Introduction
Emulsions are complex and thermodynamically unstable systems consisting of two immiscible phases (Perrier-Cornet et al., 2005; Zhao et al., 2009). There are two types of emulsions, namely, oil-in-water (O/W) and water-in-oil (W/O). The oil-in-water emulsion is a system that is made up of oil droplets dispersed in a continuous water phase, whereas the water-in-oil emulsion is a system that is made up of water droplets dispersed in a continuous oil phase (Pal, 2011). Emulsifiers are compounds that facilitate the formation of emulsions and stabilize the emulsion droplets, and consequently, are widely employed in different industries (Rouimi et al., 2005). Emulsifiers that have hydrophilic and hydrophobic groups can be characterized by hydrophilic-lipophilic balance (HLB) values. Emulsifiers with low HLB values (<7) stabilize water-in-oil emulsions, whereas emulsifiers with high HLB values (>7) stabilize oil-in-water emulsions (Pichot et al., 2010).
Sucrose fatty acid ester (SFAE) is a non-ionic small-molecule emulsifier that contains a hydrophilic sucrose group and one or more fatty acids as the lipophilic group (Szűts and Szabó-Rèvèsz, 2012). The HLB values of SFAE can be modulated by attaching different numbers and types of fatty acids to the sucrose moiety. Therefore, their HLB values can range from 1 to 16. SFAE is widely used in the pharmaceutical and cosmetics industries (Cheng et al., 2016; Choi et al., 2011). It is also produced from natural resources such as sucrose and vegetables, and have low toxicity, good taste, and high biodegradability. SFAE is increasingly being used in food and beverage industries as an emulsifier (Ariyaprakai et al., 2013).
Homogenization is a widely used process in food, pharmaceutical, and biotechnology industries that allows the mixing of two immiscible phases. The intense disruptive forces of homogenization can break down fat globules and improve the stability of emulsions by reducing the creaming rate. Homogenization not only reduces the droplet size but also deflocculates the clusters of fat globules and distributes the droplets uniformly (Floury et al., 2000; Heffernan et al., 2009). The effect of homogenization on emulsions has been reported in studies that have mostly focused on high-pressure or ultra-high-pressure homogenization (Floury et al., 2000; Lee et al., 2009; Roach and Harte, 2008). However, Perrier-Cornet et al. (2005) pointed out that ultra-high-pressure homogenization has side effects such as increased product temperature, valve corrosion, and high operating costs. Consequently, in dairy processing plants, conventional pressure homogenization (no more than 50 MPa) is still widely used for the industrial production of dairy products.
Milk-based products, such as dairy cream, butter, and ice cream, are oil-in-water or water-in-oil emulsions. Dairy cream is a representative dairy product of oil-in-water emulsion with a high milk fat content (typically 30–40%) and is prepared from milk by centrifugal separation (Hussain et al., 2017). Dairy cream is generally used to produce various food products such as cakes, soups, and creamy beverages. However, it is unstable because of its high milk fat content, which can result in creaming, coalescence, and flocculation (Long et al., 2012; Tual et al., 2006; Zhao et al., 2009). To improve the stability of creams, several studies have been conducted to evaluate the effect of emulsifiers such as sorbitan monostearate (Zhao et al., 2013), glycerol monostearate (Wu et al., 2016), and Tween 80 (Hussain et al., 2017). The objective of this study is to investigate the effect of different SFAE concentrations (0.08%, 0.16%, and 0.24% w/w) on the droplet size distribution, rheological properties, and stability of the dairy cream-based emulsions at different conventional homogenization pressures (10 MPa and 20 MPa).
Materials and Methods
Dairy cream was obtained from Seoul Dairy Cooperative (Korea). Cream was prepared by concentrating the milk by centrifugal separation. It was pasteurized without any mechanical treatment or adding other ingredients, and the final milk fat and protein contents were 38% and 2%, respectively. The SFAE (DK ESTER-F160) supplied by Dai-Ichi Kogyo Seiyaku Co., Ltd. (Japan) is used as an emulsifier. The fatty acids of the SFAE used in this study were composed of palmitic acid and stearic acid, and the HLB value of SFAE was in the range of 15–16. Sodium azide (Sigma-Aldrich Chemical Co., USA) was also used to inhibit the growth of microorganisms during storage.
