Introduction
Of all quality characteristics of meat, tenderness has been regarded by consumers as the most important characteristic (Kemp et al., 2010). Meat tenderness is the degree of ease of meat chewability, and it indicates not only physical strength required to cut the meat, but also its palatability because of the softness when consumed. Meat tenderness is a quality parameter that is affected by various other factors including chemical state of myofibrillar proteins, amount of connective tissue, post-mortem handling, and the amount of moisture and fat in meat (Lawrie, 1998). The various meat tenderization techniques include dry/wet aging, sous-vide as well as high pressure processing (Choi et al., 2016; Hwang et al., 2019; Oh et al., 2017). However, these techniques focus on modification of the physicochemical state of meat proteins, thereby reducing the shear value of treated meat.
Post-mortem proteolytic action triggers weakening of muscle tissue (Kemp et al., 2010), and there have been trials in which extrinsic proteases were used to accelerate or intensify meat tenderization. Although enzymatic tenderization is not adopted by the meat industry because of the difficulty to control the enzymatic action, this is a simple way to produce tender meat for consumers without using special devices. Among various proteases, papain and bromelain, which are naturally available in fruits such as papaya and pineapple, have been identified to hydrolyze meat proteins, thereby acting as effective meat tenderizers (Ha et al., 2013). Nevertheless, high proteolytic activity of these enzymes can produce mushy meat, which negatively affects the consumers’ preference. Consequently, enzyme tenderization is not favorable at the conditions in which meat must be further stored. To regulate the enzymatic action, therefore, it is necessary to select an enzyme that has less proteolytic activity (Ashie et al., 2002) or delays enzymatic action using encapsulation (Gibbs et al., 1999).
Classically, enzymes are encapsulated to immobilize their activity under a certain environmental condition. Various hydrocolloid substances including alginate beads or chitosan membrane have been widely adopted as coating materials of the enzymes (Srivastava et al., 2005). However, these systems lack control over the release rate of the enzyme into the substrate. In this case, double emulsion (DE) is a better system not only to encapsulate hydrophilic substances such as proteases, but also to control the release rate of enzymes (Fuentes et al., 2003). Despite numerous investigations on enzymatic meat tenderization, little information was available regarding the effect of encapsulated protease and storage on the meat quality. Therefore, we investigated the effects of bromelain liberated or embedded in DE on the eating palatability of pork loin.
Materials and Methods
Pork loin was purchased from a local market (My Hometown Livestock, Seoul, Korea) at 48 h postmortem. Bromelain BR1200 (cysteine protease from pineapple stem, optimal pH: 4.0–7.0, optimal temperature: 65°C–75°C) was obtained from Bision Biochem (Sungnam, Korea). Canola oil (Sajo Haepyo, Seoul, Korea) was used as the oil phase. Tween® 80 which is nonionic and aliphatic surfactant that derived from oleic acid and polyethoxylated sorbitan (Krstonosic et al., 2019). Tween® 80 (Samchun Pure Chemical, Seoul, Korea) was used as the outer aqueous phase emulsifier. Food polyglycerol polyricinoleate (PGPR, MASEMUL™ PGPR 9090) purchased from Musim Mas Ltd. (Indonesia) was used as the oil phase emulsifier. Sodium chloride was purchased from Duksan Pure Chemical (Ansan, Korea).
A DE containing bromelain in the inner aqueous phase was prepared by a two-step emulsification process. To fabricate water-in-oil emulsion (W1/O), canola oil phase (O) was mixed with 5% (w/v) PGPR using a propeller stirrer (Chang shin, Seoul, Korea) at 500 rpm for 30 min and internal water phase (W1) comprising 1% (w/v) NaCl and 6.667% (w/v) bromelain. The total bromelain concentration was 1% (w/v) in the final DE volume. For W1/O emulsion, 7:3 ratio of oil phase and water phase was dispersed by drop-wise method using a propeller stirrer at 700 rpm for 3 min and mixed using a high-speed homogenizer (T25 digital Ultra-turrax® high-speed mixer, IKA, Staufen, Germany) at 15,000 rpm for 5 min.
