Introduction
In the US, cows and bulls comprise a substantial portion of the total cattle harvested at 17.4% (cows) and 1.7% (bulls) of the approximately 15.86 million head slaughtered in 2020 at federally inspected facilities (USDA, 2020). Beef carcasses determined to be mature are categorized by the USDA into classes including Premium White, Breaker, Boner, Cutter, and Bull (USDA, 2022a). Although related to chronological age, maturity is assessed physiologically through darkening of the lean color and skeletal ossification (Hale et al., 2013). For the purposes of this review, youthful carcasses refer to those coinciding with a chronological age under 30 months and mature carcasses over that age. Most studies addressed, however, have examined beef carcasses with a maturity well over this threshold. Owing to high lean and skeletal maturity, mature carcasses would be ineligible for higher USDA quality grades generally reserved for cattle under the age of 30 months. Relative to USDA Choice cattle, those over 30 months of age would receive a discount of approximate $20.00 USD per 100 pounds, and those classified as hard bone would be discounted approximately $35.83 USD per 100 pounds (USDA, 2022b). Unacceptable palatability traits, especially tenderness, often results in cull cow beef being further processed into ground beef, thus lowering the economic value compared to whole muscle cuts (USDA, 2022c). Regardless, it is well established that the sale of cull cows contributes a considerable portion to the income of producers (UNL Beef, 2016).
Ensuring the consistent quality of beef products is a major priority of the industry, as uncontrolled variation in quality, especially tenderness, can contribute to consumer dissatisfaction (Picard and Gagaoua, 2017). Considerable attention has been given to improving the quality of cull cow beef prior to harvest through improved herd management, better handling practices, among others (NBQA, 2017). For example, feeding a high-concentrate diet has been shown to be beneficial to carcass and meat quality traits of cull cow beef including yield, marbling, and sensory tenderness (Gredell et al., 2018; Matulis et al., 1987; Miller et al., 1987; Schnell et al., 1997; Therkildsen et al., 2011). Particularly, Gredell et al. (2018) found that increased marbling could mitigate some of the negative impacts of increasing maturity on beef palatability. Yet, pre-harvest factors may only improve quality to a certain extent and may not always be an economical choice for producers. Compared to youthful carcasses of similar marbling scores, mature beef longissimus lumborum muscles would be rated by trained panelists as poorer for both initial and sustained tenderness scores (Crownover et al., 2016). This deficiency in tenderness of mature beef is often highly noticeable in more inherently tough cuts from the chuck and round primals. The study by Roberts et al. (2017) reported poorer initial and overall tenderness scores, greater perceptible connective tissue, and higher shear force values in the semimembranosus, biceps femoris, semitendinosus, triceps brachii, and others from carcasses classified as mature by the Canadian grading system relative to those under 30 months of age. In general, the poor tenderness of mature beef relative to youthful carcasses is dependent on the individual cut, where the differences may be less apparent in certain inherently tender muscles such as the psoas major, infraspinatus, and longissimus lumborum (Alvarenga et al., 2021; Roberts et al., 2017; Stelzleni et al., 2007). Considering these unique challenges in regard to palatability of mature beef, especially tenderness, this review aims to discuss the existing and innovative post-harvest strategies to improve tenderization.
Factors Influencing Tenderness of Mature Beef
Discrepancies in tenderness can be explained by the considerable variations that exist for the factors determining tenderness including postmortem proteolysis, background toughness, sarcomere length, and intramuscular lipid content (Warner et al., 2022). The extent to which each of these factors dictates tenderness is well known to differ between cuts, thus understanding which may be most relevant to tenderness is critical to improving quality (Anderson et al., 2012; Chun et al., 2020; Jeremiah et al., 2003; Rhee et al., 2004; Stolowski et al., 2006). An overview of how these factors influence the tenderness of mature beef is provided in Fig. 1. The degradation of myofibrillar proteins during the postmortem period is primarily attributed to the activity of calpain proteases (Kemp et al., 2010; Koohmaraie, 1992; Koohmaraie, 1994; Warner et al., 2022). Calpain-1, also referred to as μ-calpain, requires approximately 30 μM concentration of calcium ions for half-maximal activation, while a 350 μM concentration is required by calpain-2, also called m-calpain (Campbell and Davies, 2012). Both forms of calpain are considered to play a role in the postmortem tenderization of beef, with calpain-1 being attributed to early tenderization and calpain-2 with longer aging durations (Colle and Doumit, 2017). In mature beef, the study by Xiong et al. (2007) reported that the degradation of troponin-T, a marker of postmortem proteolysis, would be less apparent during aging in the longissimus thoracis et lumborum compared to younger animals. This finding was credited to the muscles from mature carcasses having been subjected to greater oxidative stress, resulting in the inactivation of calpain-2 (Xiong et al., 2007). Oxidative stress has also been demonstrated to inactivate calpain-1 through the formation of a disulfide bond in the active site (Lametsch et al., 2008). Similarly, Cruzen et al. (2014) reported that mature beef would be categorized by greater calpastatin activity and less autolysis of calpain-1. Consequently, the degradation of myofibrillar proteins such as troponin-T would be delayed, especially apparent in the triceps brachii (Cruzen et al., 2014). However, discrepancies in the role of proteolysis in explaining the differences in tenderness between animal maturities have also been reported. For instance, the study by Lucero-Borja et al. (2014) found that the amount of intact troponin-T in the longissimus thoracis of cull cows would be similar to that of steers and heifers. While differences in the postmortem proteolysis of myofibrillar proteins would likely be a contributing factor to the toughness of cull cow beef, it is also well established that postmortem proteolysis does not explain all variation in tenderness, especially when considering cuts with considerable background toughness (Anderson et al., 2012; Rhee et al., 2004; Veiseth-Kent et al., 2018).