The emulsions were comprised of 50% (w/w) dairy cream, 0.02% (w/w) sodium azide (as an antimicrobial agent), distilled water, and SFAE (0.08%, 0.16%, and 0.24% w/w). As it was difficult to dissolve the SFAE in water, distilled water was pre-heated in a water bath at 70°C for 30 min. The dairy cream was also pre-heated at 60°C to minimize protein denaturation. SFAE and sodium azide were added to the pre-heated distilled water and mixed using a stirrer (Eurostar 20 High speed digital stirrer, IKA®, Germany) at 2,000 rpm for 1 min. Next, the pre-heated dairy cream was added slowly into the aqueous phase with SFAE and sodium azide, and the mixture was allowed to mix at 2,000 rpm for 5 min. Homogenization was carried out using a two-stage valve homogenizer (APV-1000, Invensys APV, Denmark) at two different homogenization pressures (10 MPa and 20 MPa), and 20% of the total pressure was maintained in the second stage valve. Finally, the homogenized emulsions were immediately placed in ice water for 30 min and stored overnight at 5°C. The control sample did not contain any SFAE and was not homogenized.
The droplet size distribution of the dairy cream-based emulsions was determined using a laser light scattering droplet size analyzer (Mastersizer 3000, Malvern Instruments Ltd., UK). The emulsions were added to distilled water until an obscuration rate of 5–15% was achieved with stirring at 1,000 rpm. The absorption coefficient was 0.01, and the refractive indexes of milk fat and water were 1.462 and 1.330, respectively. The D[4,3], D[3,2], Dv10, Dv50, and Dv90 values were used to interpret the droplet size distribution, and calculated using Malvern software (version 3.20, Malvern Instruments Ltd., UK). The D[4,3] value is the volume-weighted mean that is defined as the average diameter calculated on a volume basis, and the D[3,2] value is the surface-weighted mean that is defined as the average diameter calculated on a surface basis. Dv10, Dv50, and Dv90 refer to the average droplet sizes corresponding to the cumulative distributions at 10%, 50%, and 90%, respectively.
The rheological properties of the dairy cream-based emulsions were determined using a rheometer (HAKKE Roto Visco-1, Thermo Fisher Scientific, Germany) with a plate-plate system (35 mm in diameter with a gap of 500 μm). Steady shear rheological properties were determined over a shear rate range of 0.4–100 s–1. To describe the steady shear rheological properties of the emulsions, the data were fitted to the well-known power law model (Eq. (1)).
where σ is the shear stress (Pa),
Dynamic shear data were obtained from frequency sweeps over a range of angular frequencies (0.63–62.8 rad s–1) at 2% strain. Haake Rheowin software (version 4.41.0000, Thermo Fisher Scientific, Germany) was used to collect the rheological data and to calculate the storage modulus (G') and loss modulus (G"). The G' value is a measure of elastic response that is recoverable, and the G" value is a measure of viscous response that is lost as viscous dissipation. In order to relax the samples prior to the steady and dynamic shear rheological measurements, all samples were allowed to rest on the plate at 4°C for 5 min. All rheological measurements were performed in triplicate at 4°C.
To measure the stability of the dairy cream-based emulsions, they were transferred to 50 mL conical tubes and stored at two temperatures (5°C and 40°C). Samples (15 mL) were collected from the top and bottom of the emulsions and their stabilities were evaluated by measuring the droplet size and distribution on day 7, day 15, and day 30.
