Introduction
Probiotic bacteria, Lactobacillus rhamnosus GG (LGG) is a well-documented probiotic strain (Doron et al., 2005), which has many clinical benefits such as preventing and relieving certain types of diarrhea (Guandalini et al., 2000), reducing the incidence of respiratory infections in children (Hojsak et al., 2010), impeding atopic disease (Guandalini et al., 2000), and preventing alcohol-induced liver injury (Bull-Otterson et al., 2013; Wang et al., 2011; Wang et al., 2013). It is well known that LGG can colonize in the intestine (Rijkers et al., 2010) while it has low resistance to various environmental factors including pH, temperature, bile salt and others (Martin-Dejardin et al., 2013). Therefore, several approaches have been adopted to improve the survival rate of probiotics in the gastrointestinal tract.
Microencapsulation is one of the most extensively used methods to protect probiotics (Zorica et al., 2012), and the selection of wall materials is crucial. Proteins (whey protein, soy protein etc.) and polysaccharides are widely applied for the microencapsulation (Sultana et al., 2000; Wang et al., 2013; Yao et al., 2017), which could improve the viability of probiotics in adverse environmental conditions. Whey protein concentrate (WPC) has been used as the wall material of microencapsulation for many years. The major protein of WPC is β-lactoglobulin which is mainly responsible for the irreversible thermal denaturation and gelling properties of whey protein (Schmitt et al., 2001), because there are two disulphide bonds and one free thiol group in β-lactoglobulin (Shimada and Cheftel, 1989). Compared with free cells, the viable counts of Bifidobacterium breve R070 encapsulated with whey protein microparticles were increased 2.6 Log cycles in yogurt stored at 4°C after 28 d and 2.7 Log cycles after exposure to simulated gastric and intestinal juices (Picot and Lacroix, 2004). In addition, Eckert et al. (2017) used whey, permeate and whey retentate as wall materials to encapsulate L. plantarum and the viable cell counts of all microencapsulation were above 6 Log CFU/g after 56 d at 20°C, which reached the minimum requirement for functional foods. Pullulan (PUL), another major wall material of the microencapsulation in this study, is a highly water-soluble microbial extracellular polysaccharide which is from the fungus like yeast, Aureobasidium pullulans (Yuen, 1974). PUL mixed with different polysaccharides in gel-forming and microencapsulation materials has been reported (Biliaderis et al., 1999). Moreover, its effect on edible films cross-linked with soy protein isolate (Xu et al., 2001) and caseinate (Kristo and Biliaderis, 2006) has been also studied.
The objective of this study was to prepare a new kind of microencapsulation to improve the resistance of probiotics (LGG) to gastrointestinal condition. The wall material of this microencapsulation was the WPC-PUL gel and the concentration of PUL and pH was optimized during the gel preparation to get the best protective effect on LGG during exposure to simulated gastrointestinal conditions. Moreover, the relationship between the gel properties and the microcapsule protection was investigated preliminarily.
Materials and Methods
In this study, Lactobacillus rhamnosus GG (ATCC 53103) was obtained from the American Type Culture Collection (ATCC). WPC containing 80% whey protein was supplied by Hilmar lngredients (CA, USA). PUL was purchased from Tokyo chemical industry Co. (Tokyo, Japan).
Lactobacilli MRS Broth was obtained from BD Biosciences-Advanced Bioprocessing (MD, USA), while mucin, pepsin, trypsin and MRS Agar were supplied by Sigma-Aldrich Co. (MO, USA). Sodium chloride (Beijing Chemical Works, China), calcium chloride (Sigma-Aldrich Co., MO, USA) were used to prepare microencapsulation. All other chemicals were of analytical grade.
The reactivation of LGG was cultured in MRS Broth (3%, v/v) at 37°C for 24 h. The cells were harvested by centrifuging at 3,000×g for 15 min at 4°C and washed twice with 0.85% sterile sodium chloride solution. Washed cells were suspended in 0.85% sterile sodium chloride solution and obtained a cell density at 10 Log CFU/mL. The viable counts of LGG were determined by pouring plate on MRS agar and incubating at 37°C for 48 h.