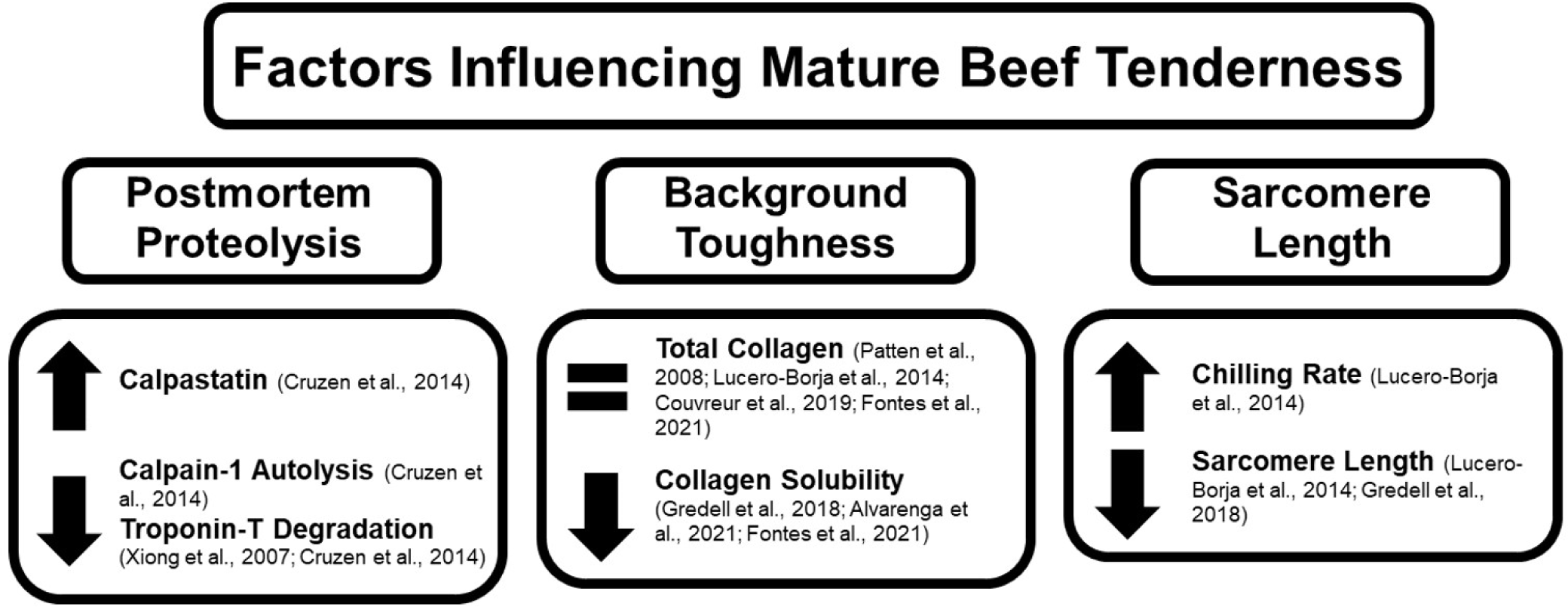
The differences in tenderness between animal maturities would likely be primarily driven by differences in the presence and extent of cross-linking of intramuscular connective tissues. These connective tissues are comprised of collagen in a matrix of proteoglycan, as well as other stromal proteins including elastin and reticulin (Lepetit, 2008). Fibrous collagen is known to form cross-linked structures and be poorly soluble, and the extent of cross-linking is known to increase with animal maturity (Purslow, 2018). The study by Patten et al. (2008) observed that total collagen content would be similar for most muscles in mature cows and bulls relative to USDA Select steers, with the exception of some differences for the gluteus medius and infraspinatus muscles. In agreement, Lucero-Borja et al. (2014) reported that total and insoluble collagen contents of longissimus thoracis muscles from mature cows would be similar to youthful carcasses. However, despite this, the cows would have greater instrumental toughness, poorer sensory tenderness scores, and a greater amount of perceptible connective tissue (Lucero-Borja et al., 2014). While no differences in total collagen content of the longissimus thoracis between cull cows and heifers were also found by Dos Santos Fontes et al. (2021), it was reported that the solubility of collagen in the cull cows would be approximately half that of heifers (5.54% versus 10.53%). Interestingly, Gredell et al. (2018) found that the longissimus muscle from mature unfed cattle would actually have a lower content of total collagen compared to young fed cattle. However, the heat solubility of the collagen from mature unfed cattle would be drastically lower (6.1%–8.1% versus 27.3%–32.5%; Gredell et al., 2018). In general, most literature supports that total collagen content would be mostly similar between animal maturities (Couvreur et al., 2019; Dos Santos Fontes et al., 2021; Lucero-Borja et al., 2014; Patten et al., 2008), although collagen solubility would decrease with increasing age (Alvarenga et al., 2021; Dos Santos Fontes et al., 2021; Gredell et al., 2018). A greater amount of perceptible connective tissue in cooked beef from mature carcasses has also been consistently reported (Jurie et al., 2007; Lucero-Borja et al., 2014; Roberts et al., 2017). Between beef and dairy type cull cows, total and insoluble collagen contents would be similar (Jurie et al., 2007).
Sarcomere length, considered as the region between adjacent Z lines on the myofibril, is another factor that would be relevant to tenderness differences between animal maturities (Ertbjerg and Puolanne, 2017; Warner et al., 2022). There is a well-established relationship between sarcomere length with both instrumental and sensory tenderness of fresh beef, the strength of which differs between individual cuts (Rhee et al., 2004). Non-fed cull cows may experience more rapid chilling owing to decreased muscling and less subcutaneous fat covering (Lucero-Borja et al., 2014; Patten et al., 2008; Stelzleni et al., 2007), which may have considerable implications on the degree of sarcomere shortening. The study by Lucero-Borja et al. (2014) found that the sarcomere length of the longissimus thoracis of cows (1.98 μm) would be similar to heifers (2.02 μm) and steers (2.00 μm). However, it was also reported that these carcasses would experience considerably more rapid chilling, as shown by a temperature at 3 hours postmortem approximately 4.7°C–5.1°C lower than heifers and steers. The temperature at 3 hours postmortem had a moderate positive correlation (r=0.54) with the area of the loin muscle. Principal component analysis indicated that more rapid carcass chilling would be associated with shorter sarcomeres among cull cows, and significant negative correlations were found between sarcomere length and objective and subjective tenderness (Lucero-Borja et al., 2014). Supporting this, Gredell et al. (2018) found that the sarcomere length of the longissimus from mature fed cattle would be similar to that of young fed cattle, while the sarcomeres of mature unfed cattle would be shorter. This contributed to mature unfed cattle having considerably higher Warner-Bratzler shear force (WBSF) values compared to the other groups (Gredell et al., 2018). As will be later discussed, preventing shortening and increasing sarcomere length is a primary rationale for applying interventions such as electrical stimulation (Boleman et al., 1996; Hwang and Thompson, 2001) or skeletal separation during chilling (Mandell et al., 2006; Streiter et al., 2012).
