Introduction
Dairy goats were introduced to Korea in the 1960s, and since then, goat milk products have been sold in the form of fresh goat milk and yoghurt (Park, 2006). Goat milk is highly nutritious and has a protein composition similar to that of breast milk (Kaminarides and Anifantakis, 1993; Urbiene et al., 1997). The casein fraction of goat milk is mainly composed of β-casein, followed by αs-casein and κ-casein, in the descending order. In cow milk, αs1-casein is the main constituent of the protein fraction (Lim et al., 2006; Sanz Ceballos et al., 2009). αs1-Casein is known to interfere with digestion in humans and cause formation of solid curds in the stomach, and its concentration is significantly lower in goat milk than in cow milk (Bernacka, 2011; Hinz et al., 2012; Yangilar, 2013).
Goat milk contains smaller fat globules and larger amounts of short- and medium-chain fatty acids than cow milk. These increase the digestibility and bioavailability of goat milk (Park, 1994; Xu et al., 2015). Goat milk also contains abundant vitamins (A, E, B1, B2, and C) and minerals (potassium, calcium, magnesium, phosphorus, sodium, iron, copper, and zinc) with a superior absorption rate (Kim et al., 2013; Kim et al., 2015; Rutherfurd et al., 2006). Furthermore, goat milk includes various bioactive peptides that possess antioxidant properties (Ahmed et al., 2015) and angiotensin-converting-enzyme (ACE)-inhibitory properties (Espejo-Carpio et al., 2013).
Increasing interest in health and nutritional benefits of goat milk is notable in the literature, particularly in the last decade. A large proportion of this research highlights better digestibility and weaker allergenicity of goat milk when compared with cow milk (Espejo-Carpio et al., 2016). High nutritional value and digestibility of goat milk make it an attractive food source for people with malabsorption syndrome and those in vulnerable age groups, for example infants and older people (Alferez et al., 2001). In terms of allergenicity of goat milk, contradicting results can be found in the literature. The majority of relevant studies shows that goat milk is less allergenic than cow milk; however, β-lactoglobulin, which is the major culprit of allergic reactions, is also present in goat milk. This substance is absent in breast milk and is one of the major proteins in cow milk (Haenlein, 2004).
Therefore, the aim of this study was to design a goat milk protein hydrolysate (GMPH), using food-grade commercial proteases. We evaluated four enzymes for selective hydrolysis of the two major proteins that pose digestibility and allergenicity issues − αs-casein and β-lactoglobulin − and attempted to identify optimal conditions to maximize the degree of their hydrolysis. To determine the hypoallergenic properties of the GMPH, the immune responses were measured by assessing the release of histamine and tumor necrosis factor α (TNF-α from human mast cells (the HMC-1 cell line)) in vitro.
Materials and Methods
Goat milk was obtained from Edam Co. Ltd. (Korea) and skimmed at 4,000 rpm for 30 min (Labogene 1736R). Four food-grade commercial proteases (Alcalase, Flavourzyme, Neutrase, Protamex) were purchased from Novozymes® (Denmark) to hydrolyze αs-casein and β-lactoglobulin, among other goat milk proteins. Incubation temperature (30-70℃), incubation time (10-60 min), and enzyme concentration (0.2-1.0%, w/w) were varied to determine the optimal conditions for hydrolysis. Upon completion of the enzymatic hydrolysis reactions, the samples were heated at 90℃ for 15 min to inactivate the enzymatic activity. They were freeze-dried and analyzed by SDS-PAGE and reverse-phase high-performance liquid chromatography (RP-HPLC). The degree of hydrolysis by each enzyme in each condition was then determined by quantification of nonprotein nitrogen (NPN).
This procedure was carried out on a 12.5% acrylamide gel, as described by Laemmli (1970). The sample was mixed with 2× sample buffer (0.125 M Tris-HCl pH 6.8, 4% SDS, 20% glycerol, and 2% β-mercaptoethanol) and then heated at 95℃ for 10 min. Electrophoresis was performed at 20 mA for 1 h, using a Mini-Protean® Tetra System and PowerPacTM HV (Bio-Rad, Hercules, USA). The gel was stained for 1 h with a Coomassie blue solution (0.025% Coomassie blue g-250, 40% methanol, and 7% glacial acetic acid). Analysis of the bands on the gel was performed using a Molecular Imager® GelDocTM XR plus Imaging system and the Image LabTM software version 5.1 (Bio-Rad).
