Introduction
In the context of public health, it is of great importance to control microorganisms during the industrial production and distribution of hatching and table eggs. Hygienic management of hatching and table eggs is also crucial for economic profit and loss. Microbial contamination of hatching eggs is known to cause problems for the poultry industry such as the production of chicks with low productivity, low hatchability and disease spread (Quarles et al., 1970; Reid et al., 1961; Samberg and Meroz, 1995). Board and Tranter found bacterial contamination on the surface of hatching eggs could reach 2 to 7 Log colony-forming units (CFU) per egg (Roberts, 2004). Outbreaks of food poisoning caused by contaminated table eggs have become a global public health issue. According to the Centers for Disease Control and Prevention (CDC, 2010), in the United States, 1,939 cases of food-borne disease linked with egg contamination were reported from May 1 to November 30, 2010. Among the causes of food-borne illnesses in Europe, contaminated eggs accounted for 21% of the total cases between 2004 to 2012. Similar instances of food poisoning caused by contaminated eggs have been reported in Austria, France, and Germany; in these instances, the eggs were distributed by the same egg packaging center in Germany (European Center for Disease Control and Prevention/European Food Safety Authority [ECDC/EFSA], 2014). Also, bacterial contamination was found on the surface of eggs collected in Korea, from a market (Chun and Hong, 2009).
In Korea, conventional disinfection (formaldehyde fumigation, UV irradiation after washing) of eggshell surfaces is used to prevent the spread of diseases and economic loss. Fumigation with formaldehyde is usually used for disinfection of hatching eggs, due to its advantages, such as economic use and highly effective as a bactericide, virucide, and sporicide. Conversely, the International Agency for Research on Cancer (IARC, 2006) has classified inhaled formaldehyde as a known human and animal carcinogen, causing serious health problems for hatchery workers (Baker and Balch, 1962; McDonnell and Russell, 1999; Whistler and Sheldon, 1989). UV irradiation after washing is another effective strategy to reduce the microbial load on eggshells, particularly for table eggs. However, the washing step can cause potential damage to the egg’s cuticle layer, and UV irradiation only inactivates microorganisms on eggshells directly exposed to the rays (De Reu et al., 2006a; EFSA, 2005).
Therefore, it is necessary to develop a new, suitable alternative to conventional hatching and table egg disinfection methods. In this study, the use of chlorine dioxide (ClO2) gas was tested as an alternative to the current disinfection procedures. ClO2 is a yellow, water-soluble gas with a strong oxidative activity (Fukayama et al., 1986; Moran et al., 1953). Furthermore, it has proved to be a rapid, effective disinfectant for bacteria, yeast, molds and viruses (Kim et al., 2016; Lowe et al., 2013; Morino et al., 2009; Sy et al., 2005). In addition, ClO2 holds promise as a disinfecting agent that can be applied to food surfaces (Gómez-López et al., 2007; Han et al., 2001; Kim et al., 2016; Lee et al., 2004; Prodduk et al., 2014).
Consequently, the efficacy of ClO2 gas to reduce the bacterial load on eggshell surfaces was compared to conventional disinfection methods. Also, egg quality was monitored during storage, to determine whether ClO2 gas caused deterioration. Finally, the hatchability of eggs treated with ClO2 gas exposure and formaldehyde fumigation was compared.
Materials and Methods
Table and hatching eggs were collected for the comparison of the bacterial reduction efficacy of ClO2 gas use on eggshells. Table eggs (n=120) were taken from an egg grading and packing facility. The samples were divided into four groups: control (untreated), UV treatment (UV irradiation after washing), and 20 and 40 ppm ClO2 gas exposure. UV treatment samples (n=30) were collected at the point of the egg processing procedure just after washing and UV irradiation (radiation: 10 mW at 253.7 nm, residence time: 4.5 s). The other samples were collected before the washing process. The egg surface was treated with ClO2 gas for 30 min.
