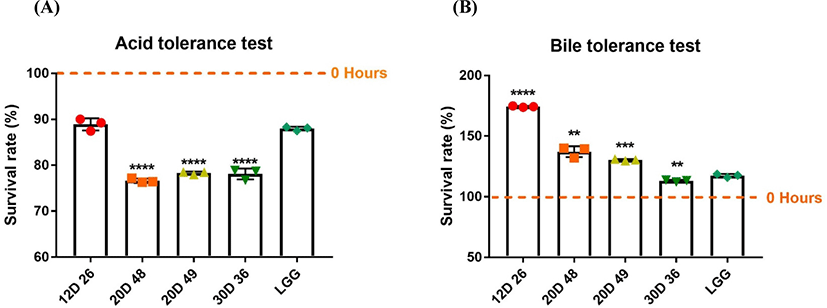
In the bile tolerance test, bacterial strains were exposed to 0.5% bile in MRS broth for 24 h. In our experiment, all isolates survived and they even grew under bile conditions. In particular, the 12D26 isolate had outstanding capacity for enduring bile stress that similar to the small intestine condition. Other three strains showed 74%–77% of survival rates which were comparably high than other bacterial strains. Overall, all strains isolated from dry-aged beef presented superior acid and bile tolerance, and 12D26 demonstrated remarkable survival capacity in these experiments.
Antibiotic sensitivity assays verify the susceptibility of bacteria to antibiotics using antibiotic discs. In the current study, we tested bacterial susceptibility to antibiotics, including ampicillin (10 μg), chloramphenicol, kanamycin, penicillin, tetracycline and vancomycin. All isolates indicated different patterns of antibiotic sensitivity and were susceptible to chloramphenicol and penicillin (Table 1). In particular, 20D49 had remarkable results because it did not have antibiotic resistance to most of the antibiotics except for vancomycin and kanamycin. Isolates 12D26 and 30D36 were resistant to kanamycin and tetracycline but sensitive to chloramphenicol, penicillin and vancomycin. Our results suggest that the bacterial isolates are susceptible to a portion of antibiotics, and L. sakei 20D49 may have a benefits with respect to the antibiotic susceptibility.
Variable | E. faecalis 12D26 | E. faecalis 20D48 | L. sakei 20D49 | E. faecalis 30D36 |
---|---|---|---|---|
Ampicillin | I | S | S | I |
Chloramphenicol | S | S | S | S |
Kanamycin | R | R | I | R |
Penicillin | S | S | S | S |
Tetracycline | R | R | S | R |
Vancomycin | S | S | R | S |
Antibiotic discs included ampicillin (10 μg), chloramphenicol (30 μg), kanamycin (30 μg), penicillin (10 μg), tetracycline (30 μg) and vancomycin (30 μg).
Probiotic strains must have outstanding antimicrobial properties to eliminate or inhibit pathogenic bacteria. The antagonistic activity of Lactobacillus and Enterococcus against bacterial pathogens is a characteristic worth consideration (Samot and Badet, 2013). Here, we tested the antibacterial activity of L. sakei and E. faecalis strains against pathogenic bacteria, including L. monocytogenes EGD-e, S. aureus Newman, S. Typhimurium SL1344 and E. coli ATCC 35150. As the result, all isolated bacteria showed outstanding antimicrobial activity against L. monocytogenes, S. aureus, S. Typhimurium and E. coli. L. sakei and E. faecalis strains produced clear zones larger than 17 mm in diameter. These results show that all of the strains isolated from dry-aged beef have antimicrobial properties that can inhibit pathogens.
Probiotics have many beneficial effects on humans under the condition that they survive and proliferate in the environment of the host’s gastrointestinal tract (Fernández et al., 2003). When they survive in the human gut, they must have the capability to adhere to the human mucus layer (Tuo et al., 2018). We identified the adhesion ability to mucin compared with a commercial strain as a positive control: LGG, which is well known for its adhesion ability. We found that all of the L. sakei strains and E. faecalis strains had mucin adhesion profiles similar to LGG (70%; Fig. 3A). In conclusion, all of the isolated strains from dry-aged beef showed adhesion ability to mucin.