Results and Discussion
The effect of homogenization pressure and SFAE concentration on the droplet size distribution of dairy cream-based emulsions is shown in Fig. 1 and Table 1. The D[3,2] and D[4,3] values of the control were 2.32 µm and 3.32 µm, respectively. In contrast, all homogenized emulsions had lower D[3,2] values (0.84–1.15 µm) and D[4,3] values (1.02–1.49 µm). Furthermore, as shown in Fig. 1, all homogenized emulsions had a smaller droplet size and narrower droplet distribution than those of the control. These results were found to be in good agreement with those of previous studies that investigated the effect of homogenization on these parameters (Lee et al., 2009; Heffernan et al., 2009). Our results could be explained by the disruptive forces occurred during homogenization. The intense turbulence and shearing forces, which were generated when the coarse emulsions passed through the interaction chamber in the homogenizer, led to the breaking up of larger droplets into smaller droplets (Floury et al., 2000; Long et al., 2012).
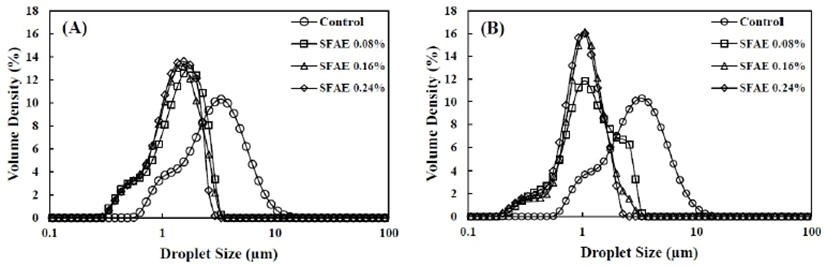
The D[4,3] value demonstrated that the droplet size of the emulsions homogenized at 20 MPa was smaller (1.02–1.28 µm) than that of the emulsions homogenized at 10 MPa (1.35–1.49 µm). However, the emulsion homogenized with 0.08% (w/w) SFAE at 20 MPa showed a wide distribution with a low Dv10 value of 0.53 µm and a high Dv90 value of 2.31 µm. In this case, an asymmetrical droplet size distribution with a shift towards larger droplets was also observed (Fig. 1B), which indicated that some of the milk fat droplets that broke during homogenization had re-flocculated. A high homogenization pressure decreased the droplet size and increased the newly formed surface area. The homogenized small droplets were rapidly re-flocculated or protected from aggregation by absorbing proteins and emulsifiers on the newly formed surface area (Jafari et al., 2004). Consequently, as the homogenization pressure increased and the droplet size decreased, the newly formed surface area became larger and required more proteins and emulsifiers to be absorbed on the droplet surface (Lee et al., 2009; Heffernan et al., 2009). Therefore, in the case of the emulsion homogenized at a high pressure (20 MPa) with low SFAE concentration (0.08% w/w), the partial flocculation of milk fat could be attributed to the lack of proteins and SFAE, which completely covered the large surface area of the newly formed milk fat droplets.
As the concentration of SFAE was increased, the D[4,3] and D[3,2] values decreased from 1.49 to 1.02 µm and from 1.15 to 0.84 µm, respectively (Table 1). This could be explained by the competition between the disruption and formation of fat droplets generated during homogenization. If the timescale of collision between the droplets was longer than the timescale of the adsorption of the emulsifier to the droplet surface, fat droplets would be re-flocculated and larger droplets will be formed (Jafari et al., 2004). Small-molecule emulsifiers such as SFAE would also be quickly absorbed at the newly formed interface, leading to further disruptions by reducing the interfacial tension (Leong et al., 2011; Pichot et al., 2010). Therefore, the addition of SFAE inhibited the re-flocculation of milk fat droplets in dairy cream-based emulsions during homogenization and thus prevented the phase separation of the initial emulsion.
