Introduction
Bifidobacteria are Gram-positive bacteria which are commonly present in healthy human’s intestinal tract; especially they occupy approximately 90% of total bacteria in feces of breast-fed infants and about 3% to 5% in adult fecal microbiota (Ham et al., 2011). Through the application of genomic sequencing technique, it has been shown that Bifidobacterium longum is absent from virulence and pathogenicity factors and it is considered as the safest bacteria without health hazards (Meile et al., 2008). The bifidobacteria have been proven to produce beneficial effects on the human host through the prevention of intestinal colonization of pathogens and production of bioactive fatty acids etc. (Kwon et al., 2017). Till now, a significant number of human Bifidobacterium genera (e.g., B. longum and B. infantis etc.) have been used as the potential probiotics (Masco et al., 2005; Srůtkova et al., 2011). In our previous study, a Bifidobacterium strain (KACC 91563) has also been proven to show its probiotic effects such as foods allergy alleviation (Kim et al., 2013).
In the dairy products such as yogurt, cheese and fermented milk etc., the probiotic B. longum has been included as a functional ingredient or starter culture in order to improve the health beneficial effects and technological quality characteristics of the products (Albenzio et al., 2013; Sabikhi et al., 2014; Song et al., 2017).
One of the crucial criteria in production of probiotic products or foods containing the probiotic bacteria is that the microorganisms included must successfully survive in the undesirable conditions (e.g., acidic and salty environments) of the gastrointestinal tract or food matrix, and this is the biggest challenge for the producers. Regarding the probiotic bifidobacteria, studies conducting on the effects of various environmental conditions have reported that the environment containing greater 3.5% NaCl significantly reduced the esterase activity, and the salt resistance of B. longum has been found to be lower than other bacterial species such as lactobacilli (Gandhi and Shah, 2015). Also, the major challenge to the bifidobacteria is acid stress which significantly reduces their viability and probiotic effects (Wang et al., 2015).
In recent time, in order to protect the probiotic microorganisms against such adverse environments of the gastrointestinal tract and foods, the microencapsulation techniques have been applied for the probiotic products production. The nature of this technique is packaging cells in small capsules by using some coating materials which provide the living cells with physical barrier (Chavarri et al., 2010). Studies have reported that the application of encapsulation resulted in improved viability and stability as well as functionality of B. longum cultures during freeze-drying and storage as well as gastro-intestinal conditions (Amine et al., 2014; Chavarri et al., 2010). Alginate is among the most commonly used coating materials for encapsulating probiotic microorganisms due to its non-toxic nature (Amine et al., 2014; Chavarri et al., 2010); however, it still shows limitations such as not always improving the probiotic microorganism’s survival (Chandramouli et al., 2004). In order to overcome the problem with alginate encapsulation, researchers have recently used fatty acids, glycerol or chitosan as the cryoprotectants to improve the survival of probiotic bacteria by reducing acid diffusion (Amine et al., 2014).
Though a great amount of information regarding the health beneficial effects of the probiotic bifidobacteria has been published and available, also many attentions have been paid to its applications in the dairy food products production as described above. To the best of our knowledge, however, there is no study focusing on evaluation of the applicable efficiency of the probiotic B. longum in fermented meat products production. Since the B. longum has shown its potential probiotic effects and also quality improvements in fermented dairy products (Albenzio et al., 2013; Song et al., 2017), therefore, the evaluation for utilization possibility of the probiotic bacteria for the fermented meat products production is necessary.
Thus, the main objective of this work was to evaluate the utilization possibility of encapsulated B. longum KACC 9156 for production of healthy fermented sausages as a functional starter culture.
Materials and Methods
In the present study, the probiotic B. longum KACC 9156 used was isolated from fecal samples of healthy Korean neonates. The whole genomic sequences and proteins-encoding sequences as well as total G+C content of the bacteria were determined as described in our previous study (Ham et al., 2011).