The fabricated primary emulsion was dispersed in secondary water phase (W2), which comprised 1% (w/v) Tween® 80, using a propeller stirrer at 700 rpm for 3 min and mixed using a high-speed homogenizer at 10,000 rpm for 3 min. The ratio of the primary emulsion (W1/O) and secondary water phase (W2) was 1:1 (Sapei et al., 2012).
The zeta potential of blank DEs and bromelain loaded in double emulsion (DE E) was measured by dynamic light scattering using a Zetasizer Nano ZS® (Malvern Instruments, Worcestershire, UK). Before zeta potential measurement, the samples were diluted to 1:200 using distilled water (DW) to avoid multiple scattering effects. The particle size of blank DEs and DE E was also determined by dynamic light scattering method using a Mastersizer 3000E® (Malvern Instruments, Worcestershire, UK). For measuring the particle size, the sample was dispersed in DW to meet certain conditions, such as continuous phase. The microstructure images of blank DE and bromelain embedded in DE were observed using an optical trinocular microscope (CX 31RTSF, Olympus Optical Co, Ltd., Japan). A drop of diluted emulsion was placed on a slide glass and covered with a cover glass. The emulsion was observed with a 400× and 1,000× lens.
The form of sample was cut into by method of Kim et al. (2015c). At least 3 pieces of pork loin (3×3×1 cm, 10 g) were prepared for each characterization. The samples were soaked in 40 mL DW, DW with 1% (w/v) bromelain solution (DW E), DE, and DE E, respectively. Raw pork loin was used as control. The samples were stored at 4°C for 24, 48, and 72 h. Physicochemical properties were analyzed after removed treatment solution and DE every reaction time.
The color of samples was measured using a color reader (CR-400, Konica Minolta Sensing Inc., Osaka, Japan), calibrated with a white standard plate (CIE L*=97.83, CIE a*=0.43, CIE b*=1.98). The CIE L*, a*, and b* values were determined as indicators of lightness, redness, and yellowness, respectively. The tests were performed in triplicate from the surface of each sample. The total color difference (ΔE) between the control and treated samples was calculated (Benjar and Athapol, 2007).
One gram of sample was weighted and placed in a 15 mL conical centrifuge tube along with a gauze as an absorbent and centrifuged at 8,000 rpm for 10 min at 4°C. The meat was removed from the tube and water holding capacity was calculated using the following formula:
The control and treated samples, which were treated for 0, 24, 48, and 72 h, were weighed before heating. The samples were packed using polyethylene bag and vacuum sealed. The sealed packages were cooked in a water bath at 75°C for 30 min and cooled at room temperature (25°C) for 30 min. Cooking loss values (%) were calculated using the following formula:
The moisture content of samples was determined by oven drying, according to the AOAC (2012) method No. 979. 12. The samples were dried at 105°C and weighed again until constant weight was reached. The moisture content was calculated using the following formula:
The pH values of samples were measured in triplicate with a pH meter (S-220, Mettler-Tol-edo Co., Zurich, Switzerland). To measure pH, 5 g of sample was mixed with 20 mL of DW and homogenized at 15,000 rpm for 15 s using a homogenizer (Silent crusher M, Heidolph Instruments, Schwabach, Germany).
Warner-Bratzler shear force (WBSF) was measured by a slight modification of the method described by Kim et al. (2015c). The WBSF of control and treated samples, treated for 24, 48, and 72 h, was measured using a texture analyzer (CT3-1000, Brookfield Engineering Laboratories, Inc., Middleboro, MA, USA) using a v-type plain probe. The cooked samples were cut to 0.5×0.5×2 cm in parallel to the muscle fiber. The samples were positioned the center of the probe and measured the shear force using the following instrument settings: measured force in compression type, 10 kg force load cell, test speed of 2 mm/s, target deformation of 15%, and trigger load of 100 g. The measurements were performed at least 5 times.