The WPC solution was prepared according to Cabuk and Tellioglu Harsa (2015) method with some modification. The WPC powder was dissolved in the distilled water at ambient temperature and stirred for 2 h using a magnetic stirrer at 4°C, then the solution was stored at 4°C overnight (about 16 h) for dissolution. The preparation of the gel solution was performed by using WPC solution to dissolve PUL with stirring for 2 h at ambient temperature and the PUL concentration was 6.0%, 7.0%, 8.0%, and 9.0%. Next, the solution was adjusted to pH 7.0, 7.5, 8.0, and 8.5 for the pH experiments. Finally, the gel solutions were transformed to the gels by heating in a closed container (80°C, 20 min) and cooling to ambient temperature.
The ratio of the washed LGG cell suspension (60 mL, section Preparation of LGG) to the WPC-PUL gel (20 mL) was 3:1 and the mixture was homogenized by stirring at a constant speed (3,000 rpm). The microparticles were prepared by extruding the emulsion into the calcium chloride solution (3 M) with 1.0 mL syringe needle, the distance between the needle and the calcium solution was controlled about 1 cm. Then the microparticles were stored in the calcium chloride solution for 30 min to immobilize the microencapsulation. After that, microparticles were washed twice with sterile distilled water and freeze-dried with a vacuum freeze dryer (GPERGN, Republic of Korea) for about 48 h, and the final moisture content of all samples were controlled less than 5% (w/w). The images of the microparticles in the calcium chloride solution were captured by a camera. The particles size of the dried microparticles was measured with vernier caliper, 100 microparticles were randomly selected for measurement.
Encapsulated LGG were released from the microencapsulation according to the methods of Krasaekoopt et al. (2004) with some modification. Briefly, 0.1 g microparticles was resuspended in 10 mL of 0.85% sterile sodium chloride solution. The number of microparticles (CFU/g) was measured by plating on MRS agar and incubating at 37°C for 48 h. After incubation, the viable counts of LGG were calculated (De Man et al., 1960).
The simulated gastric juice (SGJ) and simulated intestinal juice (SIJ) were prepared according to Gebara et al. (2013) with modification. Mucin (3.5 g/L) and pepsin (0.26 g/L) were added to SGJ after sterilization and pH was adjusted to 2.0 with 1M HCl. Trypsin (1.95 g/L) was added to SGJ and pH was adjusted to 7.5 with 1M NaHCO3 to obtain SIJ. SGJ and SIJ were sterile-filtered with a membrane filter (0.22 μm).
Based on the results (section Effect of PUL concentration and pH on the WPC-PUL gel microparticles appearance), 10% WPC, 8.0% PUL and pH 7.5 was chosen to prepare the WPC-PUL gel (80°C, 20 min) and microencapsulation as the samples. The control was free bacteria, the bacterial suspension without the gels. The viable counts of LGG in microencapsulation after exposure to SGJ and SIJ were counted as follows. First, 0.1 g of the microparticles were added to 10 mL SGJ and incubated for 0, 2, and 4 h at 37°C. Next, SIJ was used instead of SGJ and incubated for 0, 2, and 4 h at 37°C. Moreover, SGJ and SIJ were preheated at 37°C in a water bath for 20 min before the addition of microparticles. Surviving probiotics after incubation were counted by pouring plate counts in MRS agar at 37°C for 48 h.
The digestion of the gel protein was analysed by sodium dodecyl sulphite polyacrylamide gel electrophoresis (SDS-PAGE). Based on the results, 10% WPC, 8.0% PUL and pH 7.5 was chosen to make the WPC-PUL gel (80°C, 20 min) as the sample. The control (the only WPC sample) was prepared by 10% WPC at pH 7.5 without 8.0% PUL (80°C, 20 min). Then, the gel was freeze-dried to powder, and the powder was resuspended with SGJ and SIJ, then incubated at 37°C for 0, 2, 4 h and centrifugated at 12,000×g for 15 min. The supernatant was diluted with ddH2O until the concentration of protein was 1mg/mL. After preparation, the sample and control were analysed by SDS-PAGE. The electrophoresis was lasted 2 h at 120 V. Then the gel was stained with Coomassie brilliant blue R250 solution containing isopropanol, acetic acid and dH2O (5:2:15, by vol.) for 1 h and distained with a solution composed of methanol, acetic acid and dH2O (2:1:17, by vol.) for 40 min thrice. Finally, the gel was scanned by the gel imager (DNR Bio-Imaging Systems Ltd., Israel).