A total of 120 hatching eggs were taken from a hatchery (Korea). Each group of hatching eggs was treated with formaldehyde fumigation (40 mL of 40% formalin+39 mg KMnO4), and 20 and 40 ppm ClO2 gas exposure, for 20 min. The untreated group was used as a control. Thirty hatching eggs were collected, just after fumigation by formaldehyde and the others were collected before the fumigation process. All of the samples were packed to avoid additional contamination before transfer, to a laboratory at 4°C, within 3 h. To detach bacteria from the eggshell, the eggs were aseptically placed in a plastic bag with 50 mL sterile distilled water (DW) and agitated on an orbital shaker for 10 min. After shaking, 1 mL of the DW was taken and serially diluted 10-fold. The total aerobic plate count (APC) was counted using 3M PetrifilmTM Aerobic Count Plates (3M, USA). One milliliter of the appropriately diluted samples was placed on the bottom film of the plates. The bacterial colonies were counted by the plate count method after incubation at 37°C for 24±2 h. The results(Log CFU/eggshell) were expressed as mean and standard error of measurement (SEM).
To evaluate whether ClO2 gas deteriorated egg quality, 240 table eggs were used. This approach was also compared with existing commercial treatment (UV irradiation after washing), during storage under controlled temperature and humidity conditions. The average weight of all collected samples was 64.16±3.07 g. Table egg samples were collected immediately before and after the washing and following the UV irradiation process. The samples were divided into three treatment groups: control (untreated), UV with washing, and 40 ppm ClO2 gas, all followed by storage under dry (35±2% RH at 25°C) and high humidity (80±2% RH at 30°C) conditions. The quality changes were evaluated at regular intervals (7 d) for 21 d. Eggshell strength and thickness were measured by using a shell breaking strength tester and FHK shell thickness gauge (Fujihira Ltd., Japan) as external egg quality traits. York color was evaluated by Roche yolk colour fan (DSM, Switzerland). Egg albumen height and Haugh unit (HU) were determined using a quartz crystal microbalance (QCM device, Technical Services and Supplies (TSS), UK) for internal egg quality measurement of each treated group. This system uses the HU formula:
where HU=Haugh unit, H=albumen height (mm), and W=egg weight (g) (Roush, 1981).
All the eggs (N=450) collected from a hatchery (Korea) were divided into three groups (n=150), and the hatching of fertile eggs was evaluated. The control group (n=150) was exposed to formaldehyde fumigation (40 mL of 40% formalin+39 mg KMnO4) for 20 min, in the hatchery. The experimental groups were respectively exposed to 80 and 160 ppm ClO2 gas for 30 min (25°C, 80±2% RH) in the laboratory. The fertile rate, hatchability and hatching of fertile eggs were calculated using the following equations:
The ClO2 gas treatment system comprised three parts: gas generator, gas collecting chamber, and gas treatment chamber (30 L). The ClO2 gas was generated using a gas generator (CA-300, PurgoFarm Co., Ltd., Korea) used in a previous study (Kim et al., 2016). The highly pure ClO2 gas (>95% ClO2) was produced by passing aqueous NaClO2 through patented multi-porous membrane electrode assembly. The ClO2 gas was released after a sequence of electrochemical reactions and vented into a collecting chamber in the dark. The desired gas concentration in the treatment chamber was ensured, by using a C16 PortaSens II gas leak detector (Analytical Technology, USA). The experimental groups were exposed to ClO2 gas in the treatment chamber (80±2% RH at 25±2°C) for 30 min.
Analyses were performed using the SPSS statistical package for Windows (version 17.0, SPSS Inc., USA). All data were presented as mean±SEM. Analysis of variance (ANOVA) was performed, to compare the bacterial reduction effect and quality measurement results. The significant level was set at p<0.05. Experimental results were expressed using Prism 5 for Windows (version 5.01, GraphPad Inc, USA).
Results
We evaluated the APC reduction effect on table eggshells treated under three different disinfection conditions: UV irradiation after washing, and 20 and 40 ppm ClO2 (Fig. 1). The APC of the control group (untreated) was 5.97±0.03 Log CFU/eggshell. Following UV treatment, the APC was reduced by 3.51±0.14 Log CFU/eggshell. The APC results after 20 and 40 ppm ClO2 gas exposure were 4.08±0.10 and 3.16±0.10 Log CFU/eggshell, respectively. Nonetheless, all treated groups reduced more than 1.5 Log CFU/eggshell. The APC was reduced APC 2.46, 1.89 and 2.81 Log, by UV irradiation, and 20 and 40 ppm ClO2 gas exposure, respectively. The 20 ppm ClO2 treatment had a 1.89 Log higher reduction effect than the control, but 0.57 Log lower than the UV irradiation. However, the 40 ppm ClO2 gas treatment attained 2.81 and 0.35 Log higher reduction than the control and UV rays. The three experimental groups were significantly different from the control (p<0.001). The UV-treated and 40 ppm ClO2 gas-treated groups showed significantly different outcomes from the 20 ppm ClO2 gas-treated group (p<0.001). However, no significant difference in the bacterial reduction efficacy between UV treatment and 40 ppm ClO2 gas exposure was observed (p>0.05).