The shear stress (σ) versus shear rate (γ
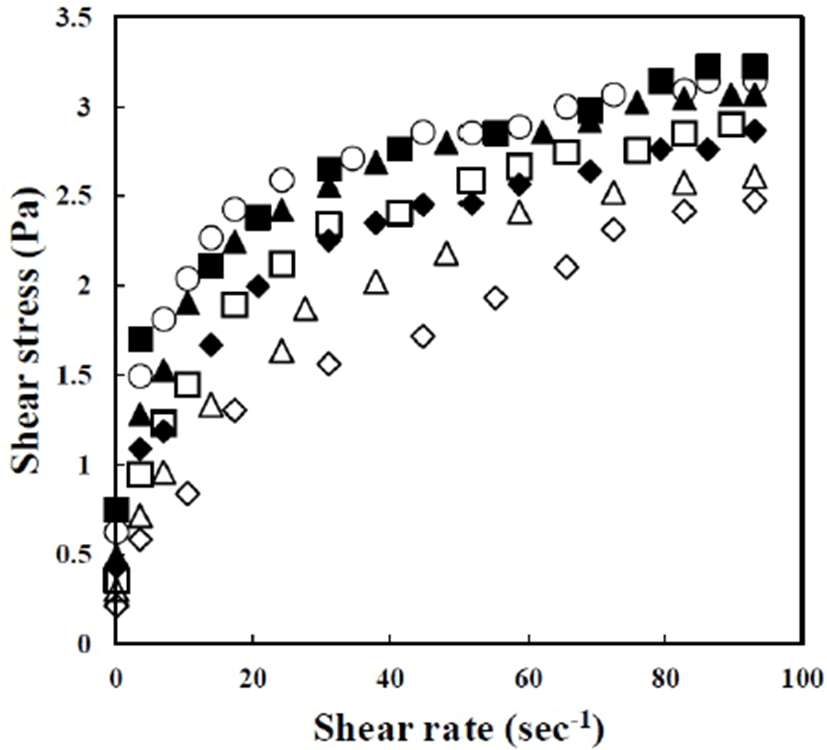
The ηa,10 values (0.10–0.21 Pa s) of the emulsions homogenized with SFAE were lower than that of the control (0.23 Pa s), and they decreased with increasing SFAE concentration and homogenization pressure. All emulsions had a lower K (0.35–1.06 Pa sn) than that of the control (1.16 Pa sn), except for the emulsion homogenized with 0.08% (w/w) SFAE at 10 MPa (K=1.28 Pa sn). At the same SFAE concentration, emulsions with a low homogenization pressure (10 MPa) had higher ηa,10 and K values than those of the emulsions with a high homogenization pressure (20 MPa). This result could be attributed to the lack of proteins and SFAE on the droplet surface. At a low homogenization pressure, the fat droplets are large and the surface of the newly formed droplets is small. Consequently, the relative concentrations of the aqueous proteins and SFAE increased at a low homogenization pressure and these emulsifiers formed micelles that led to an increase in the viscosity (Granger et al., 2005; Zhao et al., 2014).
Fig. 3 shows the changes in the storage modulus (G') and loss modulus (G") as a function of frequency (ω) for the dairy cream-based emulsions with different homogenization pressures (10 MPa and 20 MPa) and SFAE concentrations (0.08%, 0.16%, and 0.24% w/w). The G' and G" values increased with increasing ω, except for the G" of the emulsion homogenized with 0.08% (w/w) SFAE at 10 MPa. As the ω increased, the G' values increased more sharply than the G" values, indicating an increase in the elastic properties at a high frequency. The G' and G" values of the control were higher than those of all emulsions homogenized with SFAE. This is in good agreement with the results of Hussain et al. (2017); these authors found that the dynamic moduli (G' and G") values of the commercially available creams stabilized by sodium caseinate and Tween 80 decreased with the increasing homogenization pressure because of the differences in the coating layers of milk fat droplets. According to Derkach (2009), the rheological properties of the emulsions are affected by the surface properties of the fat droplets. During homogenization, the surface of the milk fat droplets is covered by proteins such as casein, and the adsorbed protein can form a casein gel matrix, thus resisting against deformation (Hussain et al., 2017; Murray, 2002). Accordingly, the decrease in G' and G" for emulsions homogenized with SFAE can be attributed to these emulsifiers being absorbed on the milk fat surface, which could result in changes in the properties of the interfacial surface. From these observations, it was concluded that SFAE concentration and homogenization pressure affect the rheological properties of dairy cream-based emulsions.