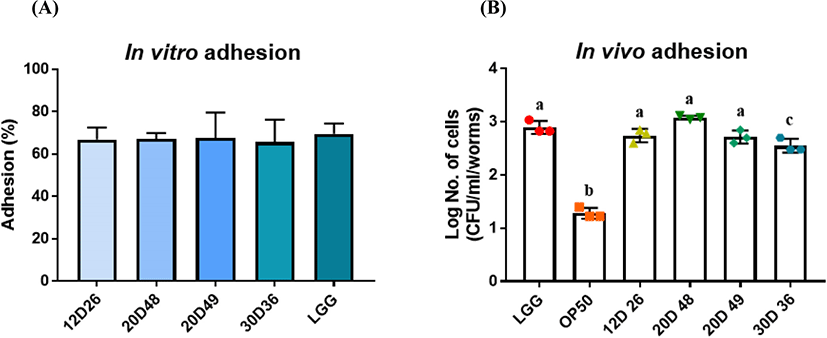
Next, we attempted to examine the adhesion capacity of the strains by using a C. elegans model. Because we defined that all of the isolates had adhesion ability in mucin in vitro, we identified their adhesion capacity in vivo using C. elegans. LGG and E. coli OP50 were used as positive and negative controls, respectively. In this study, 12D26, 20D48, and 20D49 indicated outcomes similar to LGG and higher adhesion capacity compared with OP50 by approximately two-folds. Although the 30D36 attached to the nematode intestine less than LGG, the adhesion was considerably improved compared with E. coli strain OP50. In conclusion, L. sakei and E. faecalis strains had remarkable adhesion capacity in the C. elegans model compared with the E. coli OP50 control strain.
In previous experiments, we observed that L. sakei and E. faecalis strains had great potential as probiotics. We next examined whether the isolates had beneficial health effects on the host using a C. elegans model system. In the lifespan assay, we used 6 bacterial strains, including one of L. sakei and three of E. faecalis strains isolated from dry-aged beef. Additionally, LGG and E. coli OP50 were used as positive and negative controls, respectively. In this study, we observed that the 12D26 increased the lifespan of C. elegans, similar to LGG, and its effect was distinctly distinguished from E. coli OP50 (Fig. 4). Along with the 12D26, other strains had beneficial effects on life extension compared with E. coli OP50, although their effects were slightly lower than the effect of LGG. The 20D49 was also significantly different from E. coli and LGG. Therefore, all of the isolated bacteria, especially the E. faecalis 12D26 and the L. sakei 20D49, had exceptional effects on C. elegans lifespan extension.
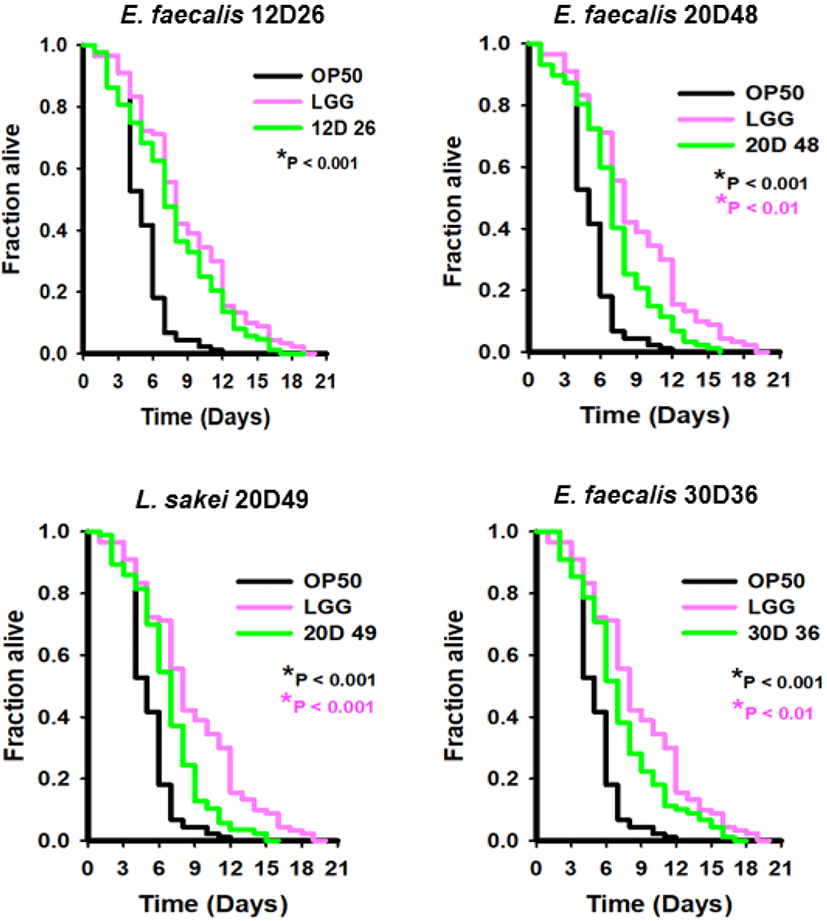
In addition to the lifespan extension analyses, we further performed killing assays to identify whether the L. sakei and E. faecalis isolates influenced host resistance against exposure to food-borne pathogenic bacteria (Fig. 5). After, C. elegans were transferred to the isolated bacterial lawn for 1 day, they were exposed to S. aureus Newman or