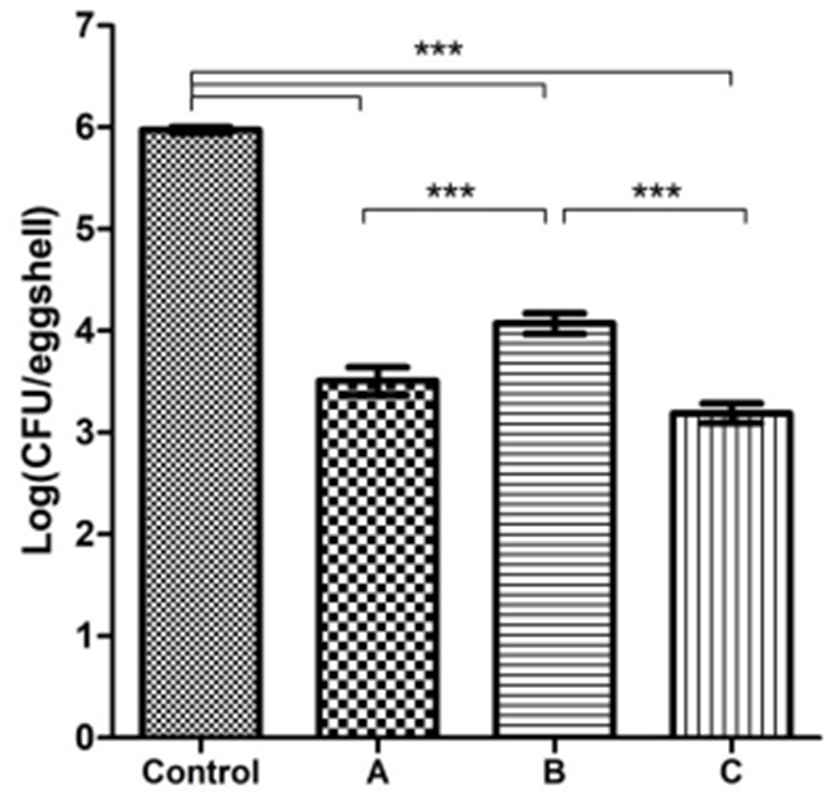
The APC reduction on the hatching egg surface (Fig. 2) revealed the formaldehyde fumigation and ClO2 gas (20, 40 ppm) treatments was 2.33±0.10, 3.38±0.11 and 2.06±1.66 Log CFU/eggshell, respectively, whereas the control group had 4.66±0.08 lag CFU/eggshell. Formaldehyde treatment achieved a 2.33 and 1.28 Log higher reduction efficacy than the control and 20 ppm ClO2 gas group, respectively. The 40 ppm ClO2 gas treatment resulted in 2.60 Log reduction effect, which was the highest value relative to the control. All treatment groups were significantly different from the control (p<0.001). In addition, both the formaldehyde fumigation and 40 ppm ClO2 gas groups, which had similar efficacies (p>0.05), were superior to the 20 ppm ClO2 gas group (p<0.001).