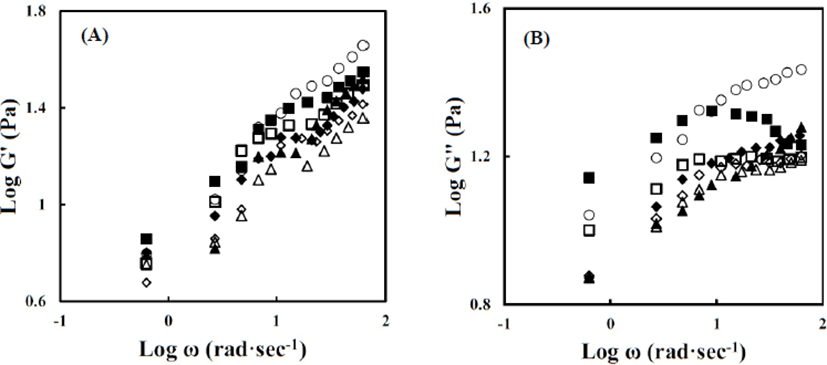
The D[4,3] value calculated from the volume distribution is more suitable than the D[3,2] value for representing the average droplet size with a higher volume and flocculation of the fat droplets (Ariyaprakai et al., 2013). Therefore, the D[4,3] value was used to examine the physical stability of the dairy cream-based emulsions during storage at different temperatures (5°C and 40°C). The D[4,3] values of the top and bottom emulsions with different homogenization pressures (10 MPa and 20 MPa) and SFAE concentrations (0.08%, 0.16%, and 0.24% w/w) are shown in Table 3. In the control, phase separation was observed after storage at 40°C for 7 d. In the case of the control sample stored at 5°C, the droplet size of the top emulsion (10.5 µm) was larger than that of the bottom emulsion (3.16 µm) on day 7, and phase separation was observed on day 15. It has been previously shown that thermodynamically unstable emulsions are easily flocculated by droplet-droplet interactions during storage (Cheng et al., 2016). Therefore, the phase separation of the control could be attributed to the flocculation of milk fat droplets during storage, owing to the unstable emulsions that were non-homogenized or without additives.
The dairy cream-based emulsions homogenized with SFAE did not show any significant change when stored at 5°C for 30 d. However, in the case of storage at 40°C, the droplet size of the top emulsion became larger than that of the bottom emulsion over the storage period (Table 3). This result implied that the flocculation of milk fat droplets had occurred in the emulsions. According to Stokes’s law, the creaming rate increases with an increase in the diameter of the droplets (Long et al., 2012). Flocculated fat could float upward during storage owing to its low density, resulting in a difference in the droplet size between the top and bottom emulsions (McCrae et al., 1999). The droplet size distribution data for the top of the dairy cream-based emulsions stored at 40°C for 30 d are shown in Fig. 4. All emulsions had a secondary peak in the larger size region during storage at 40°C for 30 d, indicating the formation of large droplets. The emulsion homogenized with 0.08% (w/w) SFAE at 20 MPa had a peak with a large tail in the larger size region after storage for 7 d. This peak was divided into two on day 15, and phase separation was observed on day 30. This could be explained by considering the formation of unstable emulsions with a low concentration of SFAE, which were unable to completely cover the newly formed droplet surface during homogenization at high pressures (Floury et al., 2000; Heffernan et al., 2009). Therefore, the milk fat droplets of emulsions stored at 40°C flocculated more rapidly than those of emulsions stored at 5°C, resulting in phase separation.