The B. longum (0.5 mL of approximately 106 CFU/mL stock frozen in –80°C deep freezer) was first cultured in 40 mL of Man, Rogasa & Sharp (MRS) broth supplemented with L-cysteine at 37°C for 48 h. Thereafter, the 40 mL of MRS containing the B. longum were sub-cultured in 3 L of the MRS supplemented with L-cysteine at 37°C for 48 h in a fermenter. The bacteria were harvested by centrifuging at 3,000×g for 10 min, and twice washed with 0.85% sodium chloride solution. The washed B. longum were then freeze-dried using a vacuum-freezing dryer. Finally, the freezing-dried bacteria (in powder form) were used for encapsulating using the protocol as described by Amine et al. (2014).
Four different treatments of fermented sausages: 1 control (C: non-inoculated), 1 added with 0.02% (w/w) commercial starter culture (CSC) containing Staphylococcus carnosus and Lactobacillus sakei (Oftering, Austria), 1 inoculated with 0.02% (w/w) encapsulated B. longum (T1) and 1 added with 0.02% (w/w) encapsulated B. longum and 0.02% (w/w) B. longum in saline solution (T2) were prepared. In our previous examinations (data not shown), non-encapsulated B. longum could not survive in the fermented sausages after 2 days of fermentation. In this work, therefore, in order to determine whether the non-encapsulated B. longum can survive in the products during processing, the non-encapsulated B. longum in saline solution was also added into the T2 and considered as an extra control. All treatments (3 batches/treatment) were prepared with about 10 kg meat mixture including: 80% pork ham, 20% pork fat, 2% NaCl, 1.5% sugar, 0.2% black pepper, 0.2% polyphosphate, 0.2% sodium ascorbate, 0.01% sodium nitrite and 0.005% sodium nitrate. All the treatments were prepared on the same day and in an identical manner, following our previous procedures (Ba et al., 2016). The humidity levels set in the fermenting rooms were same for all the treatments and control as following: 15°C/90% for 18 h, 23°C–25°C/90% for 48 h, 15°C/80%–90% for 10 days and 14°C–15°C/75%–80% until the samples reached a water activity of about 0.81–0.83. At the end of the ripening/drying (22nd day), the samples were collected and used for analyses.
The pH values of the samples during processing were measured in triplicates using a pH meter (Model 340, Mettler-Toledo GmbH, Ohio, USA). Water activity (aw) was determined using a water activity measuring instrument (Model AW SPRINT-TH 300, Novasina Co, Lachen, Switzerland) The procedures used for determinations of pH and (aw) were the same as described in our previous work (Ba et al., 2016).
The proximate compositions (protein, fat, and moisture) were analyzed using a Food ScanTM Lab 78810 (Foss Tecator Co., Ltd., Hillerod, Denmark), according to the procedure as described in our previous work (Ba et al., 2016).
The instrumental color was determined at different areas on the freshly cut surface of each sample using a Minolta Chroma Meter CR-400 (Minolta Camera Co., Ltd., Osaka, Japan) that was standardized with a white plate (Y=86.3, X=0.3165, and y=0.3242). Color was expressed according to the Commission International de l’Eclairage (CIE) system and reported as CIE L*(lightness), CIE a*(redness), CIE b*(yellowness), chroma and hue angle (h°). In which the chroma and hue angle were calculated as (a*2+b*2)0.5 and tan–1 (b*/a*), respectively.
The TPA was performed using a puncture probe (7 mm diameter) attached to a texture Analyzer (Model 4465, Instron Corp., Norwood, USA). Each sample was cut into 2.54-cm long pieces; each the cube was axially compressed twice to 80% of its original height. The speed of load cell was set at 120 mm/min and the following textural parameters were calculated: hardness (kg), cohesiveness (kg*mm), gumminess (kg) and chewiness (kg*mm).
The total lactic acid bacteria (LAB) and bifidobacteria were determined during processing (0, 4, and 22nd day). The LAB and bifidobacteria were cultured on MRS (Difco) agar and Bifidobacterium Selective Medium, respectively. Briefly, after removing the casing, about 10 g of each sample was taken aseptically and placed into sterile stomacher bag containing 90 mL of saline solution. The samples were then homogenized for 1 min using a stomacher (Model: Bagmixer 400 W, Interscience, France). Before plating, appropriately serial dilutions were made using saline buffer solution. Approximately 100 µL of each diluted solution in each sample was plated on the agar plate. The plates were then incubated in a 37°C- incubator for 24–48 h. Each sample was done in duplicates and total count was expressed as log numbers of colony forming units/gram (CFU/g).