This analysis was performed by the method of Bobe et al. (1998). Acetonitrile was of HPLC grade, and all other reagents were of analytical grade. Bovine αs-casein, β-casein, κ-casein, β-lactoglobulin, and α-lactalbumin were purchased from Sigma-Aldrich (USA). Sample buffer [0.1 M bis-Tris-HCl pH 6.8, 6 M guanidine hydrochloride-HCl, 5.37 mM sodium citrate, and 19.5 mM dithiothreitol (DTT)] was mixed with standard proteins or GMPH. The mixtures were incubated for 1 h, and then centrifuged for 5 min at 12,000 rpm (Vision VS-15000CNF, Korea). The supernatant was passed through a polyvinylidene difluoride (PVDF) syringe filter (0.22-μm pore size, Woongki Science, Korea), and diluted for injection (20 μL). The HPLC system (Waters) consisted of two HPLC pumps, a sample injector, and an absorbance detector. A silica-based C18 RP-HPLC column (250 mm length × 4.6 mm i.d., 5.0 μm; Waters) was used for protein separation with solvents A and B at a flow rate of 1 mL/min. Solvent A consisted of 0.1% acetonitrile in 0.1% trifluoroacetic acid and solvent B consisted of 90% acetonitrile in 0.1% trifluoroacetic acid in HPLC-grade water. The absorbance was measured at 220 nm, using a Photodiode Array Detector 2996 (Waters). The solvent gradient started at 25% of solvent B and was maintained for 5 min after sample injection, followed by an increase in the proportion of solvent B at 0.5%/min (for 9 min), 0.25%/min (4 min), 0%/min (4 min), and 0.5%/min (14 min), and then the proportion of solvent B was increased to 100%.
NPN contents were measured by the Folin-Lowry method (Lowry et al., 1951). In brief, 2 mL of the hydrolyzed sample and the same volume of 24% trichloroacetic acid solution were mixed, incubated for 30 min, and centrifuged at 3,000 rpm for 20 min (Labogene 1736R). Next, 1 mL of the supernatant was transferred to a fresh test tube, 5 mL of the assay reagent (2% sodium carbonate in a 0.1 N sodium hydroxide solution and 0.5% copper sulfate pentahydrate in a 1% sodium citrate solution mixed in a 50:1 ratio) was added, the mixture was incubated for 15 min at room temperature, and then mixed with 0.5 mL of the phenol reagent. After 30 min of incubation, the absorbance of the mixture was measured at 750 nm. The standard solution was prepared from bovine serum albumin.
A human mast cell line (HMC-1 cells) was obtained from the Pathophysiology Laboratory of Sahmyook University. HMC-1 cells were grown in Dulbecco’s modified Eagle’s medium (Cellgro) with 10% of fetal bovine serum (Hyclone) and 1% penicillin (Gibco) at 37℃ in a humidified atmosphere containing 5% CO2. HMC-1 cells (105/well) were cultured overnight in a 24-well plate, and then the followings (100 μL) were added: 10 μg/mL compound 48/80 (Sigma) as a positive control, and 100 μg/mL of ovalbumin (Sigma), soy protein (Esfood), goat milk whey protein, or GMPH, respectively. The cells were incubated for additional 24 h. Histamine and TNF-α released into the culture supernatant were measured by means of the histamine ELISA kit (IBL International) and human TNF-α ELISA Ready-SET-Go® Kit (eBioscience), respectively, according to the manufacturer’s protocol. The absorbance was measured at 450 nm, using a microplate reader (Molecular Devices).