The myofibrillar protein solubility was determined using the difference between total protein solubility and sarcoplasmic protein solubility. For total protein solubility measurement, 2 g of sample was homogenized with 20 mL of ice-cold 1.1 M potassium iodide in 0.1 M potassium phosphate buffer (pH 7.4) using a homogenizer. For sarcoplasmic protein solubility, 2 g of sample was mixed with 20 mL of ice-cold 0.025 M potassium phosphate buffer (pH 7.4) using a homogenizer. These mixtures were kept at 4°C overnight, after which the mixtures were centrifuged at 6,000 g for 15 min at 4°C. The supernatant was filtered using Whatman No. 1 filter paper. The total protein solubility and sarcoplasmic protein solubility were determined through protein concentrations by biuret method. In biuret reaction, bovine serum albumin was used as standard (Gornall et al., 1949). The myofibrillar protein solubility was calculated using the following formula:
Sodium dodecyl sulfate-polyacrylamide gel electrophoresis (SDS-PAGE) was performed according to a slight modification of the method by Sunantha and Saroat (2011). For SDS-PAGE, 2 g of sample was mixed with 18 mL of 5% (w/v) SDS solution (85°C) and homogenized at 15,000 rpm for 15 s using a homogenizer. The homogenized sample was incubated in water bath at 85°C for 1 h and then centrifuged for 5 min at 8,000 g at 25°C. The supernatants were mixed in 0.5 M Tris–HCl sample buffer (pH 6.8) containing 4% SDS, 20% glycerol and 10% 2-mercaptoethanol, and boiled for 3 min. Then, 20 μL of sample was loaded into a polyacrylamide gel (12% running gels and 5% stacking gels) using a Mini Protean Tetra Cell unit (BioRad Lab., Hercules, USA). The loaded gel was stained with 0.02% (w/v) Coomassie Brilliant Blue R250 (B7920, Sigma, USA) in 50% (v/v) methanol and 7.5% (v/v) acetic acid. The gel was destained with destaining solution 1 of 40% methanol and 7% acetic acid for 30 min and then destained overnight in destaining solution 2 of 15% methanol and 7% acetic acid. The separated protein bands of sample were compared with standard protein marker (Precision Plus Protein Standards, BioRad Lab., Hercules, USA).
All experiments were performed in triplicate and reported values were the average of the replicated experiments. Statistical analysis was performed using SPSS Statistics version 24.0 for windows (Statistical Package for the Social Science, Ver. 24.0 IBM., Chicago, IL, USA). To confirm significant differences among treatments, one-way analysis of variance (ANOVA) and Duncan’s multiple range test was carried out at the 95% confidence level (p<0.05).
Results and Discussion
The overall droplet size of DE E was slightly smaller than that of blank DE (p<0.05; Fig. 1A). However, the absolute value of zeta potential of DE E was decreased compared to blank DE (Fig. 1B, p<0.05). The characteristics of bromelain-loaded DE probably resulted from the surface charge of bromelain. Bromelain was positively charged in our experimental condition, with an isoelectric point of pH 9.55 (Yamada et al., 1976). The emulsifier coated on the emulsion droplets was negatively charged; hence, the electrostatic attraction between the surface and core resulted in protein-polysaccharide interactions, which led to smaller emulsion droplets (Dickinson, 1998; Turgeon et al., 2003). However, small amounts of enzymes were adsorbed onto the droplet surface during emulsification, thereby causing a change in the latter zeta-potential. Nevertheless, these changes were not drastic, suggesting that the loading of bromelain would not affect the physicochemical properties of emulsion droplets. The microstructure of DE is shown Fig. 1C. The result shows the morphology of stable emulsion structure in all emulsion treatments. Spherical dark droplet indicates encapsulation of bromelain. There are darker W1/O droplets in the DE of 1% (w/v) bromelain.