Hardness of WPC-PUL gel at different PUL concentration and pH was measured by a texture analyser (TA Instruments Ltd., UK). The two compression mode was used, between two compressions for 5 s, and the compression deformation is 20% of the sample height (the sample was a cylinder with height 50 mm and diameter 70 mm), distance of the probe set 8.0 mm, crosshead speed of 1 mm/s, at room temperature (Fiszman and Salvador, 1999; Pang et al., 2014; Turgeon and Beaulieu, 2001).
The centrifugal method was used to determine the water-holding capacity of the gels according to Kocher and Foegeding (1993) — with slight modification. The gels were centrifugated at 2,000×g for 5 min at 25°C. The results presented as percentage (water/gel, w/w).
Both the biological experiments and analytical tests were conducted at least three times. The results were analysed statistically to find the standard deviation in GraphPad Prism version 6.01 for Windows (GraphPad Software, La Jolla California USA), which is indicated in the graphs as error bars. The significance of the differences was performed by analysis of variance (ANOVA) and Least Significant Difference (LSD) method was used for post hoc analysis. Data analysis was carried out using SPSS 24.0 software (IBM Corp, Armonk, NY, USA).
Results and Discussion
In this study, 10% WPC was dissolved with dH2O, and PUL was added into WPC solution at 6.0%, 7.0%, 8.0%, and 9.0% (w/v). The influence of PUL concentration on microencapsulation appearance was shown in Fig. 1A. The shape of microparticles with 8.0% PUL (Fig. 1A.c) was best, which was round and 1.5–2.0 mm in diameter. By contrast, when PUL concentration was less than 7.0% (Fig. 1A.a and b) and more than 9.0% (Fig. 1A.d), the round-shape was not formed.
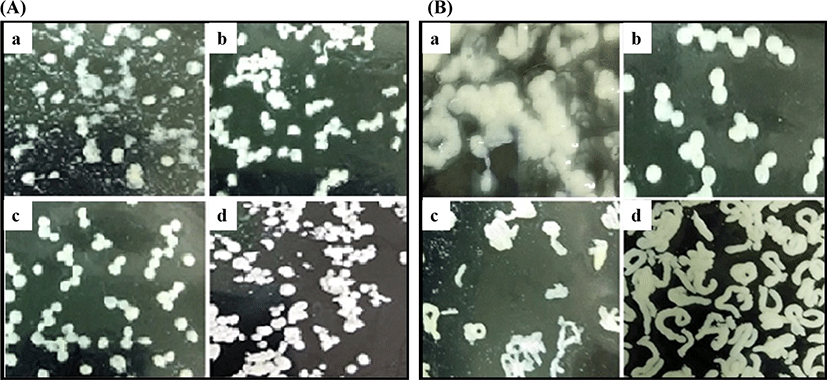
Appropriate concentration of PUL can improve the cross-linking with WPC because of its strong viscosity. However, with the addition of PUL increased to 9.0%, the viscosity became large and hindered the interaction between the molecules. Compared with the microparticles made from whey protein isolate (WPI) (Peters et al., 2015) and the cold-gelated whey protein microparticles (Peters et al., 2016), the size of microparticles in this study was too bigger than others. The microparticles were a combination of many small microencapsulation units and were produced by extruding from the needle, so the volume was large and can be visible by the naked eye without any device, easy to observe. Therefore, the images in the Fig. 1 was captured by a camera.