E. coli O157:H7 EDL933. These bacteria can kill the nematode through an infection-like process in the intestine (Irazoqui et al., 2010). When worms were exposed to the E. coli O157:H7 strain without pretreatment with the isolated strains, they were killed within 14 days after transfer to the pathogen. However, pre-exposure with our isolates attenuated the pathogenic effects, increasing the lifespan of C. elegans. In particular, 20D48 had the best ability to influence host resistance against pathogens, while the other three strains had no significant effects on prevention compared with OP50 and LGG. In experiments with S. aureus, worms were also killed within 14 days, similar to E. coli O157:H7. Pretreatment of the isolates 12D26, 20D49, and 30D36 showed comparably significant improvements in host resistance compared with OP50. In contrast, the 20D48 strain was similar to E. coli strain OP50 and did not affect host resistance against S. aureus. Based on our results, the isolated strains had different abilities to enhance nematode resistance depending on the type of pathogen. Among the strains, 20D49 and 30D36 had resistance to promote host defense conditioned with S. aureus, while they had resistance similar to E. coli strain OP50 under pathogenic E. coli O157:H7.
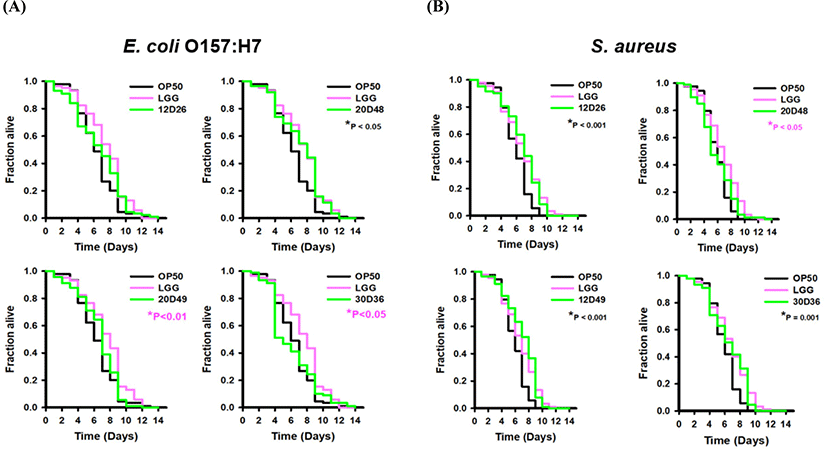
Discussion
Dry aging of beef improves flavors due to the absorption of juice and the chemical breakdown of protein and fat (Dashdorj et al., 2016). Through this alteration, the quality and safety of dry-aged beef are improved and microbiological changes play an important role in these aspects. In our previous study, total bacteria and lactic acid bacteria were significantly increased during the dry-aging period (Ryu et al., 2018). From this perspective, we were able to affirm the changed microorganisms in dry-aged beef, which may influence quality and safety in meat. In our study, isolates from dry-aged beef had superior acid and bile tolerance and adhesion abilities that were similar to LGG. Furthermore, most L. sakei and E. faecalis strains influenced the lifespan of C. elegans compared with E. coli strain OP50 in both normal plate conditions and plates inoculated with pathogens.