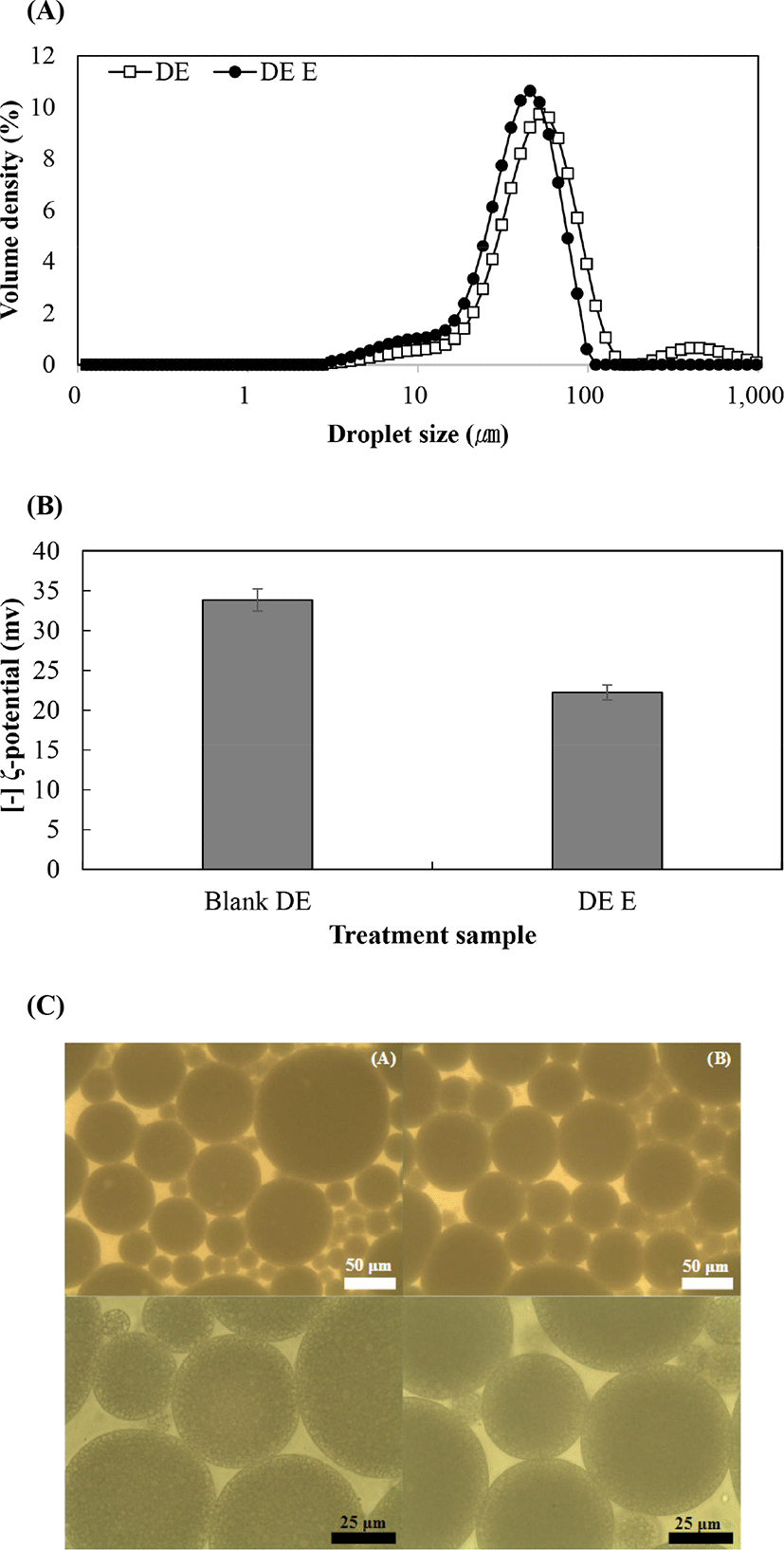
To analyze the effect of DE E on meat, the color properties resulting from each treatment were measured (Table 1). All groups of samples had higher CIE L*-value than the fresh control (0 h), and the CIE L*-value further increased with increasing immersion time (p<0.05). The lighter color of meat immersed in water has been reported in literature (Xia et al., 2012) and is known to result from moisture absorption in meat. However, immersion of meat in water (DW and DW E) showed greater increase in L-value than DE treatments (DE and DE E) (p<0.05). Meat surface was probably coated by DE droplets, which possibly prevented excessive moisture absorption. For redness (CIE a*), DE E showed no significant difference on increasing the reaction time; however, there was slight tendency of decreasing redness compared to that in control (p<0.05). For yellowness (CIE b*), there was no significant difference until reaction time of 48 h compared to the control (0 h). A significant difference was observed in DE E in 72 h (p<0.05). Total color differences showed higher values in DW and DW E, whereas DE and DE E showed lower values at all reaction times compared to DW-based treatment samples. Based on the results, DE effectively reduced the color change in pork loin during marination. The former was because of the accumulation of emulsion droplets on the meat surface whereas the latter is not yet fully understood. Nevertheless, the action of bromelain affects the color because this effect in DW E was not seen when the bromelain was encapsulated (DE E).