pH is one of the main factors affecting the structure of proteins. According to many studies (Li and Zhao, 2018; Liyanaarachchi and Vasiljevic, 2018; Otte et al., 1996), pH plays a crucial role on the structure of the whey protein gel. Therefore, the effect of pH on the microencapsulation appearance was studied (Fig. 1B). According to the results of the previous section, 8.0% PUL was chosen to prepare the WPC-PUL mixed solution (without heating). Then the solution was adjusted to pH 7.0, 7.5, 8.0, and 8.5 followed by transformed to the gel by heating, and the shape of the microparticles was observed. As shown in Fig. 1B, the effect of pH on the microparticle appearance was significant. The microparticle shape was round or irregular round at pH 7.5. With the change of pH, the shape of the microparticles became extremely irregular. This suggested that the structure of the microparticles was changed significantly when pH was 7.0, 8.0, and 8.5. We can infer that pH is a crucial influence factor on the cross-linking with WPC and PUL, and the spatial structure of the protein was destroyed due to too high or too low pH, which was not favour of the combination between WPC and PUL. According to the results of Liu et al. (2018), when pH was lower than pI of protein, the microparticles of alginate and caseinate would be formatted because of the strong electrostatic interaction between anionic groups and cationic groups. In addition, Schmitt et al. (2009) also reported that the concentration of denatured protein increased from 20% (pH 3.0) to 90% (pH 5.0). These suggested that pH plays a vital role in the formation of microparticles. However, it needs further experiments to determine the effect of pH on the structure of WPC-PUL gels.
The previous results (Fig. 1) showed that when the concentration of PUL was 8.0%, the shape of the microparticles was good, which indicated the PUL concentration can affect the density and microstructure of the microparticles. Then the effect of PUL concentration on the viability of encapsulated LGG after exposure to SGJ and SIJ was determined (Fig. 2). The same concentration of LGG cell suspension was added to different WPC-PUL gels, so the remained cell counts can reflect the effect of PUL concentration on the viability of bacteria. As shown in Fig. 2A, the viable counts of LGG were 6.92, 6.98, 7.24, and 6.80 Log CFU/g after exposure to SGJ for 4 h, when the concentration of PUL was 6.0%, 7.0%, 8.0%, and 9.0%. This suggested that the remained cell counts were largest at 8.0% PUL, so the microencapsulation made from 8.0% PUL had the best protective effect on LGG during exposure to SGJ. The viable counts of LGG were 7.24 Log CFU/g after exposure to SGJ for 0 h and 6.66 Log CFU/g for 4 h, decreased 0.58 Log CFU/g. The viability of L. rhamnosus encapsulated with the isomaltooligosaccharides and WPC decreased more than 1 Log CFU/g after exposed to SGJ (Liu et al., 2017), and the viability of L. plantarum 80 in alginate-poly-L-lysine-alginate microcapsules decreased 1.39 Log CFU/mL at pH 2.0 for 60 min (Martoni et al., 2007). Maybe the viable counts were related to the strain and the wall materials of microencapsulation. From the stomach to the intestine, pH 7.5 and 37°C in intestine were very suitable for the proliferation of LGG. The viable counts of bacteria in 8.0% PUL microencapsulation were obviously higher than other PUL concentration (Fig. 2B). After stored in the SIJ for 4 h, the cell counts of LGG were 9.04, 9.01, 9.40, and 8.98 Log CFU/g. Compared with the results after SGJ treatment, the cell counts of all samples were increased. This suggested that LGG was released from the microencapsulation gradually in the intestine with time going. When the PUL concentration was 8.0%, the viable counts of LGG was bigger than others, indicated that 8.0% PUL microencapsulation has the best protective and released effect on LGG during exposure to SIJ. Combined with the results after exposure to SGJ, when the WPC-PUL gel was made from 8.0% PUL, the viable counts of LGG was largest after both SGJ and SIJ treatment. Therefore, 8.0% was the best concentration of PUL to prepare the WPC-PUL gel for LGG protection.
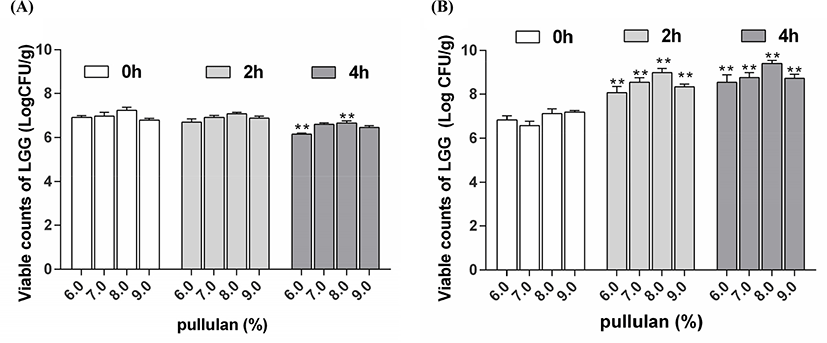
According to Ainis et al. (2018), the addition of acid and alkali not only destroyed the natural structure of the protein but caused the protein molecules to carry electric charge, resulting in electrostatic repulsion and hindering the heat-induced reaction between proteins and polysaccharides. Therefore, the effect of pH on the viability of encapsulated LGG after exposure to SGJ and SIJ was determined (Fig. 3).