The lipid oxidation was determined by measuring the thiobarbituric acid reactive substances (TBARS) content as described by Pikul et al. (1989). Particularly, each sample (10 g) was homogenized with 35 mL of 4% perchloric acid and 1 mL of 7.5% butylated hydroxyansole (BHA) at 13,000 g for 20 s using a homogenizer (Polytron MR-2100, Kinematica AG, Luzern, Switzerland). Prior to filter through No. 1 Whatman filter paper, the volume of the homogenate was adjusted to 50 mL with 4% perchloric acid solution. Thereafter, 5 mL of each filtrate was taken and transferred to separate 50 mL- tubes and added with 5 mL of 0.02 M thiobarbituric acid solution. After mixing, the reaction mixture was heated at 80°C for 1 h in a water-bath. Finally, approximately 1.5 mL of each sample was taken and the absorbance was measured at 532 nm using an UV-visible spectrophotometer (ProteomeLab Du-800, Brea, USA). The TBARS values were expressed as mg malonaldehyde/kg (MAD/kg) of sample. Three repetitions were applied for each sample in each treatment.
Biogenic amines were determined using the protocol as described in detail in our previous study (Ba et al., 2016). The samples were derivatized by dansyl chloride (5-dimethylaminonaphthalene-1-sulfonyl chloride, DCl) and then separated onto an Eclipse XDB-C8 column (150 mm×4.6 mm×5 μm particle size, Supelco) connected to a high performance liquid chromatography (HPLC, Agilent 1100, Agilent Technologies, Inc., Waldbronn, Germany). The separated amines were identified by comparison of the retention times of known standards. The concentrations of the identified amines were expressed in mg/kg of sample.
Fatty acid compositions in samples were extracted using a solvent mixture of chloroform: methanol (2:1, v/v) as described by Folch et al. (1957) and then the extract was methylated using the procedure of Morrison and Smith (1964). The fatty acids were separated on a capillary column (30 m×0.32 mm i.d.0.25 µm film thickness) connected to a Gas Chromatography (GC, Model Star 3600, Palo Alto, USA). The GC condition was set as follows: 250°C for injection port and 300°C for detector. The free fatty acids in samples were identified by comparing their retention times with those obtained from standard fatty acids. The results were expressed as relative percentages based on total peak area.
Ten randomly-selected sausages from each the treatment were used. The panelists used were the members of Animals products Development Division, National Institute of Animal Science, Korea, and they were chosen on the basis of previous experiences in sensory evaluation of fermented meat products. The sensory samples were prepared as described in by Ba et al. (2017). Briefly, each sausage sample from the treatments was tested by 6 panelists. And six 0.3-cm thick pieces were taken from each the sausage, placed onto dishes and coded with random numbers. The sensory samples were randomly allotted into sessions and each session had 6 panelists. The panelists were served with the sensory samples in a random manner. The panelists evaluated 4 major sensory traits such as color, odor, taste and overall acceptability for each samples and rated using a 7-point scale (7=extremely like; 6=like very much; 5=like moderately; 4=neither like nor dislike; 3=dislike moderately; 2=dislike very much and 1=dislike extremely).
The obtained data was statistically analyzed using a Statistic Analysis System (SAS) package (SAS, 2007). The data were analyzed by using the General Linear Model procedure considering treatment/or processing time as the main effect. The differences between means were compared by using Duncan's Multiple Range Test, and significance was defined at (p<0.05).
Results and Discussion
The proximate compositions of the fermented sausage samples at the end of ripening were analyzed and found as follows: The levels of fat, moisture and protein among the treatments ranged from 37.27% to 39.93%, 19.42% to 23.37% and 28.20% to 32.02%, respectively. Statistical results showed that these compositions were not significantly different among the treatments, as the same raw materials and formulation were used for their production. In general, the levels of fat and protein contents in all the samples in this study were higher than those reported for the same product type in our previous works (Ba et al., 2016; Ba et al., 2017). These contrasting results could be related to the moisture content differences among the studies.