0 h data of each treatment in same experimental data is same because 0 h reaction time means non treated.
A-D Means with different capital letters are significantly different (p<0.05) when comparing treatment type at each time.
Water holding capacity of meat is defined as the ability of meat to retain intrinsic or added moisture within its internal muscle structure and prevent the release of water under external influences such as centrifugation, force, or heat. Water holding capacity could be related to physical properties such as texture, color, and appearance (Sunantha and Saroat, 2011). In this study, the water holding capacity of each treatment group was evaluated to analyze the effect of meat immersed in DE E for 0, 24, 48, and 72 h (Table 2). Bromelain hydrolyzed proteins into small molecular peptides, resulting in reduced myofibrillar proteins to shrink and trap water in the extra-cellular space (Sunantha and Saroat, 2011). Overall, a decrease in the water holding capacity was observed in all treatment groups except control (p<0.05). DE E showed the highest water holding capacity values among treated samples, except the control in the overall reaction time compared with other treatments, but there were no significant differences. The water holding capacity of hydrolyzed sample showed lower values compared to controls in other studies. WHC is an important indicator of eating quality was highest in control. (den Hertog-Meischke et al., 1997). In this study, the water holding capacity values except for control, showed a significant decline at 24 h and the values continuously increased till 72 h. It is indicated that proteolytic hydrolysis of meat protein was increased compared to 48 h and 72 h. Especially, DE and DE E, groups with DE treatment, exhibited higher water holding capacity values than DW and DW E groups with DW treatment, perhaps due to NaCl addition to the inner aqueous phase to improve stability of DE. Water holding capacity results, which were affected by the internal aqueous phase surfactant NaCl, suggest that DE acts as a carrier system and delivers encapsulated substance to the inner and outer phases. According to Kim et al. (2015b), water holding capacity of chicken breast muscle increased with increasing amount of NaCl. Boles and Swan (1997) reported that the salt level of meat could affect the net charge of internal muscle protein and lead to alteration in muscle protein solubility. Thus, the oil phase of DE could affect the water holding capacity values of meat samples treated with DE.
0 h data of each treatment in same experimental data is same because 0 h reaction time means non treated.
A-D Means with different capital letters are significantly different (p<0.05) when comparing treatment type at each time.
Cooking loss of meat is defined as the loss of liquids, such as moisture and soluble substances during cooking because of the denaturation of protein and decomposition of cell membranes (Mudalal et al., 2014). The effect of DE E on the cooking loss of meat was analyzed for 0, 24, 48, and 72 h (Table 2). In this study, the cooking loss of the order DW E>DW>DE>DE E was observed at all reaction times except for control. DW treatment samples showed higher cooking loss than DE treatment samples due to larger amount of moisture absorption. Meanwhile, cooking loss of DE E treatment was lower than that of DW E treatment. It would be explained that partially hydrolyzed protein has more hydrophilic nature, and thereby, Koh et al. (2019) studied enhancing water binding properties such as cooking loss in this study. All treatments except for DE and DE E were significantly different at all reaction times (p<0.05). The cooking loss value was observed to be the highest in DW E and was less in other treatments (p<0.05), with a significant increase in cooking loss values of DW E according to the reaction time (p<0.05). Initial DW E treatment showed high moisture absorption during marination, and rapid enzymatic hydrolysis. Upon cooking, therefore, most absorbed moisture was lost because of structural degradation of muscle tissue and resulted in the highest cooking loss among treatments (Xiong et al., 2012). The treatments of meat immersed in DE base (DE, DE E) showed lower cooking loss values than water base treatment (DW, DW E) perhaps because of the oil in DE. Fibers and fibrils in meat were coated with soaked oil. Sunantha and Saroat (2011) suggested that the cooking loss values indicated and were related to the bromelain hydrolysis level. Pawar et al. (2007) reported that the increase in cooking loss of treated samples when compared with control was caused by myofibrillar and sarcoplasmic protein degradation.