Another factor which may impact the tenderness of cull cow beef would be the content of intramuscular lipids. As previously discussed, several studies have supported that feeding a high-concentrate diet prior to slaughter could contribute to superior marbling and palatability traits (Matulis et al., 1987; Miller et al., 1987; Schnell et al., 1997; Therkildsen et al., 2011). While the focus of this review is on post-harvest factors, some consideration to intramuscular lipid content as a factor which may influence quality should be made. The study by Patten et al. (2008) reported that the proximate lipid content of fed beef and dairy cows would be mostly similar to USDA Select carcasses across several cuts. However, especially among non-fed beef cows, the intramuscular lipid content would be considerably lower in the gluteus medius, infraspinatus, and tensor fasciae latae muscles (Patten et al., 2008). Among non-fed dairy cows, intramuscular lipid content would be mostly similar to USDA Select carcasses (Patten et al., 2008), although marbling scores may be slightly elevated (Stelzleni et al., 2007). While feeding a high-concentrate diet has been shown to coincide with greater accretion of intramuscular lipids (Patten et al., 2008; Stelzleni et al., 2007; Therkildsen et al., 2011), improvements in tenderness would be mostly attributable to an increase the heat solubility of collagen (Aberle et al., 1981; Miller et al., 1987; Stelzleni et al., 2007), rather than direct improvements through marbling. In a study of cull cows and young bulls, Dransfield et al. (2003) found that the highest eating quality class would be categorized by both greater lipid content and lower insoluble collagen.
Post-Harvest Strategies
The implications of chilling on the quality of beef has been well documented (Savell et al., 2005). As previously discussed, mature beef animals, especially non-fed dairy cattle, often exhibit rapid carcass chilling from a low extent of muscling and subcutaneous fat covering (Lucero-Borja et al., 2014; Patten et al., 2008; Stelzleni et al., 2007). Consequently, shortening of the sarcomeres has been reported in several studies (Gredell et al., 2018; Lucero-Borja et al., 2014). Electrical stimulation has been applied as a method to accelerate glycolysis after slaughter in an effort to prevent excessive shortening of the sarcomeres through the rapid ATP depletion prior to chilling during the conversion of muscle to meat. In general, electrical stimulation would result in an immediate increase in the rate of postmortem glycolysis, contributing to a rapid decline in pH (Hwang and Thompson, 2001; Hwang et al., 2003; Simmons et al., 2008). Some studies have reported a positive impact of electrical stimulation on the tenderness of mature beef (Boleman et al., 1996; McKeith et al., 1980; Sonaiya et al., 1982), while others have not (Hawrysh and Wolfe, 1983). This discrepancy would likely owe to the study by Hawrysh and Wolfe (1983) applying extra low voltage electrical stimulation to the carcasses rather than higher voltages. The study by Sonaiya et al. (1982) reported that electrical stimulation would decrease WBSF values of the longissimus, semimembranosus, and triceps brachii muscles from cows compared to unstimulated carcasses. However, fragmentation of the myofibrils and degradation of troponin-T would not be impacted by stimulation (Sonaiya et al., 1982). Boleman et al. (1996) observed that electrical stimulation would decrease Instron shear force values of the longissimus from non-fed cows from 8.5 to 5.7 kg. For cows on feed for 84 days, no impact of electrical stimulation on instrumental tenderness would be found (Boleman et al., 1996). While this may be partly due to time on feed increasing the amount of heat soluble collagen, this effect would likely be attributable to increased size of the carcass and greater subcutaneous fat thickness lessening cold shortening (Boleman et al., 1996).
An alternative approach to accelerating postmortem glycolysis during rapid carcass chilling through electrical stimulation would be the use of delay chilling. In delay chilling, the carcass would be held at a higher temperature for a certain period prior to chilling. The study by Fields et al. (1976) found that delaying carcass chilling for a period of 12 to 20 hours at a temperature of 14°C to 19°C would be beneficial to tenderness attributes. In general, delay chilling resulted in lengthened sarcomeres and greater instrumental tenderness, although the differences would be negligible as postmortem aging progressed (Fields et al., 1976). Conversely, Phoya and Will (1986) found that delay chilling for a period of 4 hours prior to hot boning would have increase the WBSF values and negatively impact the sensory tenderness of the longissimus, psoas major, and quadriceps femoris muscles. This negative impact would likely owe to the effects of hot boning more so than delay chilling. In mature carcasses, hot boning has been shown to negatively impact tenderness across various muscles such as the longissimus and semimembranosus (Berry and Cross, 1982; Eilers et al., 1994; Pivotto et al., 2014; Will and Beaty, 1986). For instance, Berry and Cross (1982) found that hot boning would result in shortened sarcomeres and decreased collagen solubility, thereby increasing instrumental toughness. However, hot boning when used in conjunction with enhancement with functional ingredients (e.g., sodium tripolyphosphate, lactic acid) could be advantageous to the tenderness development of mature beef (Eilers et al., 1994; Pivotto et al., 2014) and will be discussed in greater detail in Section 3.4.