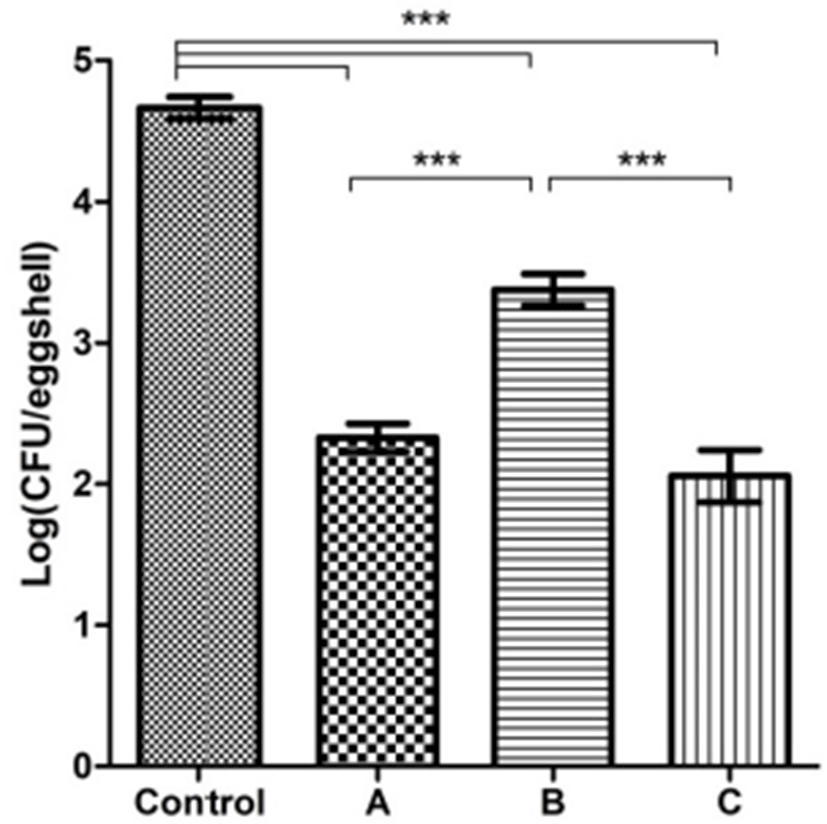
Data on the internal quality (albumen height, HU, yolk color) of table eggs stored under high temperature and high humidity (30°C, 80±2% RH; Table 1) versus relatively lower temperature and dry conditions (25°C, 35±2% RH; Table 2) were compared. Under the high temperature condition, the albumen height of all groups decreased throughout the storage (Table 1). In the control group, albumen height decreased from 9.86 (0 d) to 2.57 (21 d), whereas this decline was less rapid following UV treatment from 8.59 (0 d) to 2.49 (21 d). The albumen height of the 40 ppm ClO2 gas group reduced from 9.63 (0 d) to 3.31 (21 d), presenting a similar trend to the other groups. Although its value at the end of storage was higher than the control (2.57±0.44) and UV treatment group (2.49±0.19), the differences among the groups at 21 d were not significant (p>0.05). Storage under the dry conditions (25°C, 35±2% RH; Table 2), resulted in similar decreasing trends in albumen height data to that at high temperature and increased humidity. Albumen height fell from 9.39 (0 d) to 2.92 (21 d) in control group. Both the UV irradiation and 40 ppm ClO2 gas treatment showed similar trends to the control, with no significant differences among the results.
In all groups, the HU had a decreasing tendency throughout both storage conditions (Tables 1 and 2), similarly to the albumen height. The control and the UV treatment group values decreased from 96.80 (0 d) to 26.27 (21 d) and from 91.66 (0 d) to 34.49 (21 d), respectively. The HU of the 40 ppm ClO2 gas group (46.69±3.44 at 21 d) was higher than the control (26.27±9.42) and UV treatment group (34.49±3.53), displaying a slightly slower rate of decline than the other groups, although it was not significant (p>0.05). Regarding yolk color, there were no significant differences among all treatment groups during the entire storage period, irrespective of the storage conditions (p>0.05). Likewise, there were no prominent changes in the external qualities of table eggs under both storage conditions. Both eggshell strength and thickness increased among all treatment groups as storage progressed, and no significant differences occurred (Tables 3 and 4; p>0.05).
The fertility rate of all groups was over 94% (Table 5). Among groups, the control (formaldehyde fumigation) and 80 ppm ClO2 exposure resulted in more than 84% hatchability, whereas, the 160 ppm ClO2 group had the lowest values (Table 5). The hatching of fertile eggs was similar between the control and 80 ppm ClO2 treatment (p>0.05).