Results
Hydrolysis of goat milk proteins by 0.4% alcalase at 60℃ for 30 min was found to be the most effective, resulting in a significant reduction in the amounts of αs-casein and β-lactoglobulin in the GMPH according to SDS-PAGE analysis (Fig. 1A). Cow milk protein (CMP) and goat milk protein (GMP) were also analyzed in parallel for comparison. In Fig. 1A, it is evident that the amounts of all five major proteins studied (αs-casein, β-casein, κ-casein, β-lactoglobulin, and α-lactalbumin) markedly decreased in GMPH (compared with CMP and GMP) with increasing incubation temperature, incubation time, and enzyme concentration, as indicated by the fading intensity of the bands on the electrophoretic gel. Nonetheless, the rates of hydrolysis at a higher incubation temperature (> 60℃), incubation periods longer than 30 min, and enzyme concentrations higher than 0.4% yielded no significant differences in the optimal conditions, with respect to NPN content, as shown in Fig. 1B. The NPN content under the optimal conditions was approximately 6 mg/mL. Measurements of the degree of hydrolysis of GMPH by the O-phthaldialdehyde method and quantification of free amino nitrogen indicated tendencies similar to the trend in the results of the NPN method (data not shown).
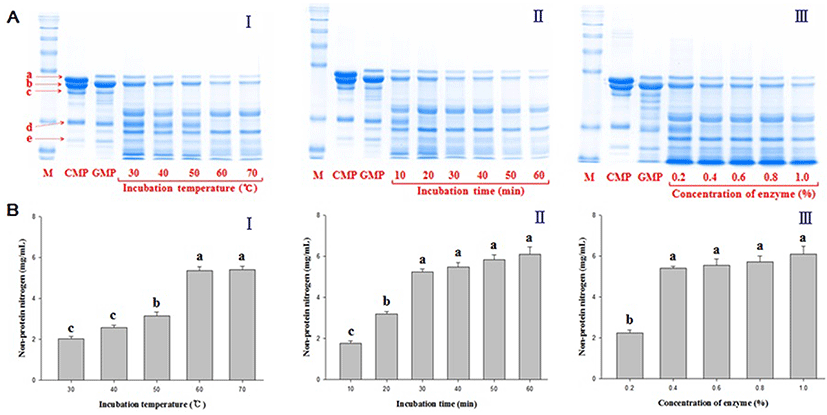
Hydrolysis of the five major proteins in GMPH – κ-casein, αs-casein, β-casein, α-lactalbumin, and β-lactoglobulin – by 0.4% alcalase at 60℃ for 30 min was confirmed by SDS-PAGE and RP-HPLC (Fig. 2). In comparison with the bovine milk protein standard, it was evident that αs-casein and β-lactoglobulin were effectively hydrolyzed in GMPH. According to the SDS-PAGE results and the chromatograms in Fig. 2, a ~40% reduction in αs-casein content was achieved with alcalase under optimal conditions, as well as near-complete elimination of β-lactoglobulin (GMPH in Fig. 2).
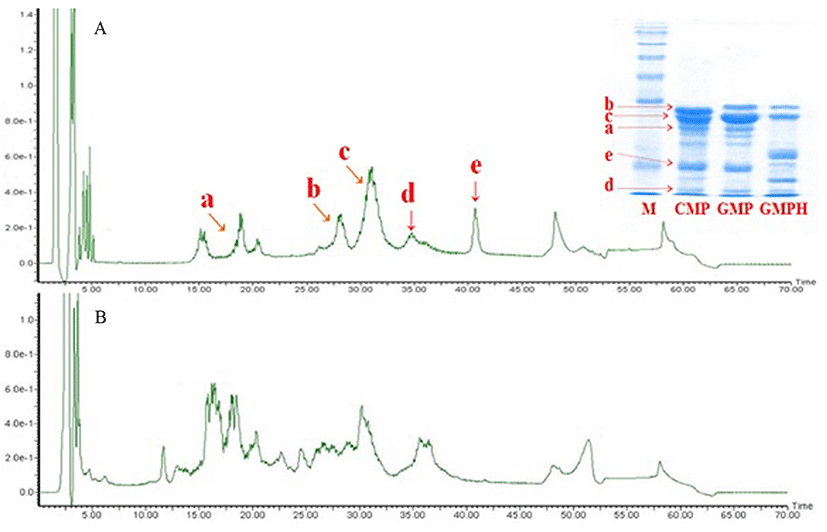
HMC-1 cells were incubated with the GMPH or other protein samples to test whether they elicit an allergic reaction. Treatment with compound 48/80 as a positive control, ovalbumin, soy protein, or goat milk whey protein (GMW) containing β-lactoglobulin significantly (p<0.01) stimulated the release of both histamine and TNF-α when compared to the control, in the descending order. In contrast, treatment of HMC-1 cells with the GMPH did not significantly induce either histamine or TNF-α release when compared to the control, as shown in Figs. 3A and 3B, respectively. Less than 15 ng/mL histamine and less than 5 pg/mL TNF-α were released by HMC-1 cells treated with the GMPH.