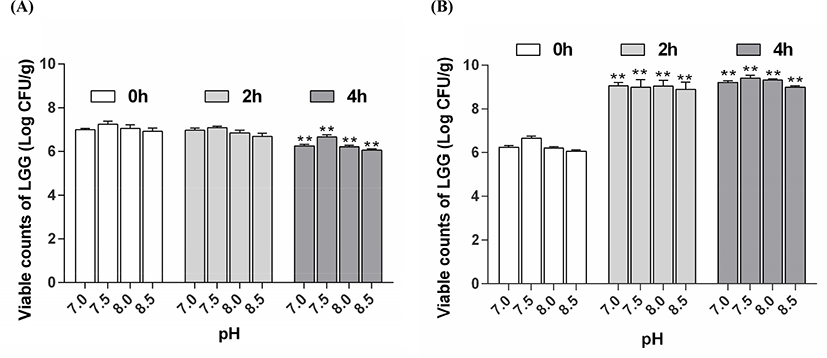
In this study, the results (Fig. 3A) showed that the viable counts of LGG was 6.24, 6.66, 6.21, and 6.06 Log CFU/g after exposure to SGJ for 4 h, when the WPC-PUL gels were prepared at pH 7.0, 7.5, 8.0, and 8.5. The viable counts of LGG was maximum at pH 7.5, suggested that the WPC-PUL gel prepared at pH 7.5 has the best protective effect during exposure to SGJ. Importantly, the probiotics must be released from the microencapsulation to grow and proliferate in the intestine (Almuzafar and Amin, 2017). The viable counts of LGG were 9.21, 9.41, 9.32, and 8.99 Log CFU/g (Fig. 3B) after exposure to SIJ for 4 h, when the gels were made at pH 7.0, 7.5, 8.0, and 8.5. The result presented that all cell counts of LGG encapsulated with the gel increased and the pH 7.5 gel was significantly higher than the gels made at other pH. This showed that LGG encapsulated with the gel prepared at pH 7.5 can released better than others in SIJ. Because the counts of LGG encapsulated with the gel at pH 7.5 was largest after both SGJ and SIJ treatment, pH 7.5 was the best choice for microencapsulation.
The current work was aimed to determine whether LGG encapsulated with WPC-PUL gels can serve as a protective vehicle to transport and release bacteria in gastrointestinal juice. Therefore, the viability of free bacteria (FB) and microencapsulated LGG (ME) was measured after stored in the SGJ and SIJ for 4 h continuously (Fig. 4).
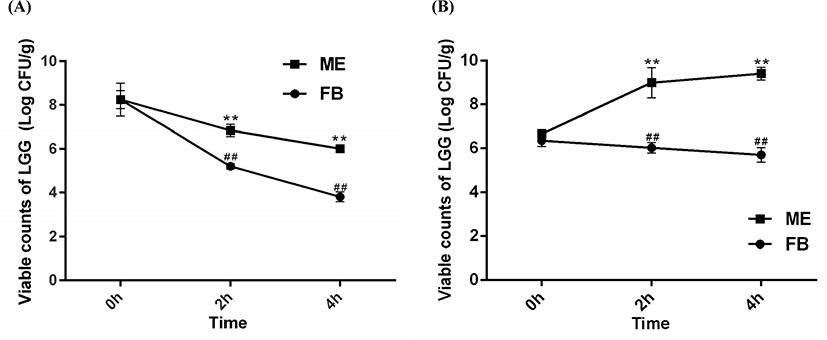
Based on the previous results, LGG was encapsulated with the WPC-PUL gel made from 10% WPC, 8.0% PUL and pH 7.5. The microcapsule was stored in the SGJ and SIJ for 4 h continuously. Compared with the results (Fig. 4A), the survival rate of FB was decreased by 52.44% after exposure to SGJ for 4 h. However, the viable counts of ME were 7.24 Log CFU/g at 0 h and 6.66 Log CFU/g at 4 h, and the rate of viability was decreased only 8.01%, which was better than FB. The reason may be that the activity of FB has been destroyed by the acid environment of the gastric juice. Therefore, we can infer that LGG encapsulated with WPC-PUL gels has a better survival rate during exposing in the gastric juice.