One method that may be utilized to prevent cold shortening during the chilling process would be skeletal separation. During the skeletal separation process, muscles are physically stretched through being subjected to the weight of the hanging carcass enabled through severing of the thoracic and/or lumbar vertebrae. The study by Mandell et al. (2006) found that skeletal separation would increase the sarcomere length of the longissimus thoracis and longissimus lumborum by 0.15 and 0.14 μm, respectively, relative to conventionally chilled controls. This resulted in the longissimus thoracis and anterior portion of the longissimus lumborum having significantly lower WBSF values, although the effect was negligible in the posterior section of the loin (Mandell et al., 2006). Similar intermuscular differences were reported by Streiter et al. (2012), where skeletal separation would increase sarcomere length of the longissimus thoracis and longissimus lumborum muscles from cull cows but not the semimembranosus or semitendinosus. The impacts on WBSF values coincided with these observations, likely due to negligible physical stretching induced in the round coupled with a greater amount of intramuscular connective tissues (Streiter et al., 2012).
A novel method with great promise to improve the tenderness development of cull cow beef would be the use of vascular chilling. In this process, a chilled isotonic solution containing substrates such as glucose and maltose is infused into the vasculature following exsanguination. The recent study by Kethavath et al. (2022) applied the vascular chilling process to cull dairy cows of poor body condition. Relative to conventionally chilled controls, vascular chilling resulted in a more rapid pH decline and considerably longer sarcomeres of the longissimus lumborum (1.80 versus 1.42 μm). Consequently, a remarkable decrease (55%–58%) in WBSF values of the longissimus lumborum with vascular chilling was observed. These findings were attributed to the conventionally chilled carcasses exhibiting cold shortening due to poor body condition, which may have been mitigated if electrical stimulation had been used. The more rapid pH decline facilitated by the infusion of metabolizable substrates through vascular chilling was considered to prevent shortening, as the optimal conditions for cold shortening would be at low temperatures when muscle pH is still high (Kethavath et al., 2022). Similar findings were reported by Santos et al. (2016) where slow pH decline in the longissimus thoracis et lumborum from cull cows would be associated with greater toughness. Similarly, Lucero-Borja et al. (2014) also suggested that cull cows exhibiting a more rapid temperature decline but not a more rapid pH decline would create the ideal conditions for shortening. Thus, it is reasonable that accelerating the rate of postmortem glycolysis in mature beef carcasses would be beneficial for tenderness development. However, further study would be necessary to determine how effective vascular chilling would be in relation to other methods such as electrical stimulation in cull cows.
As previously discussed, beef tenderness can be improved during postmortem storage through the activity of endogenous proteolytic enzymes degrading myofibrillar structure (Huff Lonergan et al., 2010; Kim et al., 2018; Warner et al., 2022). Generally, aging is conducted in anaerobic packaging to serve as a protective barrier and minimize product loss in a process known as wet aging. Most tenderization during the aging process would be attributable to degradation of structures at the Z disk connecting adjacent sarcomeres by calpains (Huff Lonergan et al., 2010). Especially if physically disruptive processes are applied, other enzymes with proteolytic activity on myofibrillar and stromal proteins like cathepsins may contribute to tenderization (Wang et al., 2022). Especially relevant to the tenderness development of mature beef would be the degradation of intramuscular connective tissues. Although intramuscular connective tissues exhibit little degradation early postmortem, disintegration with extended aging may be relevant to final product quality (Nishimura et al., 1998).
The study by Xiong et al. (2007) found that while WBSF of the longissimus from older animals would be greater than young carcasses, this cut would still exhibit considerable tenderization from 1 to 10 days postmortem. However, it is also known that the impacts of postmortem aging on tenderness would be muscle-specific (Kim et al., 2018; Ma and Kim, 2020; Nair et al., 2019; Stolowski et al., 2006). In cull cows, consistent improvements in WBSF values have been found in the longissimus thoracis and longissimus lumborum muscles from 7 to 21 days postmortem (Streiter et al., 2012). However, while the semitendinosus would decrease in WBSF from 7 to 14 days, additional tenderization would not be achieved past this, and negligible tenderization would be observed in the semimembranosus (Streiter et al., 2012). Similarly, Mandell et al. (2006) reported significant decreases in instrumental toughness of the longissimus thoracis and longissimus lumborum over the course of 28 days of aging. However, the positive effects on round muscles including the semitendinosus, semimembranosus, and biceps femoris would be limited (Mandell et al., 2006). In contrast, Bhat et al. (2018a) found that the semimembranosus and biceps femoris muscles from cull cows would improve in instrumental tenderness over the course of 14 days of postmortem aging, attributable to an increase in myofibrillar fragmentation and degradation of myofibrillar proteins. These discrepancies could owe from those carcasses being electrically stimulated (Bhat et al., 2018a), whereas electrical stimulation was not used by Streiter et al. (2012) and Mandell et al. (2006). Intermuscular differences were also reported by Alvarenga et al. (2021) who found that the longissimus lumborum from mature carcasses would decrease in shear force approximately 30.5% from 2 to 28 days postmortem; however, the semitendinosus would only decrease by approximately 3.4%. Consequently, only 7.3% of those longissimus lumborum steaks would be considered tough by 28 days postmortem (Alvarenga et al., 2021), indicating that sufficient aging could overcome the toughness of certain cuts from the loin and rib primal. However, while the study by Franco et al. (2009) found that aging would decrease instrumental toughness of the longissimus thoracis from cull dairy cows, additional tenderization past 14 days would not be observed. Consequently, those steaks would still be categorized as “tough” (WBSF>4.6 kg; Belew et al., 2003) even by 42 days of postmortem aging (Franco et al., 2009). Lucero-Borja et al. (2014) found that sensory tenderness scores of the longissimus thoracis from cows would not improve from 3 to 14 days of aging, and those steaks would be inferior in initial and overall tenderness scores compared to heifers and steers. Sufficient postmortem aging may be an effective strategy to produce cull cow beef steaks with acceptable tenderness; however, it may be unable to overcome the inherent toughness of certain muscles, and some cuts even from the loin and rib primals may never reach an acceptable threshold. Another important consideration with extended aging durations would be a coinciding decrease in oxidative stability (Ma et al., 2017), which has been demonstrated in cull cow beef longissimus thoracis et lumborum muscles during retail display (Vitale et al., 2014).