To analyze the effect of DE E on meat moisture content values of meat were measured at each reaction time of 0, 24, 48, and 72 h (Table 2). The range of moisture content in the treatment group was 72.32%–76.01%. There was significant difference (p<0.05) based on the immersion substance used (DW and DE), but no significant difference with moisture content was observed in the treatment groups DW, DW E, DE, and DE E, except at 24 h treatment. In this study, the moisture content in all treatment groups was increased compared to the values at 0 h treatment (71.348±0.071%). According to Sunantha and Saroat (2011), the results showed that the moisture content of beef, chicken, and squid-sprinkled bromelain powder decreased when the concentration of bromelain increased. In this study, however, pork loin was immersed in various marination conditions; hence, moisture absorption would increase the moisture content with increasing reaction time. In DE treatments, moisture absorption was not obvious when compared to that in water treatments, which was also explained by surface coating of emulsion droplets. Conversely, bromelain alone did not affect the moisture content of pork.
According to Goli et al. (2007), the highly important properties of pH could affect water holding capacity, tenderness, and juiciness. Fig. 2A shows the result of the influence of DE E on pH for reaction times 0, 24, 48, and 72 h. The pH range of all treatment groups was 5.74–5.96, and the pH of groups with 0 h treatment time was 5.87. Manohar et al. (2016) exhibited that the pH range of sample treated with pineapple extract bromelain was 5.47–6.93. There was a pH order of DW>DW E>DE>DE E until reaction time of 48 h, but, in that of 72 h, pH of DE E was slightly increased in comparison with DE. In the treatment group, DW E and DE E, to which the bromelain was added, the pH was lower than that of each control group,, DW and DE, except for reaction time of 72 h. Sunantha and Saroat (2011) reported that protein was broken down into amino acids by hydrolysis reaction of bromelain enzyme, thereby pH of meat treated with enzymes was decreased. Hence, Gault (1985) suggested that there are possibilities that reason for pH changes, which was caused by postmortem metabolism and substance application in meat during technological process. DE and DE E samples, which were based with DE showed the smaller tendency of pH than DW and DW E, which was treated with based DW in 0, 24, 48, and 72 h. It seems that there was oxidation of oil in DE that was delivered to the intramuscular of meat by immersion treatment.