As time went on, the viability of FB was decreased gradually in the SIJ (Fig. 4B) while the viable counts of ME were increased. This suggested that WPC-PUL gels played an important role in slow release and protection for LGG. Compared with the survival rate of bacteria, FB was decreased by 10.24% but ME was increased by 41.14% (9.40 Log CFU/mL), which presented that LGG encapsulated with WPC-PUL gels could release and colonize well in the intestinal juice. The other reason for the increasing of the viable cells in the SIJ may be that the protein and polysaccharides in the wall material provided a nitrogen source and a carbon source to promote LGG growth. The wall gel of microencapsulation was digested and the probiotics in the core were released; therefore, the bacteria can colonize in the intestinal tract and confer benefits on the host (Buddington, 2009).
Moreover, many studies of encapsulated and free probiotics exposure to the simulated gastrointestinal juice was also reported (Gbassi et al., 2011; Picot and Lacroix, 2004). L. acidophilus was encapsulated with calcium alginate and the viable counts after exposure to SGJ and SIJ were 9.0 Log CFU/g, which also had a significant increase (p<0.05) compared with free cells (Chandramouli et al., 2004). As well as B. adolescentis encapsulated with a combination of alginate and gelatine, the results showed that the microencapsulation played a significant role in protecting bacteria from the simulated gastrointestinal conditions (Annan et al., 2008). The results of this study are consistent with previous studies, that is, the microencapsulation can improve the survival rate of probiotics in gastrointestinal juice effectively.
To further confirm the protection of the WPC-PUL gel for LGG, the distribution of the gel protein after exposure to SGJ (Fig. 5A) and SIJ (Fig. 5B) was analysed by SDS-PAGE. As shown in Fig. 5, the 18.4 kDa bond is β-lactoglobulin (β-lg) and the 14.2 kDa bond is α-lactalbumin (α-la). WPC is mainly consisting of β-lg (54%) and α-la (21%), thereby the digestion of the gel protein can be reflected. The sample (the WPC-PUL gel made from 10% WPC, 8.0% PUL and pH 7.5) and control (the only WPC sample, without 8.0% PUL and others same with the sample) were incubated in the SGJ and SIJ for 4 h. Then the supernatant after centrifugated at 12,000×g for 15 min was considered as the digested part of the samples. With time went on, the control was digested in the SGJ completely while the WPC-PUL gel sample digested slightly (Fig. 5A). This indicated that the dissolution of the WPC-PUL gel in the SGJ was poor, which can preserve LGG in the microencapsulation and resist the destruction of low pH. In the SIJ (Fig. 5B), compared with the control, the sample digested incompletely, but with the extension of time, the gap was more and more small. This indicated that the WPC-PUL gel can digest gradually and release LGG then enable it colonize in intestinal tract.