Another recently considered method to improve the quality attributes of cull cow beef would be the application of dry aging. In contrast to wet aging, dry aging does not use a protective barrier but rather controls environmental factors such as temperature, humidity, and air speed. The use of dry aging has been shown to improve the palatability traits of beef through the generation and concentration of flavor precursors including amino acids and nucleotides (Setyabrata et al., 2021). Considering many cull cows are finished on forage, it has been shown that dry aging would be beneficial to the sensory flavor and tenderness of grass-fed beef longissimus lumborum steaks (Berger et al., 2018). The findings for flavor could be partly attributed to a decrease in certain plant-derived compounds such as terpenoids, which may add a vegetal or grassy flavor to the meat (Setyabrata et al., 2021). A recent study by Setyabrata et al. (2022) examined the use of dry aging on the longissimus lumborum muscles from cull dairy cows. Over the course of 28 days of aging, it was found that dry aging would have no impact on the instrumental or subjective tenderness scores relative to conventional wet aging. However, dry aging would be beneficial at reducing negative flavor attributes such as animal fat and sour notes (Setyabrata et al., 2022). In partial agreement, Obuz et al. (2014) found that wet aged longissimus lumborum steaks from cull cows would have greater overall tenderness scores compared to dry aging; however, dry aging would decrease the intensity of off-flavors. In general, tenderness development between aging methods would be mostly similar, while the application of dry aging could be considered by producers as a potential means to reduce negative flavor attributes associated with beef from mature animals. An important consideration regarding this method would be the loss of saleable product through the formation of a dried surface that must be trimmed prior to consumption (Berger et al., 2018; Obuz et al., 2014; Setyabrata et al., 2022). Some consumers may be willing to pay a premium price for dry aged beef (Berger et al., 2018; Setyabrata et al., 2022), although the percentage willing to pay this premium may be slightly lower for beef from cull cows compared to youthful carcasses (Setyabrata et al., 2022). Regardless, dry aging could be beneficial to reduce some of the off-flavors frequently observed in cull cow beef with poor marbling (Gredell et al., 2018; Stelzleni and Johnson, 2010).
Mechanical tenderization methods are routinely applied in the meat industry to improve the tenderness of inherently tough beef. These may include the use of blades or needles to physically sever the myofibers, as well as the use of tumbling or massaging to disrupt and solubilize muscle proteins (Bhat et al., 2018b). In a study of blade tenderization on the longissimus, biceps femoris, and semimembranosus muscles from mature beef carcasses, Tatum et al. (1978) found that the process would be beneficial to decrease instrumental toughness. However, mechanical tenderization would not be sufficient to improve these cuts to the point of being comparable to non-mechanically tenderized steaks from youthful carcasses. Sensory panelists rated the blade tenderized longissimus from mature carcasses as having similar myofibrillar tenderness; however, a greater amount of perceptible connective tissue resulted in overall tenderness being poorer (Tatum et al., 1978). When blade tenderization is combined along with additional postmortem aging, improvements in myofibrillar tenderness scores of the longissimus lumborum from cull cows has been shown (Obuz et al., 2014). However, such improvements would be found only if wet aging was applied rather than dry aging (Obuz et al., 2014), which could be related to both dry aging and mechanical tenderization resulting in moisture loss and poorer juiciness in some studies (Jeremiah et al., 1999; Setyabrata et al., 2022).
While tumbling is routinely applied alongside enhancement with a brine or marinade solution (Boles and Shand, 2002; Garmyn et al., 2020; Morrow et al., 2019), several recent studies have explored its potential application in the absence of these functional ingredients in beef (Morrow et al., 2019; N’Gatta et al., 2021; Nondorf and Kim, 2022; Tuell et al., 2021; Tuell et al., 2022). Considering the primary rationale for tumbling is often as a method of brine incorporation, studies using tumbling for this purpose will be discussed in Section 3.4. However, tumbling in the absence of brine may allow producers to apply additional postmortem aging in an attempt to promote the degradation of myofibrillar and stromal proteins (Nondorf and Kim, 2022; Tuell et al., 2021; Tuell et al., 2022). Highlighting this, tumbling alone would be insufficient to tenderize the rectus abdominis muscle from youthful carcasses without additional postmortem aging (Morrow et al., 2019). However, the recent study by Tuell et al. (2022) found that the combination of tumbling for 120 min with 10 additional days of postmortem aging would improve the subjective tenderness liking of semitendinosus steaks, although WBSF values would be unaffected. Further, the study by N’Gatta et al. (2021) found that extended tumbling durations could influence the textural properties of the myofibers and connective tissue of the semitendinosus from cows. Regarding the longissimus lumborum from dairy cows, recent results from Nondorf et al. (2022) found that tumbling for 90 min would increase consumer tenderness liking and overall liking without additional postmortem aging. However, with further aging, the benefits would be negligible (Nondorf et al., 2022). These benefits would be mostly attributable to tumbling causing considerable fragmentation to the myofibrils, while intramuscular connective tissues would be mostly unaffected (Nondorf and Kim, 2022; Tuell et al., 2021; Tuell et al., 2022).
The use of hydrodynamic shockwaves may be an effective means of tenderizing beef from mature carcasses, although the effects have been largely inconsistent in the literature. In the process, meat in vacuum bags would be placed in a tank of water and subjected to shock waves produced from explosives such as trinitrotoluene and pentaerythritol tetranitrate (Marriott et al., 2001). The study by Marriott et al. (2001) found inconsistent results regarding the tenderness of longissimus steaks from cull cows subjected to this treatment. Likely, these discrepancies would be related to hydrodynamic shock waves having no impact on the concentration and properties of collagen (Marriott et al., 2001). Similar findings were reported by Schilling et al. (2003) where hydrodynamic shockwaves resulted in a numerical decrease in WBSF of the longissimus lumborum but no considerable impact on sensory tenderness scores. However, hydrodynamic shockwaves used in conjunction with blade tenderization was shown to benefit instrumental but not sensory tenderness (Schilling et al., 2003). Accordingly, the process may have some positive impact on tenderization, although the benefits may not outweigh detriments such as the loss of an adequate vacuum seal of the packaging (Marriott et al., 2001).