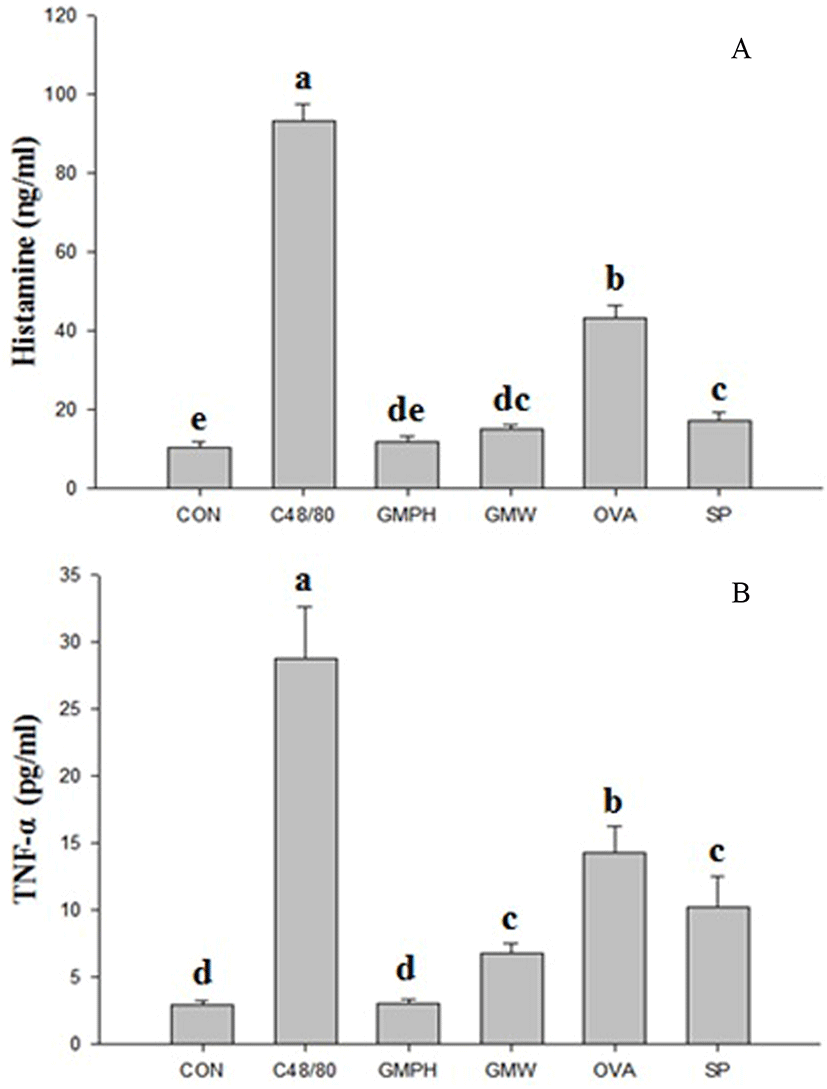
Discussion
Four food-grade commercial enzymes (Alcalase, Neutrase, Protamex, and Flavourzyme) were used to selectively hydrolyze and reduce the concentrations of αs-casein and β-lactoglobulin, which are related to digestive problems and allergic reactions, respectively, in goat milk. It was shown here that alcalase treatment effectively hydrolyzed αs-casein and β-lactoglobulin: better than any other commercial enzymes tested.