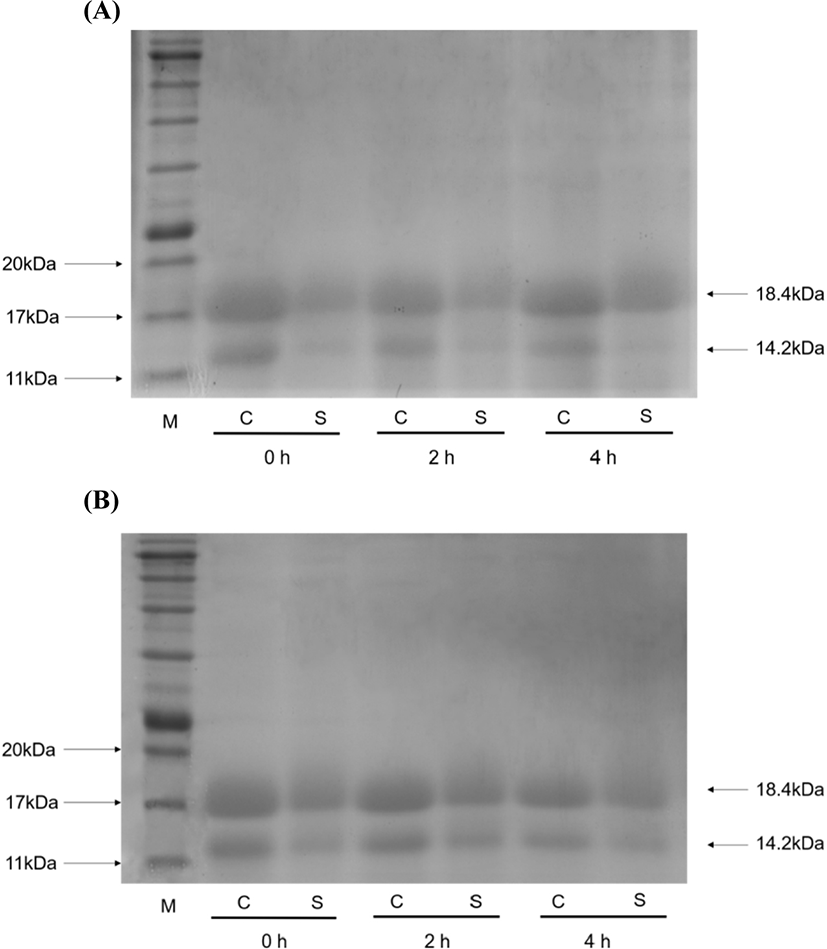
The hardness of WPC-PUL gels was increased with the PUL concentration increased (Fig. 6A). When the addition of PUL reached 9.0%, the gel hardness was changed sharply from 18.1 N/mm2 to 27.0 N/mm2, and the gel was semi-solid. The interesting thing was that the microparticles shape and the viable counts of LGG were the best when the PUL concentration in the WPC-PUL gel was 8.0% not 9.0%. This result suggested that the hardest gel did not have the best protective effect. Zhang et al. (2012) has studied the compound film made from whey protein, PUL, and ferulic acid. The properties of the film can be improved obviously by adding PUL and ferulic acid. The compound film has good tensile properties, piercing performance and lower water vapor transmittance, which the optimal film-forming composite was ferulic acid 200 mg/100 mL, PUL 150 mg/100 mL. Moreover, the effect of xanthan gum on the physical properties of thermal induced whey protein gel was studied by Bryant and Mcclements (2000), and the results showed that 0.1% xanthan gum can cause great change of the gel hardness and the speed of formatting gels by adding sodium chloride solution. These indicated that the addition of polysaccharides can affect the hardness of the gel, which consisted with the result in this study.
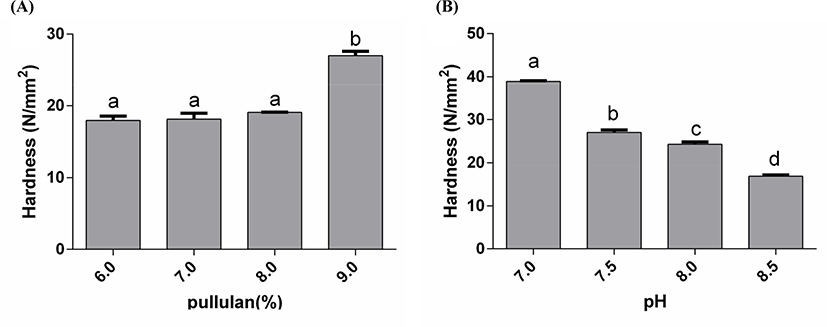
The differences in hardness of gels formed at different pH were significant. As shown in Fig. 6B, with pH increased, the hardness of the gels was decreased. When pH was 7.5 and 8.0, the gels hardness has no significance (p>0.05). The hardness of the gel at pH 7.0 was highest. This also suggested that the protective effect of the microencapsulation was related to the properties of the WPC-PUL gel, but the hardest gel did not have the best protective effect. Anema (2018) reported the effect of pH on the properties of acid gels prepared by heated skim milk with glucono-δ-lactone. The results showed that the gel had higher hardness and yield strains when pH of the milk increased at heating. Moreover, the emulsion gel can be made from WPI and gliadin nanoparticles (GNP)-stabilized emulsion and the strongest gel was formed when pH was near the isoelectric point of the proteins (pH 5 and 6.8) (Zhu et al., 2018). These indicated that pH plays a crucial role on the hardness of the gel. Compared with WPI (protein concentration more than 90%), the protein content of WPC was 80% in this study. Although the protein concentration of WPC was lower than WPI, the gels made from WPC and PUL in this study also presented good protective effect at a lower product price.