High-intensity, high-frequency ultrasonication has also been examined as a method to influence the tenderness attributes of mature beef muscles. During ultrasonication, ultrasonic waves are applied to a food, resulting in several physical and biochemical changes (Jayasooriya et al., 2004). Got et al. (1999) found that this treatment when applied prior to the onset of rigor would result in cull cow semimembranosus muscles with considerably longer sarcomeres (2.20 versus 1.92 μm); however, no impact on sarcomere length would be observed if ultrasonication were applied post-rigor. Further, the treatment was shown to increase the amount of free calcium and promote the degradation of myofibrillar structure. Likely owing to the high amount of insoluble collagen which ultrasonication had no impact on, the overall effect on tenderization during postmortem aging was minimal (Got et al., 1999). However, a recent study by Wang et al. (2022) indicated that power ultrasonication could positively impact the tenderness of semitendinosus from younger carcasses by increasing the activities of cathepsins B and L and increasing the solubility of collagen. In contrast to high-intensity, high-frequency ultrasonics, power ultrasound would use greater watts but low frequency (Jayasooriya et al., 2004). Further research regarding if power ultrasonication would be feasible for applications in mature beef muscles would be necessary, although the potential to impact the intramuscular connective tissues would be desirable.
High-pressure processing may be another feasible method for improving the tenderness of mature beef. Using the hot boned longissimus thoracis and gluteus medius muscles from culled cows and bulls, Morton et al. (2017) found that high-pressure processing at pressures of 175 and 250 MPa would be beneficial for tenderization. For the longissimus thoracis of cull cows, processing at 175 and 250 MPa would result in an immediate 5.8 to 5.9 kg decrease in shear force, respectively. Twenty-eight days of aging was necessary to achieve similar values for tenderness, thus high-pressure processing could be beneficial to reduce the necessary duration of postmortem aging. However, higher pressures (250 MPa) could potentially impact color through lightening of the meat (Morton et al., 2017).
Another promising method for improving the tenderness development of mature beef cuts would be the use of pulsed electric fields. During the process, brief electric pulses would be applied to a food between two electrodes (Bhat et al., 2019a). The electric field generated may then result in profound changes to the structure of the myofibers, such as an increase in the permeability of the cell membrane. In studies of the biceps femoris (Bhat et al., 2019b) and from culled dairy cows (Bhat et al., 2019c), it has been shown that pulsed electric field would result in an increase in calpain-2 activity during early postmortem aging, thereby increasing the degradation of myofibrillar proteins including troponin-T and desmin. However, the existing literature on pulsed electric field on cull cow beef have shown its potential impact to improve tenderization to be limited (Bhat et al., 2019b; Bhat et al., 2019c). This would likely be related to the high extent of background toughness of round muscles from mature carcasses being the driving factor for the lack of tenderness development. Regardless, considering the positive impacts on the degradation of myofibrillar proteins, pulsed electric field could be applicable in some circumstances where less connective tissue is present (i.e., cuts from the loin/rib primals) or used in conjunction with another post-harvest intervention.
Numerous functional ingredients have been incorporated into brine and marinade solutions aimed at improving the quality and palatability attributes of cull cow beef. Often, these would include a mixture of sodium chloride and phosphates (Hoffman, 2006; Holmer et al., 2009; Pivotto et al., 2014). Both instrumental and sensory tenderness have been shown to improve with the application of brine solutions containing sodium chloride and phosphates across various muscles like the longissimus, semitendinosus, semimembranosus, and others (Hoffman, 2006; Holmer et al., 2009; Pivotto et al., 2014). Holmer et al. (2009) found that a brine containing sodium chloride and phosphate would improve the sensory tenderness scores of all ten muscles evaluated, and WBSF would be decreased in all muscles except the adductor. Similarly, sodium chloride and sodium tripolyphosphate brine has been shown to improve the instrumental tenderness of hot boned longissimus lumborum and semimembranosus muscles from cull cows (Pivotto et al., 2014). The study by Hoffman (2006) found that enhancement significantly improved instrumental tenderness from 50.4 to 36.7 N in the longissimus and from 48.7 to 42.0 N in the semitendinosus. This resulted in trained sensory panelists rating the enhanced muscles as greater for tenderness with less residue after chewing. The positive impacts of enhancement were more apparent in the longissimus compared to the semitendinosus, although the process was overall beneficial to both cuts (Hoffman, 2006).
Considering the role of calpains in the postmortem tenderization of beef (Kemp et al., 2010; Koohmaraie, 1992; Koohmaraie, 1994; Warner et al., 2022), numerous studies have utilized brines including calcium chloride (Bunmee et al., 2014; Diles et al., 1994; Eilers et al., 1994; Kerth et al., 1995; Kong et al., 2006; Morgan et al., 1991; Morris et al., 1997; Whipple and Koohmaraie, 1993) or calcium ascorbate (Pivotto et al., 2014) to improve the tenderness of cull cow beef. In general, the effects of calcium chloride enhancement have been shown to be positive on tenderization across various muscles including the longissimus, gluteus medius, and semimembranosus (Bunmee et al., 2014; Eilers et al., 1994; Kerth et al., 1995; Kong et al., 2006; Morgan et al., 1991; Morris et al., 1997), although Whipple and Koohmaraie (1993) found no impact on WBSF values. Similarly, Diles et al. (1994) found that calcium chloride would have no impact on instrumental tenderness, while sensory tenderness scores would be improved. Discrepancies in the effectiveness of calcium chloride enhancement are likely highly influenced by the postmortem timepoint at which the injection occurs. Highlighting this, the study by Whipple and Koohmaraie (1993) did not inject until 5 days postmortem, during which no activity of calpain-1 was detected. In contrast, several of the aforementioned studies injected calcium chloride into hot boned muscles (Bunmee et al., 2014; Diles et al., 1994; Eilers et al., 1994; Kong et al., 2006; Morgan et al., 1991; Morris et al., 1997). While this could result in the shortening of sarcomeres (Morgan et al., 1991), it is likely that greater myofibrillar fragmentation during the aging process would outweigh this in general (Bunmee et al., 2014; Kong et al., 2006). Contesting this, however, Pivotto et al. (2014) found that calcium ascorbate enhancement in hot boned longissimus lumborum and semimembranosus muscles from cull cows would result in considerable shortening of the sarcomeres and subsequently greater toughness.