When we analyzed the microbiota composition of dry-aged beef, the relative abundance of the Lactobacillus genus was highest. Accordingly, we isolated the L. sakei strain from dry-aged beef and characterized its functionality as a probiotic. L. sakei is found in various fermented foods, especially in dry-aged meat, and it has many beneficial effects on the host. Previous studies discovered that L. sakei regulates allergic Th2 responses, which enhance Treg gene production and change the relative abundance of gut bacteria (Kwon et al., 2018). Moreover, the treatment of L. sakei induced reductions in adipose tissue and various biomarkers associated with obesity resulting from the gut microbiota-modulating ability and stimulation of SCFAs by L. sakei.
In this study, our results clearly showed that the L. sakei and E. faecalis isolates from dry-aged beef possess acid and bile tolerances, anti-bacterial activities, and great adhesion abilities. A previous study demonstrated that the L. sakei strain isolated from fresh pork sausage presented a low survival rate under pH 2.5 conditions; however, we confirmed that L. sakei isolated from dry-aged beef had a superior survival rate at pH 2.5. In addition to acid tolerance, the bile tolerance of L. sakei from fresh pork sausage decreased with time, while L. sakei from dry-aged beef increased with time (Gomes et al., 2012). These differences would be due to the dry-aging process, considering that the experimental results of acid and bile tolerance are similar. Furthermore, because of the subspecies differences of isolates from dry-aged beef, the four strains might have different abilities in acid and bile tolerance compared with previous reports. L. sakei is known for producing bacteriocin, which influences the adhesion of pathogenic bacteria (Winkelströter et al., 2011). Previously, L. sakei can inhibit the bacterial growth of gram-positive pathogens, including L. monocytogenes (Liserre et al., 2002). In addition to L. sakei, E. faecalis also produce bacteriocins that inhibit gram-positive pathogen growth (Toit et al., 2000). In this respect, we found that all of the isolates from dry-aged beef have the ability to inhibit not only gram-positive pathogens but also gram-negative pathogens. Regarding the adhesion ability, L. sakei isolated from healthy humans feces had low or absent adhesion ability in a C. elegans model, while the L. sakei strain isolated from dry-aged beef had great adhesion ability in a C. elegans and a mucin assay (Lee et al., 2015; Park et al., 2014). This implies that the significant C. elegans adhesion ability of the isolates might result from differences at the subspecies level.
C. elegans takes bacteria as a source of nutrition, and bacteria play a key role in the regulation of C. elegans lifespan (Kim and Mylonakis, 2012). The 12D26 strain had an excellent effect on C. elegans lifespan extension in particular. For the killing assay, bacterial isolates showed different abilities with each pathogen. In this study, 20D49 and 30D36 had the ability to attenuate the S. aureus infection; however, they had little ability to affect the host resistance against E. coli O157:H7. Various studies have investigated the effects of Lactobacillus on the lifespan and fitness of C. elegans (Kim and Mylonakis, 2012; Park et al., 2014; Park et al., 2018). However, there are a few studies that utilize the C. elegans model for L. sakei application. In the model system, we identified the effects of L. sakei and E. faecalis on antiaging and innate immunity using the lifespan and killing assays. These strains extended the lifespan of C. elegans and protected them from the bacterial pathogenicity, ultimately prolonging the life of nematodes.
Taken together, L. sakei and E. faecalis strains exhibited remarkable ability as probiotics, including acid and bile tolerance, good performance in antibiotic sensitivity tests, and antibacterial activity. Additionally, they showed magnificent results based on C. elegans lifespan and C. elegans killing assays. Based on this study, we identified L. sakei and E. faecalis strains isolated from dry-aged beef could be attractive probiotic candidates for human wellness as well as production of fermented meat.