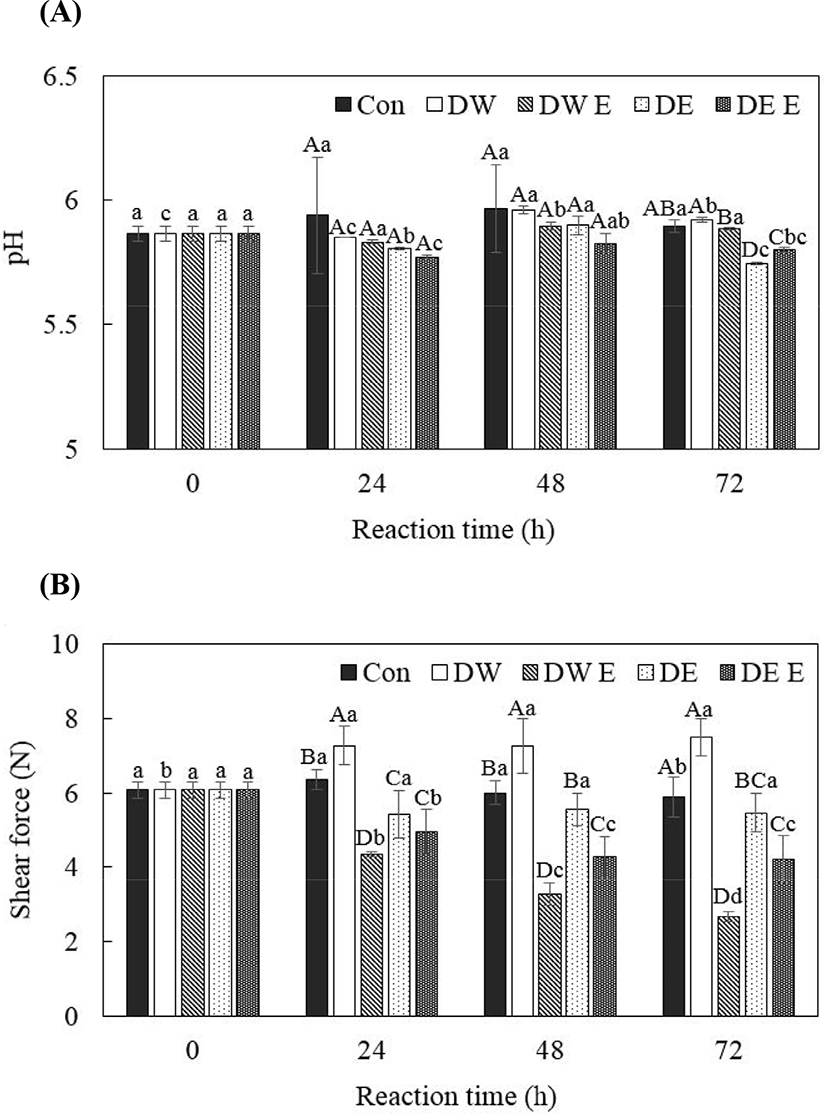
Fig. 2B shows the results of effect of DE E on WBSF for 0, 24, 48, and 72 h. The WBSF values of DW E showed a significant reduction as the reaction time increased, and the lowest value among the overall reaction time compared to other sample groups (p<0.05). Also, it was observed that DE E slightly continuous decrease of WBSF values. DW and DE were not added with any enzyme, but WBSF values of DE was significantly smaller than DE in the overall reaction time (p<0.05). Tenderness of meat is related to the amount of fat present in intramuscular regions. The oil in DE was solubilized or soaked in muscle and that potentiates the sensation of tenderness, not tenderness affected by enzyme proteolysis. The tendency of this result of WBSF was observed in following results of myofibrillar protein solubility.
Similar results were exhibited in WBSF (Fig. 2B) and myofibrillar protein solubility (Table 3). According to Dikeman et al. (1971), there is negative relationship between tenderness and myofibrillar protein solubility. The differences between myofibrillar protein solubility and shear force are properties of tenderness measurement, that is, myofibrillar protein solubility and shear force are chemical and physical analysis for tenderness of meat, respectively. In the result of WBSF, DW and DE showed significant difference in 0, 24, 48, and 72 h (p<0.05), whereas, in the result of myofibrillar protein solubility, there is remarkable gap of result values between DW and DE compared with WBSF. As explained in shear force, it is due to oil phase in DE soaked in intramuscular regions of meat. It caused to lubricate movement in fibers and fibrils in muscle and potentiates to perceive physical tenderness, not by degradation of myofibrillar component. Overall, meat treated with DE E was affected firstly by oil phase in DE, and then, bromelain that slightly released from DE due to interaction with food matrix.
0 h data of each treatment in same experimental data is same because 0 h reaction time means non treated.
A-D Means with different capital letters are significantly different (p<0.05) when comparing treatment type at each time.