Table 1 presents the weight loss percent and water activity (aw) of the samples at the end of ripening, and the pH values in the samples during the processing periods (0, 4, and 22 days). The aw-values among the treatments ranged from 0.80 to 0.81, and the values in all treatments were not significantly different from the control (p>0.05). It is well known that the water activity largely influences the shelf-life stability of food products; low aw can increase the shelf-life stability (Fernandez-Salguero et al., 1993). The aw-values in all the samples in the present study were lower than the values (0.85–0.87) reported for the same product type in literature (Corral et al., 2016) but similar to the aw value reported by Ba et al. (2016). The contrasting results could be related to the differences in moisture contents in samples among the studies. The weight loss ranged among the treatments from 49.29% to 51.19%. The control or the treatment with CSC had approximately 1% higher weight loss level in comparison to those made with encapsulated B. longum, while the similar loss level was found for the two treatments made with the encapsulated B. longum (T1 and T2). Corral et al. (2016) reported lower weight loss levels (37%–38%) for the same product type; this could be due to the higher moisture contents (37%–38%) in samples in their study.
Regarding the pH values, no statistical differences occurred among the treatments at the processing day (0 day) (p>0.05). At 4th day, the pH values significantly (p<0.05) differed among the treatments in the following order: Control>T1 (encapsulated B. longum)>T2 (encapsulated B. longum+B. longum in saline)>CSC, being their mean values of 6.07, 5.29, 4.98 and 4.86. The pH values continuously declined with prolonged ripening time up to 22nd day with the same order; the values were found as 6.03, 5.24, 4.88 and 4.81 for the control, T1 (encapsulated B. longum) and T2 (encapsulated B. longum+B. longum in saline) and CSC, respectively. The pH variations could be related to the differences in the levels of fermenting activities by LAB or other bacterial species among the treatments. In general, the control presented the highest pH values whereas the treatment with CSC showed the lowest value after 4–22 days fermenting/ripening. These results are in agreement with the findings of Ba et al. (2016) and Sun et al. (2016), who also reported lower pH values for samples inoculated with microbial starter cultures. However, when compared to the pH values (6.03–6.07) of control samples during processing (4–22nd day) the samples made with encapsulated B. longum presented significantly lower values (4.84–5.29). This means that the samples inoculated with encapsulated B. longum showed the higher acidifying activity in comparison with the control, suggesting the fermentative efficiency of the bifidobacteria in the products. Regarding this, Nguyen et al. (2009) also reported the strong fermentative activities of Bifidobacterium strains in fermented milk. Similar findings have also been reported for other food products such as dairy products (e.g., cheese) inoculated with B. longum (Albenzio et al., 2013; Sabikhi et al., 2014; Song et al., 2017). These authors have reported that the cheese samples inoculated with B. longum had significantly lower pH values throughout the processing periods as compared to non-inoculated samples. In general, the pH values in all the samples inoculated with the encapsulated B. longum in the present study were almost similar to values (4.5–4.9) reported for the dairy products added with B. longum as mentioned above, and were similar to pH values (4.66–5.24) in fermented sausages made with starter cultures containing LAB (Ba et al., 2016; Kaban et al., 2009; Sun et al., 2016).
The changes in number of LAB and APC as well as the viable counts of B. longum during processing are presented in Table 2. The LAB count showed differences among the treatments on all days determined. The LAB counts ranged among the treatments from 4.83 to 6.97, 7.87 to 8.73 and 7.95 to 8.88 Log CFU/g at 0, 4, and 22nd day, respectively. It was observed that the number of LAB significantly (p<0.05) increased after 4 days of fermentation and then did not significantly increase during the ripening process for all the treatments, except the treatment with CSC. At the end of ripening, the LAB counts were in the following order: CSC>Control>T1 (encapsulated B. longum)>T2 (encapsulated B. longum+B. longum in saline), being their mean values of 8.88, 8.68, 8.44, and 7.95 Log CFU/g, respectively. In general, the samples added with CSC presented the highest LAB count after 4–22 days of ripening; and this may be responsible for the lowest pH values (Table 1) in these samples. Although the control samples presented the second highest LAB count (after the CSC treatment), however, they had higher pH values than the samples inoculated with the encapsulated B. longum (T1 and T2) (Table 1). This suggests that there were a high number of spontaneous non-starters LAB in the control samples (Leroy et al., 2006).