Discussion
Table egg quality control and hygienic management are important throughout the entire production stage to delivery to consumers. Although diverse methods are used throughout the world to eliminate egg surface contaminants and control microorganisms, incidences of diseases due to microbial contamination of eggs have been consistently occurring (CDC, 2010; ECDC/EFSA, 2014; Ghasemian Safaei et al., 2011). In the USA, Australia, and Japan, visible contaminants on the surface of eggs are removed in the washing stage, during egg production (Chousalkar et al., 2010; Hutchison et al., 2003). Besides, microorganisms present on the egg surface are controlled using short-time UV irradiation (Chavez et al., 2002; Coufal, 2003; De Reu et al., 2006a; EFSA, 2005). However, UV irradiation has disadvantages, in that the disinfecting efficacy is reduced in the presence of organic substances and, as mentioned above, only the area directly exposed UV rays is affected (De Reu et al., 2006a). Although in Korea, table eggs typically undergo the UV irradiation after washing, bacterial contamination levels exceeding 104 CFU/mL have been documented on the shells of table eggs distributed in hypermarkets in the country (Chun and Hong, 2009). Bacteria contamination at more than 105 CFU/mL on egg conveyor belts, collection equipment and other incompletely disinfected eggs has been widely reported in various studies (De Reu et al., 2008; ECDC/EFSA, 2014; EFSA, 2005; Hutchison et al., 2003). Moreover, contamination of egg surfaces affects the degree of microbial invasion (De Reu et al., 2006b, De Reu et al., 2008).
In earlier works, UV irradiation reduced APC by 1–2 Log and Escherichia coli and 3–4 Log for Salmonella. spp.. with no effect on the inside of eggs, even at high intensities of irradiation (Chavez et al., 2002; Coufal, 2003; De Reu et al., 2006a). In present study, 40 ppm ClO2 gas treatment reduced the APC to a similar extent as that of UV irradiation. However, the washing process, required to remove fecal and visible contaminants before UV irradiation, has been known to physically damage the cuticle layer of the egg surface, thereby allowing the invasion of microorganisms and affecting the internal quality (Chousalkar et al., 2010; EFSA, 2005; Hutchison et al., 2003). In our earlier study, application of 40 ppm ClO2 gas reduced Salmonella enterica and Salmonella gallinarum more than 4 Log (Kim et al., 2016). In the current investigation, the total APC of the untreated egg surfaces was 5.9 Log, which was decreased 1.8 and 2.8 Log, by the 20 and 40 ppm ClO2 gas treatments, respectively. The use of ClO2 gas as an alternative disinfectant has the advantages of preventing damage to the cuticle caused by washing during the egg production process, disinfecting all eggs without cross-contamination, and having similar disinfection efficiency to existing methods (Lee et al., 2004).
Commonly, eggs are sanitized before being sold to the market, and their quality could be altered by artificial exposure to chemical disinfectants. The current work evaluated both, internal and external quality, respectively, to investigate whether ClO2 gas affects egg quality and freshness. Egg quality includes both the internal (e.g., yolk color, albumen quality) and external (e.g., shell breaking strength, shell weight, shell thickness) characteristics (Roberts, 2004). Proper measures of the internal quality and freshness of eggs are known be albumin height, HU, and yolk color (Caner and Cansiz, 2008; Roberts, 2004). These internal egg quality traits decreased under both room and high temperature storage conditions. In particular, albumin height and HU had lower values and declined more rapidly under the high temperature and humidity environment (30°C, 80±2%) when compared with room conditions (25°C, 35±2%). Internal egg quality is known to be greatly affected by the storage period and temperature (Caner and Cansiz, 2008; Jones and Musgrove, 2005; Roberts, 2004). During storage, although no statistically significant difference in changes in internal egg quality (HU, albumen height) occurred among the control, UV irradiation, and ClO2 treatment groups, the ClO2 treatment tended to show lower rates of reduction compared to the other groups under high temperature conditions. No ClO2 gas residues have been previously detected inside eggs, but ClO2 gas is known to maintains the quality of vegetables and extends storage durations and chemical reactions in food (Fukayama et al., 1986; Gómez-López et al., 2007). Moreover, ClO2 has a boiling point of 11°C at 760 mmHg, and thus, according to Dobson and Cary (2002), no residual ClO2 should remain inside eggs after gas spraying at room temperature. Nevertheless, the exposure of ClO2 gas could affect egg compounds related to taste and quality.