Several studies have examined the use of calcium chloride along with lactic acid, generally showing them to be synergistic for tenderization (Cruzen et al., 2015; Eilers et al., 1994; Morris et al., 1997). Highlighting this, Eilers et al. (1994) found that calcium chloride and lactic acid together would result in the greatest decrease in WBSF values of semimembranosus muscles, which would be maintained over the course of 17 days of postmortem aging. Regarding the effects of lactic acid only, several studies have reported that lactic acid would decrease pH, increase the activity of lysosomal enzymes including cathepsins B and L, and contribute to the degradation of myofibrillar and connective tissue structure (Berge et al., 2001; Ertbjerg et al., 1999). Particularly, lactic acid has been shown to increase the heat solubility of collagen from pectoralis profundus muscles from cull cows, as well as promote the degradation of myofibrillar proteins including myosin heavy chain and actin (Berge et al., 2001). While having positive impacts on tenderization, some studies have reported negative impacts on flavor attributes with the use of acidic marinades, such as sour notes (Eilers et al., 1994; Morris et al., 1997; Pivotto et al., 2014). This could be mitigated to a certain extent with the use of other flavorings in the solution (Morris et al., 1997).
A recent innovation in enhancement research would be the use of vacuum impregnation as a method to alter the textural attributes of beef. Vacuum impregnation has been identified as a method to incorporate a solution into a food through the use of vacuum and atmospheric pressures (Salvatori et al., 1998). The study by Leal-Ramos et al. (2018) found that vacuum impregnation could be used as a method to evenly distribute brine solution throughout the longissimus of cull cows. Scanning electron microscopy images found that vacuum impregnation would increase the amount of myofibrillar swelling (Leal-Ramos et al., 2018). Although textural attributes were not assessed, it is reasonable to postulate that the process may be beneficial to tenderness as well. Tenderness and water-holding attributes are often closely related owing to the plasticizing effect of water on muscle proteins (Hughes et al., 2014). Supporting this, multiple studies on enhancement alone without a physically disruptive process to aid in brine penetration (e.g., tumbling) have observed benefits to water-holding, juiciness, and tenderness attributes (Gao et al., 2015; Hoffman, 2006; Holmer et al., 2009; Morrow et al., 2019).
Summary and Conclusion
Beef from mature carcasses presents multiple challenges to producers to deliver consistently high-quality products to consumers. However, various post-harvest interventions have resulted in cull cow beef that may meet or exceed consumer expectations. These innovations typically target one or more of the driving factors influencing beef tenderness including postmortem proteolysis, background toughness, and sarcomere length (Warner et al., 2022). In general, cull cow beef would be categorized as having a lower extent of postmortem proteolysis (Cruzen et al., 2014; Xiong et al., 2007), a high concentration of highly cross-linked, insoluble collagen (Alvarenga et al., 2021; Dos Santos Fontes et al., 2021; Jurie et al., 2007), and potentially shorter sarcomeres if rapid chilling occurs (Gredell et al., 2018; Lucero-Borja et al., 2014). Post-harvest processing techniques during carcass chilling (e.g., electrical stimulation, delay chilling, hot boning, skeletal separation, vacuum impregnation; Table 1), postmortem aging (e.g., wet aging, dry aging; Table 2), physical interventions (e.g., blade/needle tenderization, tumbling, hydrodynamic shockwaves, ultrasonication, high-pressure processing, pulsed electric field; Table 3), and enhancement with functional ingredients have great promise in improving the tenderness and palatability traits of beef from mature carcasses (Table 4). However, it is also important to note that the palatability of beef from older animals is not only dependent on tenderness but also flavor and juiciness. Thus, it is important that gains in tenderness through these interventions do not adversely impact other eating quality traits. Regardless, these processing interventions may be viable to make beef from mature carcasses more suitable for intact, whole muscle cuts and thus add value to producers.
Source | Muscle studied | Chilling treatment | Outcomes |
---|---|---|---|
McKeith et al. (1980) | Longissimus | Electrical stimulation | Decreased shear force. |
Sonaiya et al. (1982) | Longissimus, semimembranosus, triceps brachii | Electrical stimulation | Decreased shear force but no impacts on myofibrillar fragmentation and troponin-T degradation. |
Hawrysh and Wolfe (1983) | Longissimus, semitendinosus | Electrical stimulation | No impact on shear force and subjective tenderness. |
Boleman et al. (1996) | Longissimus | Electrical stimulation | Decreased shear force for cattle on feed for 0, 28, and 56 d. No impact on shear force for cattle on feed for 84 d. |
Fields et al. (1976) | Longissimus, sternomandibularis | Delay chilling | Lengthened sarcomeres resulting in lower shear force and greater sensory tenderness. |
Phoya and Will (1986) | Longissimus, psoas major, quadriceps femoris | Delay chilling and hot boning | Increased shear force and decreased sensory tenderness of all muscles. |
Berry and Cross (1982) | Longissimus, semimembranosus | Hot boning | Shortened sarcomeres of longissimus but not semimembranosus. Decreased collagen solubility and increased shear force. |
Will and Beaty (1986) | Longissimus, psoas major, quadriceps femoris | Hot boning | Increased shear force of longissimus but not psoas major and quadriceps femoris. |
Eilers et al. (1994) | Semimembranosus | Hot boning | Increased shear force without enhancement but decreased shear force with enhancement. |
Pivotto et al. (2014) | Longissimus lumborum, semimembranosus | Hot boning | Increased shear force and sensory toughness of longissimus lumborum without enhancement. Hot boning was beneficial to tenderness when used with sodium tripolyphosphate. |