The viability of the bifidobacteria was determined during fermenting/ripening process. Our results showed that the initial viable counts of bifidobacteria (day 0) were 5.88 and 7.05 Log CFU/g for the T1 (added with 0.02% encapsulated B. longum) and T2 (added with 0.02% encapsulated B. longum and 0.02% B. longum in saline solution), respectively. At 4th day, the numbers of bifidobacteria tended to decrease (e.g., 5.40 and 5.08 Log CFU/g in the T1 and T2, respectively), and no significant differences occurred between these two treatments (p>0.05). These results signified that the non-encapsulated B. longum (in saline solution) added into the T2 could not survive in the meat mixture after 4 days of fermentation. After 22 days of ripening the viable counts of the bifidobacteria were found at 2.83 and 3.17 Log CFU/g for the T1 and T2, respectively and no statistical differences were found between these two treatments. When compared to the viable counts of probiotic B. longum in the fermented meat products in the present study, other researchers have reported higher number of added probiotic bifidobacteria (6.50–7.55 Log CFU/g) in dairy products (Albenzio et al., 2013; Song et al., 2017). The probiotic bifidobacteria are known to sensitive to the unfavorable conditions (e.g., high salt content or acidity) (Gandhi and Shah, 2015; Wang et al., 2015), and the viabilities of encapsulated bifidobacteria were significantly reduced in fruit juices or after freezing storage (Amine et al., 2014; Wang et al., 2015). This study for the first time, the bifidobacteria were encapsulated and added as a functional starter culture in the meat products, and the results confirmed that a certain number (approximately 50%) of encapsulated probiotic B. longum could survive in the final products. The intake of probiotic bifidobacteria or foods containing these probiotic bacteria has been associated with a variety of health-promoting benefits (Sarkar and Mandal, 2016). Moreover, fermented sausage is the most popular and widely consumed meat product in many countries (Oliveira et al., 2018). Therefore, further solutions are needed to improve the survival rate of the probiotic bifidobacteria in the fermented sausages.
APC is generally used as an important indicator indicating the microbiological quality of food products during processing and storage. Results showed that the APC showed significant differences among the treatments on all days examined, and all the control and treated samples significantly increased in the APC with increased ripening time, except the treatment with CSC which showed a decrease. At the end of ripening, the samples made with CSC had the lowest APC (5.81 Log CFU /g), followed by the T2 (7.87 Log CFU/g), while the T1 (encapsulated B. longum) and the control had the highest APCs (approximately 8.6–8.7 Log CFU/g). The lower APC in the samples made with CSC or encapsulated B. longum (T2) could be related to their higher acidic environment (as indicated by lower pH in Table 1) because pH in an important quality trait, and in order to be considered “shelf-stable” the ultimate pH of finished products must be 5.3 or lower (Ba et al., 2016). Chaves-Lopez et al. (2015) also reported the APC of about 8–9 Log CFU/g for the same product type ripened for 28 days.
The levels of TBARS and biogenic amine in the samples determined at the end of ripening are shown in Fig. 1A and Fig. 1B, respectively. Regarding the TBARS content, its values significantly (p<0.05) differed among the treatments, in the following order: CSC>Control>T1 (encapsulated B. longum)>T2 (encapsulated B. longum+B. longum in saline), being their mean values of 1.42, 2.53, 1.11, and 0.99 mg MDA/kg sample, respectively. This means that the treatment with CSC presented the highest lipid oxidation level, followed by the control. These results agree with the findings of Ba et al. (Ba et al., 2016; Ba et al., 2017). Interestingly, both the treatments (T1 and T2) with the encapsulated B. longum presented the lowest TBARS contents in comparison to the control or CSC treatment, suggesting that the oxidation of lipid probably was inhibited by the added B. longum in these samples. Regarding this, previous studies have also reported the strong antoxidation activities of Bifidobacterium species isolated from infant’s feces (Kim et al., 2003). When compared to the TBARS levels (1.5–3.16 mg MDA/kg) reported for the same product types (Ba et al., 2016; Chaves-Lopez et al., 2015), the samples made with the encapsulated B. longum (T1 and T2) presented much lower values (0.99–1.11 mg MDA/kg sample). Since the lipid oxidation has been found associated with quality deterioration and health risks (Grun et al., 2006), the inoculation of the probiotic B. longum therefore could enhance the lipid oxidation stability of the fermented meat products.