It is known that milk αs-casein consists of αs1- and αs2-casein. Although αs2-casein appears harmless, αs1-casein interferes with digestion and protein absorption by forming solid curds in the stomach (Kim et al., 2014). Both cow and goat milk contain αs-casein. However, cow milk protein is mainly composed of αs1-casein, whereas goat milk mostly contains αs2-casein and a small amount of αs1-casein (Yangilar, 2013). For this reason, goat milk rarely interferes with digestion in humans. Nevertheless, optimal hydrolysis conditions for the proteases were explored to reduce the amounts of both αs1-casein and β-lactoglobulin. Since hydrolysis of GMP with alcalase at higher temperature, incubation time, and enzyme concentration yielded excessive amounts of protein degradation products in GMPH, the optimal hydrolytic conditions were assumed to be those when β-lactoglobulin was degraded almost to nil. The resultant effect of hydrolysis with alcalase was clearly observed in the SDS-PAGE and RP-HPLC analyses, particularly when compared with CMP and GMP.
The GMPH was tested for allergic reactions by measuring histamine and TNF-α release from HMC-1 cells. Generally, when the human body is exposed to an allergen, Langerhans cells recognize it, causing activation of Th2 lymphocytes, which drive immunoglobulin E (IgE) production. The secreted IgE binds to high-affinity IgE receptor (FcεRI) on the surface of mast cells (Novak and Bieber, 2005), and the excited mast cells contribute not only to the release of histamine, which is a mediator of the immediate hypersensitivity reaction, but also to TNF-α production in the late phase (Je et al., 2013; Kalesnikoff and Galli, 2008). Here, mast cells (cell line) were treated with compound 48/80 (C48/80) as a positive control, as well as other types of proteins including ovalbumin, soy protein, GMW, and GMPH.
As a mixed polymer of p-methoxy-N-methyl phenylethylamine, the C48/80 compound is widely used to stimulate the release of histamine and TNF-α (Matsui et al., 2015). Ovalbumin and soy protein are also reported to induce an allergic reaction (Cordle, 2004; Zhang et al., 2015). Two indicators of an allergic reaction were significantly increased in the groups treated with C48/80, ovalbumin, GMW, or soy protein, but the GMPH did not elicit allergic reactions when compared to the control (no significant difference with control). The allergic reaction to GMW may have been induced by the presence of β-lactoglobulin. Hence, the GMPH can be considered a hypoallergenic food product. Further in vivo experiments are under investigation. Moreover, De Gobba et al. (2014) and Osman et al. (2016) reported that the GMPH prepared by means of alcalase has antioxidant properties and antibacterial activity, and contains ACE-inhibitory peptides, which can be effective at prevention and treatment of lifestyle diseases such as hypertension (Espejo-Carpio et al., 2013).
Conclusion
Goat milk protein was hydrolyzed with 0.4% alcalase at 60℃ for 30 min, and this procedure effectively reduced the concentrations of αs-casein and β-lactoglobulin, as confirmed by the results of SDS-PAGE and RP-HPLC. The GMPH did not induce the release of histamine and TNF-α from HMC-1 cells, i.e., did not elicit an allergic reaction in a human mast cell line. The results of this study confirm better digestibility and hypoallergenic properties of the GMPH than that of other types of milk protein. Goat milk production dramatically decreases in the winter season, resulting in an imbalance of annual goat milk supply and demand, and causing instability of the entire goat milk industry (Lim et al., 2006). The seasonal imbalance of goat milk supply and demand could be overcome by converting the goat milk collected in the summer season into the GMPH as a powdered form, and by using it to develop functional foods. Food applications may include the development of a formula milk powder and other types of dairy products for infants, the elderly, and convalescent patients, thus replacing cow milk. With the increasing consumer demand for elimination of sources of allergens from foods, further research is warranted for the suitability of the GMPH as a novel ingredient for dairy product development, and for the effects of GMPH on health in comparison with those of other sources of milk protein.