Water-holding capacity is one of the most fundamental texture properties of gels (Li et al., 2018). When the concentration of PUL was 6.0%, 7.0%, 8.0%, and 9.0%, the water-holding capacity of the gels was 37.9%, 40.9%, 50.3%, and 50.5% (Fig. 7A). This presented that the water-holding capacity increased gradually with the increase of PUL concentration. On the other hand, the change tend of gels water-holding capacity also increased with the raise of pH. When the gels were made at pH 7.0, 7.5, 8.0, and 8.5, the gels water-holding capacity was 44.4%, 49.8%, 55.2%, and 60.0% (Fig. 7B). However, the viable counts of LGG were not the best when the water-holding capacity reached maximum, suggesting that the relationship between the protection and the water-holding capacity of the gel is non-linear.
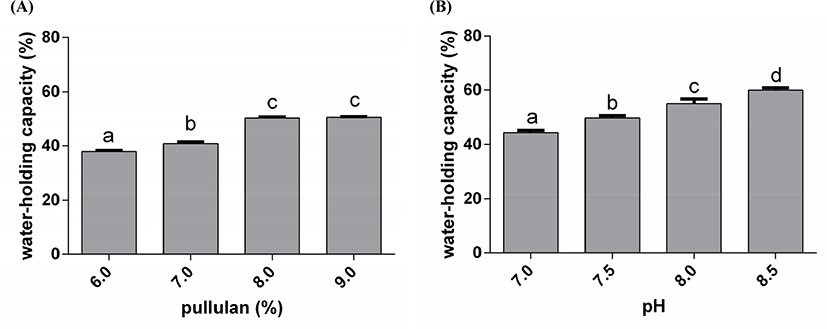
In the process of gelation, there may be hydrophobic interaction, hydrogen bond, covalent bond and electrostatic attraction. The content of disulphide bond in the gels was increased with pH. The structure of PUL also contains many hydroxyl groups, which have hydrophilic interaction. In addition, the acid gel groups, like hydroxyl, became ionization in alkaline solution, which lead to the gel carried negative charges, and expanded by the electrostatic repulsion (Schmitt et al., 2009). In contrast, in an acidic environment, the gel was syneresis and reduced the moisture. In the solution of WPC and PUL, the system became negatively charged. With the action of electrostatic repulsion inside the networks, protein networks were stretched. In addition, PUL is a neutral polysaccharide, which can combine with protein into network space structure and make water molecules more easily enter the protein networks to interact with hydrogen bonding. From the previous research, the water-holding capacity of whey protein isolate gels was 70% when the addition of sodium chloride was 250 mM (Chantrapornchai and Mcclements, 2002). In this study, the water-holding capacity of WPC-PUL gels was about 50% without sodium chloride. Therefore, microencapsulation made from the WPC-PUL gel was suitable for the products which have strict moisture and iron requirements, such as probiotics and dried products.
Conclusion
In this study, the microencapsulation made from WPC-PUL gel is effective to improve the viability of LGG in the gastrointestinal juice. The concentration of PUL and pH are optimized during the gel preparation, and the optimum gel is made from 10% WPC, 8.0% PUL and pH 7.5. Moreover, the hardest gel does not have the best protective effect, the same as the water-holding capacity. Therefore, the relationship between the protective effect and the properties of the gel is non-linear, and the mechanism needs the further study.