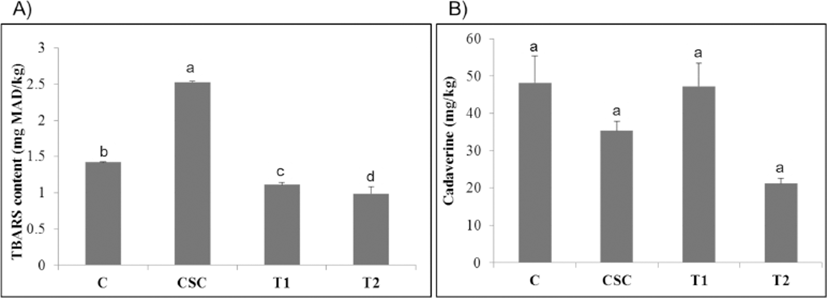
It has been reported that the ingestion of foods containing high amounts of biogenic amines can cause hazard to consumer’s health (Latorre-Moratalla et al., 2012). In the present study, cadaverine was the unique biogenic amine found in all the samples at the end of ripening (Fig. 1B). Our finding agrees well with that of Latorre-Moratalla et al. (2017), who reported that cadaverine is the most frequently found biogenic amine in dry fermented sausages. The levels of the amine were 48.27, 47.26, 35.30, and 21.23 mg/kg for the control, T1 (encapsulated B. longum), CSC and T2 (encapsulated B. longum+B. longum in saline), respectively and no statistical differences were found among the treatments (p>0.05). Histamine and tyramine are the main dietary biogenic amines associated with health hazard (Latorre-Moratalla et al., 2012), however, none of them were found in all the samples in the present study, probably due to their absences or produced levels were too low under the detection limit. In general, the levels of the cadaverine detected in all the samples in the present work fell within the limits (17.12–50 mg/kg) for fermented sausages by Sun et al. (2016) and Ba et al. (2017), but were much lower than the levels (100–250 mg/kg) reported by Tabanelli et al. (2012). Although the cadaverine itself has been proven as a non-toxic compound however its presence may enhance the toxicity of other biogenic amines (Ruiz-Capillas and Jimenez-Colmenero, 2005). The biogenic amines are mainly produced from the bacterial decarboxylation of amino acids (Latorre-Moratalla et al., 2012) and a variety of microbial starter cultures such as LAB, enterococci and staphylococci etc. have been reported as the biogenic amines producers (Tabanelli et al., 2012). From the obtained results, therefore, it may be said that the B. longum is not an amines-producing LAB strain.
The color and textural traits of fermented sausages inoculated with encapsulated B. longum measured at 22nd day of ripening are presented in Table 3. The samples added with encapsulated B. longum (T1 and T2) presented L* (lightness), a* (redness) and b* (yellowness), Chroma and hue angle - values of 49.79–51.29, 12.65–13.86, 8.27–9.48, 15.12–16.79 and 33.14–34.40, respectively. Of which the values for lightness, redness and yellowness were not significantly different from those of the control or the treatment with CSC (p>0.05). Additionally, the values of lightness, redness and yellowness in all the samples were almost similar to those reported for the same product type added with other CSCs (Ba et al., 2016). Contrastingly, Lorenzo et al. (2013) reported higher values while Ba et al. (2017) reported lower values for all these color traits of the fermented sausages samples. These contrasting results could be related to the differences in formulations, conditions and starter contents used among the studies. From the obtained results it might be said that the addition of B. longum did not cause any defects in the color characteristics of the final products.