Protein solubility is used for determining denaturation of protein and its effects on properties such as water holding capacity and cooking loss (Mudala et al., 2014). According to Mudala et al. (2014), the reduction in myofibrillar protein solubility of white striped chicken breast meat was attributed to denaturation of protein due to its low pH compared to that of the control. Some studies have reported a remarkable connection between myofibrillar protein solubility and water holding capacity, drip loss, cooking loss, and moisture content (Van Laack et al., 1994; Warner et al., 1997). The myofibrillar and sarcoplasmic protein solubility have been measured every 0, 24, 48, and 72 h after immersion in bromelain solution and DE E (Table 3). In this study, DW and DE did not affect the solubility of myofibrillar protein, while bromelain treatments showed a decrease in solubility with increasing reaction time (p<0.05). In particular, DW E showed more rapid decrease in solubility of myofibrillar protein than DE E (p<0.05). However, sarcoplasmic protein solubility of bromelain-treated samples was observed to increase compared with that of samples not treated with bromelain. Protein hydrolysis was manifested by breakage of peptide bonds between amino acids and progressing hydrolysis could be confirmed by lower response to Biuret reaction (Ketnawa and Ogawa, 2018). It could be inferred that in 24 h, enzymes were completely encapsulated by DE, and after 48 h, they started to be released from the inner aqueous phase of DE and have effects on tenderizing meat. Kim et al. (2015a) reported that meat marinated with 2% Sarcodon aspratus, which has proteolytic effect, showed degradation of myofibrillar protein and led to lower myofibrillar protein solubility and shear force than control group and samples treated with kiwi extract. These results suggested that the higher proteolysis level was related to lower myofibrillar protein solubility, and this proteolytic capability affected low shear force. In addition, Shin et al. (2008) reported that high concentration of Sarcodon aspratus extract protease to treat samples revealed lower myofibrillar protein solubility and higher sarcoplasmic protein solubility than other treatments. Hydrophilic proteins could be increased during storage (Ramachandran et al., 2006).
To support changes in meat protein, which were a result of enzyme proteolysis, after each 0, 24, 48, and 72 h meat treatment with enzyme solution and enzymes loaded in DE, SDS-PAGE were performed (Fig. 3). Myosin is the major structural protein component in muscle and is composed of two MHCs (approximately 200 kDa) and four light chains (Schiaffino and Reggiani, 1996). When compared with treatment samples, the MHC band of control, DW, DE, and DE E showed similar patterns, while the MHC patterns of DW E showed remarkably degraded small molecular proteins within 24 h. Moreover, the effect of degradation of MHC in DE E was shown after 48 h, reflecting that DE system delayed the release of bromelain. Thus, DE E could be active in proteolytic hydrolysis after 48 h. After 72 h, one MHC band disappeared, and accumulation of low Mw components continued. Based on the results, catalytic action of bromelain was greatly delayed by encapsulation in DE, which effectively prevented the over-tenderization of pork loin as shown in DW E treatment.
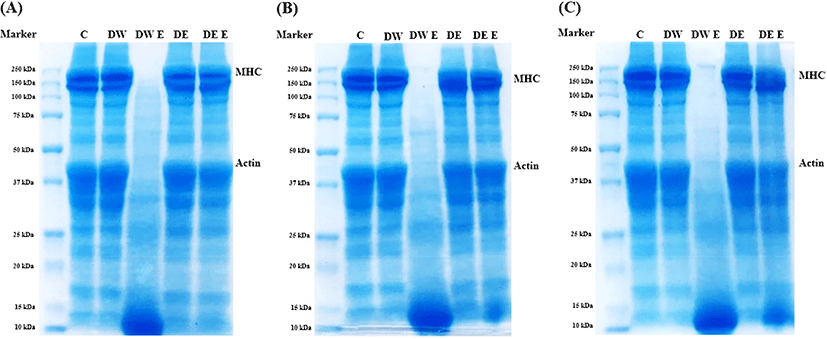
Conclusion
Based on the results, both DE system and bromelain had positive effects on the physicochemical properties of pork loin. Double emulsion was effective in suppressing meat decoloration during marination. Bromelain caused hydrolysis of meat proteins, which resulted in better tenderization of meat; however, the catalytic action occurred rapidly (within 24 h). However, encapsulation of bromelain with DE system reduced the proteolytic action. Therefore, the results indicated that bromelain-loaded DE had the potential to tenderize meat, which is a necessity for the meat industry and elderly HMR food where meat must be handled for a relatively long period before consumption.