The external egg quality is known to be affected more by laying hens than by storage conditions or disinfection methods (De Reu et al., 2006b; Roberts, 2004). We did not observe significant differences in the external egg quality traits (eggshell thickness and strength), among the control, UV rays-, and ClO2 gas-treated groups. In this context, ClO2 gas can be used as an alternative disinfectant without significant adverse effects on the internal and external qualities of eggs.
Hygienic processing of hatching eggs is important for the entire poultry industry. The hatchery environment has a suitable temperature and humidity for microbial growth and owing to the various equipment, such as chicken boxes and fiber egg trays, it is not easy to clean (Braswell et al., 1970). At the time of laying, the natural bacterial load on an eggshell may range from 300 to 500 CFU (Mauldin, 2008). This number may increase rapidly: 1 h after an egg is laid, there can be 20,000–30,000 bacterial cells present (North and Bell., 1990; Samberg and Meroz, 1995). Typical contaminating microorganisms are among the genera Micrococcus, Salmonella, Pseudomonas, and Escherichia(Mayes and Takeballi, 1983). It has been demonstrated that if hatching eggs are not disinfected before incubation, residual excessive bacterial contamination can reduce hatchability. Quarles et al. (1970) noted a negative correlation between the contamination of hatching eggs and hatchability: cross-contamination of hatching eggs was associated with reduced hatchability. Although a contaminated egg can be hatched, the productivity of the hatchery may decrease due to indirect influences, such as poor quality of chicks, inadequate growth and performance, and increased post-hatch mortality (Reid et al., 1961). Thus, economic losses could increase if eggs are hatched in poor sanitation conditions. Formaldehyde fumigation is mainly used for hatchery disinfection because it is known to destroy a wide range of microorganisms such as bacteria, viruses and fungi (Baker and Balch, 1962; Braswell et al., 1970; Kim and Kim, 2010; McDonnell and Russell, 1999; Whistler and Sheldon, 1989; Williams, 1980). In current work, the APC of the untreated group was at least 4 Logs. The formaldehyde fumigation and the 40 ppm ClO2 gas treatment showed similar bacterial reduction effects to each other, with APCs of 2 Logs or higher (Fig. 2). Additionally, when the ClO2 gas exposure (80 ppm) was compared with the formaldehyde fumigation as a control, the hatchability results were analogous to each other (Table 5). The application of 160 ppm ClO2 gas in the present study is similar to the use of 100 ppm ClO2 aqueous solution, used in a previous investigation that led to reduced hatchability (Samberg and Meroz, 1995). We evidenced that the practical use of ClO2 gas at a constant concentration (80 ppm) on hatching eggs could be equally effective as formaldehyde fumigation.
Although formaldehyde fumigation can be used easily, economically, and widely, as a conventional method of egg disinfection, it may be dangerous to workers. According to the Centers for Disease Control and Prevention, direct exposure to formaldehyde could cause symptoms, such as irritation of the eyes, nose, and throat, with tearing. The United States Occupational Safety and Health Administration has set the formaldehyde concentration use at 0.75 ppm (8 h) and 2 ppm (within 15 min) (Harvey Jr, 2015). To prevent toxic effects of formaldehyde fumigation, the chemical residue must be neutralized and removed, using 25% ammonium hydroxide solution (Samberg and Meroz, 1995). When used at high concentrations in eggs, formaldehyde can lower hatchability and damage the cuticle layer of egg surfaces (McDonnell and Russell, 1999; Whistler and Sheldon, 1989). Conversely, ClO2 gas has been studied in various fields, such as fruits, vegetables, and space, and can be easily removed with proper ventilation, without additional neutralization steps (Han et al., 2001; Kim et al., 2016; Lee et al., 2004; Lowe et al., 2013; Morino et al., 2009; Prodduk et al., 2014). Hence, disinfection with gaseous ClO2 can be considered as a safe alternative to formaldehyde fumigation, in hatcheries.
In conclusion, disinfection with ClO2 gas could be regarded as a safe and effective way to control microorganisms on the surfaces of both hatching and table eggs. Moreover, no negative effects on egg quality, and no ClO2 residual inside and outside of eggs after disinfection were evidenced. As a further study, evaluation of ClO2 gas efficacy on bulk of eggs should be conducted in the practical workplace.