Mandell et al. (2006) | Longissimus lumborum, longissimus thoracis, semimembranosus, semitendinosus, vastus lateralis, biceps femoris, rectus femoris | Skeletal separation | Decreased shear force of the anterior longissimus lumborum, longissimus thoracis, and semimembranosus but not other muscles. |
Streiter et al. (2012) | Longissimus lumborum, longissimus thoracis, semimembranosus, semitendinosus | Skeletal separation | Lengthened sarcomeres and decreased shear force of the longissimus lumborum and longissimus thoracis but not semimembranosus and semitendinosus. |
Kethavath et al. (2022) | Longissimus lumborum, semimembranosus, triceps brachii | Vascular chilling | Decreased shear force of the longissimus lumborum supported by lengthened sarcomeres. |
Source | Muscle studied | Aging treatment (d) | Outcomes |
---|---|---|---|
Mandell et al. (2006) | Longissimus lumborum, longissimus thoracis, semimembranosus, semitendinosus, vastus lateralis, biceps femoris, rectus femoris | Wet aging (2, 7, 14, 28) | Decreased shear force of the longissimus lumborum, longissimus thoracis, semimembranosus, vastus lateralis, and rectus femoris but not semitendinosus and biceps femoris. Consistent aging response in longissimus lumborum and longissimus thoracis but limited tenderization past 7 d for other muscles. |
Xiong et al. (2007) | Longissimus | Wet aging (1, 3, 10) | Decreased shear force throughout aging period. Less troponin-T degradation among older carcasses. |
Franco et al. (2009) | Longissimus thoracis | Wet aging (1, 7, 14, 21, 35, 42) | Decreased shear force during initial aging period but additional tenderization not found past 14 d. |
Streiter et al. (2012) | Longissimus lumborum, longissimus thoracis, semimembranosus, semitendinosus | Wet aging (7, 14, 21) | Decreased shear force of the semitendinosus but not semimembranosus from 7 to 14 d. Shear force of the longissimus lumborum and longissimus thoracis dependent on skeletal separation and enhancement. |
Vitale et al. (2014) | Longissimus thoracis et lumborum | Wet aging (0, 3, 6, 8, 14, 21) | Decreased shear force at 3, 8, 14, 21 d of aging compared to 0 and 6 d. |
Bhat et al. (2018a) | Semimembranosus, biceps femoris | Wet aging (1, 2, 7, 14) | Decreased shear force throughout aging period supported by increased myofibrillar fragmentation. |
Alvarenga et al. (2021) | Longissimus lumborum, semitendinosus | Wet aging (2, 14, 28) | Decreased shear force of longissimus lumborum but not semitendinosus from 2 to 14 d. By 28 d, only 7.8% of longissimus lumborum steaks from cull cows categorized as tough. |
Obuz et al. (2014) | Longissimus lumborum | Dry or wet aging (2, 23) | Overall tenderness scores improved with aging regardless of aging type, but wet aging had greater overall tenderness to dry aging. |
Setyabrata et al. (2022) | Longissimus lumborum | Dry or wet aging (28) | Aging type had no impact on shear force or sensory tenderness. |
Source | Muscle studied | Physical treatment | Outcomes |
---|---|---|---|
Tatum et al. (1978) | Longissimus, biceps femoris, semimembranosus | Blade tenderization | Increased overall tenderness of longissimus but limited impact on shear force across muscles. |
Obuz et al. (2014) | Longissimus lumborum | Blade tenderization | Increased myofibrillar tenderness when used with wet but not dry aging. |
N’Gatta et al. (2021) | Semitendinosus | Tumbling | Reduced myofibrillar and connective tissue toughness. |
Nondorf et al. (2022) | Longissimus lumborum | Tumbling | Increased sensory tenderness for unaged steaks only. No impact on shear force or myofibrillar fragmentation. |
Marriott et al. (2001) | Longissimus | Hydrodynamic shock wave | Inconsistent effects on instrumental and sensory tenderness. |
Schilling et al. (2003) | Longissimus lumborum | Hydrodynamic shock wave, blade tenderization | Decreased shear force through both hydrodynamic shock waves and blade tenderization. |
Got et al. (1999) | Semimembranosus | High-intensity, high-frequency ultrasound | Lengthened sarcomeres and released free calcium when applied pre-rigor but had no impact on myofibrillar resistance of raw meat. Application post-rigor decreased myofibrillar resistance on day 6 only but did not impact sarcomere length. |
Morton et al. (2017) | Longissimus thoracis, gluteus medius | High-pressure processing | Decreased shear force and improved sensory tenderness. |
Bhat et al. (2019b) | Biceps femoris | Pulsed electric field | No impact on shear force or myofibrillar fragmentation but increased calpain-2 activity and proteolysis of myofibrillar proteins. |
Bhat et al. (2019c) | Semimembranosus | Pulsed electric field | No impact on shear force or myofibrillar fragmentation but increased calpain-2 activity and proteolysis of myofibrillar proteins. |
Source | Muscle studied | Enhancement treatment | Outcomes |
---|---|---|---|
Hoffman (2006) | Longissimus, semitendinosus (cold boned) | Commercial mix including sodium chloride, sodium lactate, sodium tripolyphosphate, etc. | Decreased shear force and increased sensory tenderness. |
Holmer et al. (2009) | Longissimus, adductor, complexus, gracilis, pectineus, psoas major, rectus femoris, semimembranosus, serratus ventralis, vastus lateralis (cold boned) | Sodium chloride, phosphate | Increased sensory tenderness in all muscles and decrease shear force in all muscles except adductor. |
Pérez-Juan et al. (2012) | Semimembranosus, semitendinosus (cold boned) | Sodium chloride, sodium lactate, lactose, sodium ascorbate | Decreased shear force. |
Morgan et al. (1991) | Longissimus, gluteus medius, semimembranosus (hot boned) | Calcium chloride | Decreased shear force and sensory tenderness despite shorter sarcomeres. |
Whipple and Koohmaraie (1993) | Longissimus, semimembranosus (cold boned) | Calcium chloride | No impact on shear force. |
Diles et al. (1994) | Longissimus (cold and hot boned) | Calcium chloride | Increased sensory tenderness but no impact on shear force. |
Eilers et al. (1994) | Semimembranosus (hot boned) | Calcium chloride, lactic acid | Decreased shear force. |
Kerth et al. (1995) | Longissimus (cold boned) | Calcium chloride | Decreased shear force. |