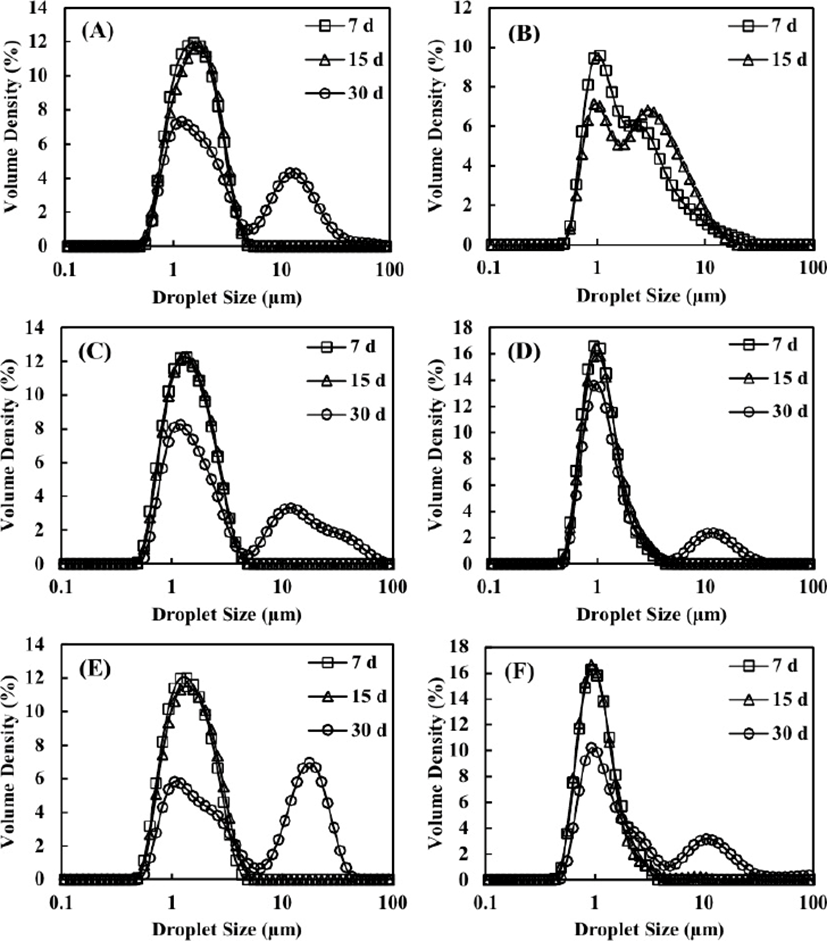
When stored at 40°C for 30 d, the droplet size of the top emulsion increased with the increasing SFAE concentration at the same homogenization pressure, indicating that with a higher SFAE concentration, the emulsion would be more unstable at high temperatures. These findings could be attributed to the protein-surfactant interactions, which affected the stability of the emulsions because of competitive adsorption and displacement (Rouimi et al., 2005). In the presence of an emulsifier, the interfacial strength was reduced as a result of competitive adsorption with proteins, and consequently, the proteins initially adsorbed at the fat droplet interface were released into the aqueous phase (Granger et al., 2005). This produced a thinner and more fragile membrane on the fat droplets, and the emulsion became more susceptible to partial flocculation. Similarly, in the case of emulsions stabilized by milk protein and SFAE, it was found that SFAE weakened the interfacial layer of fat droplets in the emulsions by displacing the proteins (Cheng et al., 2016; Tual et al., 2006). Therefore, the increase in droplet size with an increase in SFAE concentration was attributed to the SFAE adsorbed on the fat droplet, which contributed to the instability of the emulsions at a high temperature (40°C) because of fragile membranes.
Conclusions
In the present study, we found that the physical properties of dairy cream-based emulsions were significantly affected by the addition of SFAE and the homogenization pressure. The droplet sizes in the emulsion decreased with the increasing SFAE concentration by further disrupting the milk fat droplets during homogenization. The rheological properties of the dairy cream emulsions were also dependent on the SFAE concentration and homogenization pressure. This result was attributed to the differences in the interfacial surface, which was covered with SFAE during homogenization. Although the cream emulsions homogenized with SFAE were very unstable during storage at 40°C, all emulsions stored at 5°C were stable for 30 d. From these observations, it was suggested that the addition of SFAE contributed to the formation of stable dairy cream emulsions during homogenization, and that the emulsions with SFAE were very stable during storage at refrigeration temperature (5°C). Therefore, the findings of this study are useful for producing food and beverages that contain dairy cream.