Regarding the textural profiles, our results showed that except the hardness, the values for the other remaining traits such as cohesiveness, springiness, gumminess and chewiness in the samples added with the encapsulated B. longum (T1 and T2) were not significantly different from those of the control or the treatment with CSC (p>0.05).
In the present study, the total fatty acid compositions were determined in order to characterize and determine whether the added B. longum affects the lipolysis that alters the fatty acid compositions of the final products. The fatty acid profiles are presented in Table 4. The outcome of our analysis showed that fourteen fatty acids were detected on all the samples. Significant differences in levels of some fatty acids including: stearic acid (C18:0), linolenic acid (C18:3n3), erucic acid (C20:1n9) and arachidonic acid (C20:4n-6) occurred among the treatments (p<0.05). In particular, the level of the C18:0 was highest in the control samples whereas it was lowest in the samples inoculated with encapsulated B. longum (T1 and T2) or CSC. While, the polyunsaturated fatty acids (PUFA) such as C18:3n3 and C20:4n6 whose levels were significantly higher in the samples inoculated with encapsulated B. longum (T1 and T2) in comparison to the control or CSC treatment (p<0.05). On the other hand, the total unsaturated fatty acids (UFA) and n-6/n-3 ratio also showed differences among the treatments, with higher UFA levels were found in the samples made with B. longum (T1 and T2) than the control (p<0.05). From the point of view of health and nutrition, the UFA especially PUFA (e.g., C18:3n3) exert the significant effects on physiological processes and human health (Jump, 2002). According to the recommendations for the healthy diet by the American Heart Association (Krauss et al., 1996), the lower the n-6/n-3 fatty acids the healthier the diet. Our analysis showed that the n-6/n-3 ratio was significantly lower in the samples made with encapsulated B. longum (T1 and T2) than that in the control or the treatment with CSC (p<0.05). Fatty acids in fermented sausages are derived mainly from the muscle tissues and fat, and though the same raw materials, formulation and production conditions were used for all the treatments in the present study, however, the differences in levels of the fatty acids still occurred among them. This could be due to the differences in lipolysis activities by the microbial lipase (Chen et al., 2017) and lipid oxidation degrees (Fig. 1A) among the treatments during fermenting/ ripening process, and the samples made with encapsulated B. longum (T1 and T2) presented the more desirable fatty acid profiles than those inoculated with CSC.
Table 5 shows the scores for the color, odor, taste and acceptability of the samples made with probiotic B. longum at the end of ripening. Results showed that the panelists gave significantly (p<0.05) higher scores for color and odor traits for the inoculated samples than for the non-inoculated control. Especially, the treatments with B. longum (T1 and T2) had the color, odor and taste scores comparable to those of the ones made with CSC. Regarding the acceptability, the similar scores were given for the samples added with B. longum (T1 and T2) and those added with CSC (p>0.05). Only the treatment that showed significantly (p<0.05) higher acceptability score than the non-inoculated control was T2 (0.02% encapsulated B. longum+0.02% B. longum in saline solution). This could be associated with the synergistic effects of their higher odor and taste scores. From the obtained results, it may be said that the inoculation with the probiotic B. longum did not cause adverse effects whereas it partially improved the eating quality of the final products.
Conclusion
The bifidobacteria were encapsulated and added as a functional ingredient and starter culture for production of functional fermented sausages. The inoculation with B. longum resulted in the greater acidifying activity compared to the non-inoculated control but lower than the CSC. In general, the application of encapsulation successfully preserved approximately a half of bifidobacteria viability in the final products. It was observed that the products made with B. longum showed lower lipid oxidation levels, and presented more desirable fatty acid profiles. Especially, the addition of the B. longum partially improved the eating quality, without adverse effects on technological quality of the final products. However, further study on the modifications of encapsulation technology or formulations (e.g., reducing salt level and increasing the initial added count of bifidobacteria) and processing condition etc. is needed in order to improve the viability of the probiotic bifidobacteria, thus improving the functionality of the final fermented meat products.